Fig. 1
Intracellular zinc accumulation, as occurs early in excitotoxic conditions, results in the activation of multiple ROS- and RNS-generating mechanisms; which in turn result in further zinc accumulation, leading to cell death. Mitochondrial zinc accumulation leads to the loss of ΔΨm, inhibition of respiration, ROS production, and the development of the mitochondrial permeability transition pore and the promotion of apoptosis through cytochrome c release. NADPH activation by zinc acting through protein kinase C (PKC) represents a particularly robust source of excitotoxic ROS. The direct activation of nNOS and 12-LOX by zinc also represent important sources of oxidative stress and further zinc liberation. DNA damage induced by ROS and RNS generation results in PARP activation and cell death. ROS/RNS elevation induced by zinc can also activate p38 MAPK, which promotes K+ efflux through KV2.1, leading to cell shrinkage and cell death. See text for details
A striking feature of free zinc-induced ROS and RNS generation is that these reactive species interact with cellular targets to oxidatively liberate further zinc. In this way, increased free zinc induces the formation of ROS and RNS that subsequently induce further intracellular zinc release leading to cell death, showing a neurotoxic mechanism of positive feedback between free zinc and ROS/RNS.
Summary
Zinc has many indispensable roles in cellular and neuronal function. Levels of free zinc are tightly regulated by intracellular metal binding proteins and zinc transporters. While assiduously regulated under normal conditions, free zinc levels can rise rapidly under conditions of oxidative stress, wherein ROS and RNS liberate zinc from proteins, and the elevated zinc induces further ROS and RNS generation. Questions on the precise relationship between ROS/RNS, zinc, and calcium elevation remain, where the clarification of the ions’ respective roles in injury represent important future paths of investigation.
The neurotoxic relationship between increased intracellular zinc levels and the generation of ROS has many implications. Important among them is that the reactive species can induce zinc release and increased intracellular free zinc. This elevated zinc can then promote further elevation of ROS and RNS, demonstrating positive-feedback signaling that ultimately leads to cell death under an array of injury scenarios. Therapeutic interventions in these deleterious signaling cascades represent novel approaches at reducing the ROS/RNS and zinc accumulation-induced cell injury under a variety of excitotoxic scenarios.
Declaration of Conflict of Interest
The authors have no conflict of interest to declare.
References
1.
An C, Shi Y, Li P, Hu X, Gan Y, Stetler RA, Leak RK, Gao Y, Sun BL, Zheng P, Chen J (2014) Molecular dialogs between the ischemic brain and the peripheral immune system: dualistic roles in injury and repair. Prog Neurobiol 115:6–24PubMedCentralCrossRefPubMed
2.
3.
4.
Aravindakumar CT, Ceulemans J, De Ley M (1999) Nitric oxide induces Zn2+ release from metallothionein by destroying zinc-sulphur clusters without concomitant formation of S-nitrosothiol. Biochem J 344(Pt 1):253–258PubMedCentralPubMed
5.
6.
Besser L, Chorin E, Sekler I, Silverman WF, Atkin S, Russell JT, Hershfinkel M (2009) Synaptically released zinc triggers metabotropic signaling via a zinc-sensing receptor in the hippocampus. J Neurosci Off J Soc Neurosci 29:2890–2901CrossRef
7.
8.
9.
Caldwell DF, Oberleas D, Clancy JJ, Prasad AS (1970) Behavioral impairment in adult rats following acute zinc deficiency. Proceedings of the Society for Experimental Biology and Medicine. Soc Exp Biol Med 133:1417–1421CrossRef
10.
11.
Clausen A, McClanahan T, Ji SG, Weiss JH (2013) Mechanisms of rapid reactive oxygen species generation in response to cytosolic Ca2+ or Zn2+ loads in cortical neurons. PLoS One 8, e83347PubMedCentralCrossRefPubMed
12.
Cole TB, Wenzel HJ, Kafer KE, Schwartzkroin PA, Palmiter RD (1999) Elimination of zinc from synaptic vesicles in the intact mouse brain by disruption of the ZnT3 gene. Proc Natl Acad Sci U S A 96:1716–1721PubMedCentralCrossRefPubMed
13.
14.
15.
16.
17.
18.
19.
20.
21.
Gee KR, Zhou ZL, Ton-That D, Sensi SL, Weiss JH (2002) Measuring zinc in living cells. A new generation of sensitive and selective fluorescent probes. Cell Calcium 31:245–251CrossRefPubMed
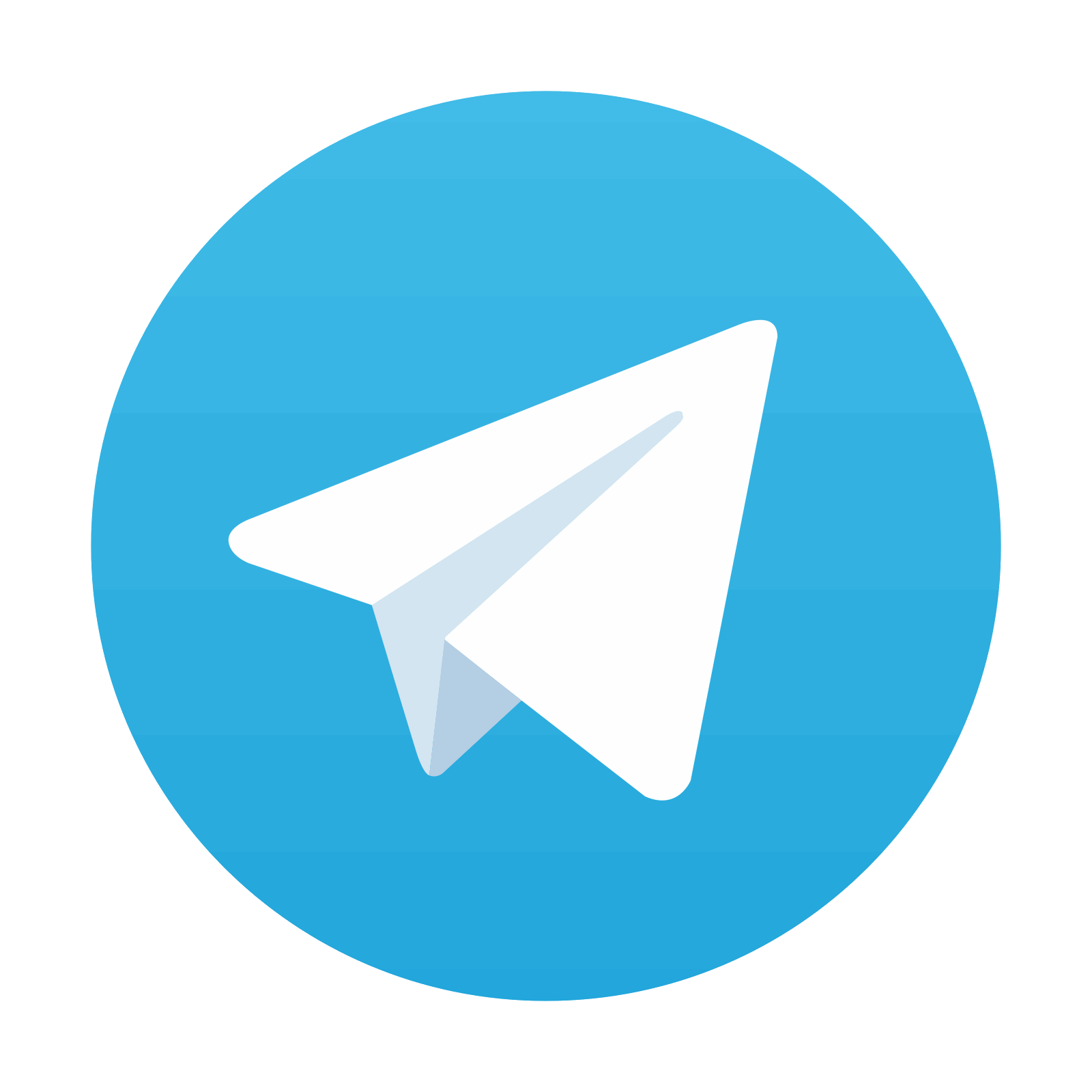
Stay updated, free articles. Join our Telegram channel

Full access? Get Clinical Tree
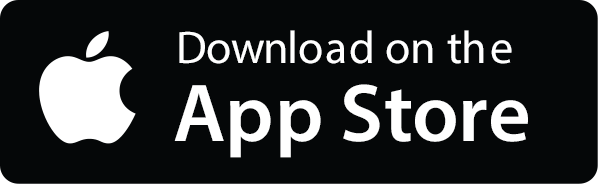
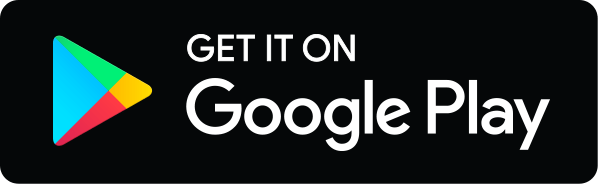