18 Deep Brain Stimulation Approximately one-third of patients with epilepsy will have persistent seizures despite maximal antiepileptic drug (AED) therapy.1–3 Resective brain surgery is typically indicated for patients with refractory partial seizures and can result in at least a 90% reduction in seizure frequency, though permanent neurological deficits or death can occur in nearly 4% of cases.4 At least 50% of patients are not candidates for resection or choose not to undergo such an invasive procedure. Some of these patients opt to have a vagus nerve stimulator (VNS) placed as an adjunct to medical therapy, which is associated with up to a 50% reduction in seizure frequency.5 However, most of these patients will not be seizure free. Thus, due to the success of deep brain stimulation (DBS) for movement disorders,6,7 combined with its advantages of adjustability, reversibility, and less risk of permanent neurological deficits then surgical ablation,8 there has been an explosion of research into implantable devices for treating pharmacoresistant epilepsy.9,10 The mechanism by which DBS may diminish seizures is not completely understood. However, stimulation-induced disruption of unopposed network activity is one hypothesis that appears to be consistent with available data.11,12 Evidence from subthalamic nucleus (STN) DBS suggests that high-frequency stimulation may block epileptiform activity in the cortex,13–15 whereas low-frequency stimulation may drive or synchronize cortical activity. Seizure suppression with electrical stimulation of deep brain structures is effective in animal models using various neural targets, including the cerebellum, hippocampus, caudate nucleus, thalamus, STN, and mammillary nuclei.16–20 We will focus on animal studies of DBS of the anterior nucleus of the thalamic (ANT), which is currently being studied in an ongoing double-blind randomized trial (SANTE), evaluating its efficacy for epilepsy treatment.21 As of March 27 2006, 58 patients at 15 centers had been implanted with deep brain stimulators in ANT. Animal studies investigating efficacy of ANT DBS for epilepsy primarily reflect the work of Mirski and colleagues. Bilateral electrolytic lesions of the tracts connecting the mammillary bodies to the ANT in guinea pigs resulted in essentially complete protection from pentylenetetrazol-induced seizure activity.22 This finding was supported by observations of enhanced glucose metabolism in ANT following administration of both pentylenetetrazole and ethosuximide to guinea pigs.23 Recently, high-frequency DBS (100 Hz) of the ANT has been shown to increase the clonic seizure threshold in a pentylenetetrazole-induced seizure model.24 Interestingly, low-frequency (8 Hz) DBS was proconvulsant as found in earlier studies.15,25 In addition, bilateral ANT DBS may prolong the latency of onset of status epilepticus following administration of pilocarpine, whereas seizures were eliminated by bilateral anterior nuclear thalamotomy.26 Efficacy of ANT DBS for epilepsy in animal models is still unclear given reports of lack of effect, as well as exacerbation of seizure frequency by chronic ANT DBS.26,27 In 1937, James W. Papez described a circuit linking hippocampal output via the fornix and mammillary nuclei in the posterior hypothalamus to ANT.28 ANT projects to the cingulum bundle deep to the cingulate gyrus, which travels around the wall of the lateral ventricle to the parahippocampal cortex, then completes the circuit by returning to the hippocampus.29 Atrophy, magnetic resonance imaging (MRI) signal change, or sclerosis of structures within the classic circuit of Papez has been noted in mesial temporal sclerosis, as well as other forms of epilepsy.30 Stimulation of targets within this circuit is hypothesized to result in direct anterograde cortical stimulation. ANT is a preferred target for DBS because of its relatively small size and projections to limbic structures ultimately affecting wide regions of neocortex. Furthermore, it is not as deep or close to basal vascular structures as the mammillary nuclei. ANT has been lesioned stereotactically resulting in improved seizure control in humans.31 These anatomical connections and precursor ablative techniques have motivated investigation of ANT DBS for epilepsy. ANT DBS is indicated for partial onset epilepsy with or without secondary generalization associated with frequent seizures, resulting in falls, injuries, and impaired quality of life, refractory to 12 to 18 months of at least two therapeutically dosed antiepileptic agents. Patients should be without evidence of progressive neurological or systemic disease and have failed surgical resection and/or vagal nerve stimulation. Scalp video electroencephalogram (EEG) monitoring is necessary to characterize seizure types and demonstrate bilateral or nonlocalizing findings. Preoperative imaging should rule out any lateralizing structural abnormalities. Subjects, family, or the legal guardian must be capable of recognizing the patient’s seizures and maintaining an accurate seizure diary. General endotracheal anesthesia or local anesthesia is administered prior to placement of the Leksell frame. Frame tilt should be parallel to the lateral canthal-external auditory meatal line, which is itself approximately parallel to the anterior commissure–posterior commissure (AC-PC) line. After attachment of the MRI localizer, a 1.5 T MRI is obtained with both fast spin echo inversion recovery and standard T2 images. Computed tomography (CT) can also be used. Target sites were selected from MRI by using 1-mm thick axial, coronal, and sagittal spoiled gradient echo pulse sequences. Indirect localization of ANT is performed with reference to a standard stereotactic atlas38 by identifying the AC-PC line on the sagittal image. AC and PC coordinates relative to the center of the frame are obtained to calculate the midcommissural point (MCP). ANT is 5 mm lateral and 12 mm superior to MCP.38 Because ANT is visible in the floor of the lateral ventricle on MRI, direct localization is possible and frame coordinates are calculated. Simulation of the planned trajectory from entry point to ANT is performed using a surgical navigation system (Medtronic Stealth navigation, Medtronic, Inc., Louisville, CO) to confirm avoidance of sulcal vessels (Fig. 18.1). The MRI is downloaded into the StealthStation computer. Inputting the entry point at or anterior to the coronal suture plots the trajectory, as well as the anterior-posterior and lateral arc coordinates for the Leksell frame. Fig. 18.1 Diagram demonstrating simulation of the planned trajectory to the anterior nucleus of the thalamus for deep brain stimulation using a surgical navigation system (Medtronic Stealth navigation, Medtronic, Inc. Louisville, CO) with a superimposed standard stereotactic atlas. The patient is placed in a supine or semi-sitting position, and after sterile preparation of the unshaven head, and local infiltration with 1% Xylocaine (lidocaine) 1:100,000 epinephrine, an incision is made overlying the coronal suture. Bur holes are placed, and the dura and pia are sharply incised and cauterized. A guide cannula is inserted through the bur hole and advanced deep into the brain to a point 10 mm from the desired target under direct fluoroscopic and Leksell frame guidance. Under local anesthesia, a monopolar single-unit recording electrode (Advanced Research Systems, Atlanta, GA, or FHC, Inc., Bowdoinham, ME) can be introduced to confirm anatomical depth for entry into thalamic tissue after traversing the lateral ventricle. No units are recorded while positioned in the ventricle, but the electrode tip is advanced until recordings are first heard (ANT superficial surface) and then until units cease (intralaminar region) and recommence (dorsomedian nucleus of the thalamus) (Fig. 18.2). Extracellular action potentials are amplified with a GS3000 (Axon Instruments, Sunnyvale, CA) or Leadpoint (Medtronic, Inc., Minneapolis, MN) amplifier and simultaneously recorded using standard recording techniques (300 to 10,000 Hz), together with a descriptive voice channel. ANT neurons are identified based on (1) regional characteristics; (2) a firing rate previously described for human recordings33; and (3) and a characteristic burst firing pattern. After removing the single-unit recording electrode, a stimulation lead (Radionics Stimulation/Lesioning Probe, Burlington, MA) is introduced to elicit the driving response, suggestive of ANT placement (Fig. 18.3). ANT DBS has been associated with recruiting rhythms on cortical EEG in patients with the most pronounced seizure frequency reduction.34 These changes in EEG signal morphology, however, can be elicited from several other thalamic nuclei.25 Once this lead is removed, the DBS lead is advanced to ANT, ensuring that all contacts of the lead are within thalamic parenchyma. The cannula and lead stylet are withdrawn under fluoroscopy, after test stimulation demonstrates no adverse effects. Another fluoroscopic image is done to show that the electrode is secure. The stimulation leads we use are Medtronic 3387 (Medtronic, Inc.) DBS depth electrodes with four platinum-iridium stimulation contacts 1.5 mm wide with 1.5 mm edge-to-edge separation because ANT is relatively larger than other DBS targets. The lead is secured to a bur hole cap, and the skin incision is closed. The Leksell frame is removed, and the head, neck, and infraclavicular regions are sterilized in preparation for placement of the internal pulse generator (IPG) (model Itrel II, Soletra, or Kinetra; Medtronic, Inc.) in a subclavicular pocket bilaterally. The scalp incision is reopened for connection of the lead to an extension wire (Medtronic 7495 Lead Extension, Medtronic, Inc.), which is tunneled subcutaneously to an IPG. Fig. 18.2 Sagittal representation with planned trajectory of electrode implantation for the anterior nucleus of the thalamus. Recordings from single-unit monopolar electrode shown at right from various depth levels, identifying entry into thalamic tissue after traversing the lateral ventricle. (From Kerrigan JF, Litt B, Fisher RS, et al. Electrical stimulation of the anterior nucleus of the thalamus for the treatment of intractable epilepsy. Epilepsia 2004;45(4):346–354. Adapted with permission.) Fig. 18.3 Scalp EEG driving response to left-sided anterior nucleus of the thalamus stimulation. (From Kerrigan JF, Litt B, Fisher RS, et al. Electrical stimulation of the anterior nucleus of the thalamus for the treatment of intractable epilepsy. Epilepsia 2004;45(4):346–354. Adapted with permission.) After reversal and recovery from anesthesia, placement location of the DBS leads is confirmed by MRI or CT (Fig. 18.4). Generally, patients should undergo postoperative monitoring in the epilepsy-monitoring unit, where simultaneous scalp and thalamic EEGs through the implanted leads can be recorded. Patients are discharged to home 2 days post-operatively, but multiple outpatient visits are usually necessary to optimize stimulation parameters. Stimulators are turned on approximately 10 to 14 days postoperatively. For initial stimulation, frequency is set at 90 to 130 Hz, pulse width at 60 to 90 microseconds, and pulse amplitude at 4 to 5 V; and the contact inducing the optimal clinical effect at minimal voltage and with the fewest side effects is identified. The benefits of bipolar versus monopolar stimulation remain to be determined as do “cycling” versus “continuous” stimulation. A recent study of bilateral ANT DBS in four patients with refractory epilepsy showed no significant difference in seizure frequency between cycling and continuous stimulation period using within-group comparisons.32 One proposed method of cycling or intermittent stimulation using an “open-loop” device (discussed below) is through a “duty cycle” of 1 minute on and 5 minutes off.9 Intermittent stimulation may increase the battery life of the IPG; is theoretically safer for neural tissue than chronic, continuous stimulation; and has been successful in clinical trials for vagus nerve stimulation for epilepsy.34 Unfortunately, we are limited to small-sample human pilot studies in evaluating ANT DBS as a treatment for pharmacoresistant epilepsies, though a multicenter randomized clinical trial of intermittent bilateral ANT DBS for medically intractable seizures is currently underway.21 Despite significant individual variation in outcome, overall bilateral high-frequency ANT DBS appears to be safe, well-tolerated, and effective in some subjects with inoperable, refractory epilepsy. Table 18.1 summarizes the results of seven pilot studies of ANT DBS. Fig. 18.4 A T1-weighted sagittal (A) and T2-weighted axial (B) magnetic resonance image demonstrating lead placement for deep brain stimulation of the anterior thalamic nucleus. Upton et al (1987)39 reported on 4 of 6 patients with intractable epilepsy who experienced a significant reduction in seizure frequency, one of whom was completely seizure free at follow-up.39 This may be due to microthalamotomy or lesioning of the ANT, an effect that has been noted previously.40 In fact, electrode implantation appears to decrease seizure frequency itself, and activation of the IPG and multiple subsequent adjustments to stimulatory parameters or contacts stimulated may not be linked to any further benefit in seizure control.32,37 However, in one patient, the IPGs were turned off after 3 years of DBS with maintenance of improved seizure control for more than 1 year. Thus, it is difficult to distinguish the relative effects of electrode insertion and stimulation to the reduction in seizure frequency. The effect of microthalamotomy on seizure propagation, however, remains to be debated as a recent study was unable to detect a lesion effect during the immediate postoperative period.35 Other pilot studies of intermittent ANT DBS have reported significant reduction in frequency and propagation of generalized tonic-clonic seizures (GTCSs) and complex partial seizures in 4 of 5 patients.33 However, only 1 of the 5 patients showed a statistically significant reduction in total seizure frequency. Importantly, discontinuation of DBS resulted in an immediate increase in seizure frequency and intensity that improved when stimulation was resumed. This suggests that ANT DBS–induced inhibition is at least in part implicated in reducing seizure frequency.32,33,35 The beneficial response to closed-loop stimulation (described below) provides further support for a beneficial role of electrical stimulation. A recent study reporting long-term follow-up noted that electrode implantation and stimulation in ANT was followed by more than a 50% seizure reduction in 5 of 6 patients. However, significant benefit in two patients was not seen until years 5 and 6, respectively, with the addition of further adjuvant AEDs.37 There is evidence for a long-term effect of ANT DBS on the propensity to develop seizures,37 and indeed, ongoing clinical benefit after cessation of DBS has been noted in tremor.41 Bitemporal mesial epilepsy, as opposed to extratemporal or poorly localized seizures,33,34,37 may be most responsive to ANT DBS given a recent report of more than a 75% reduction in seizure frequency.35 Seizure frequency decreased by 93% in one patient, which is the most dramatic response reported in the literature. Alternatively, a higher mean frequency (157 Hz) may at least in part explain this impressive response. ANT DBS appears to be a safe, well-tolerated treatment of refractory seizures with all of the reported complications and stimulation-induced adverse effects being mild and transient. Reported adverse effects due to stimulation are paranoid ideation, intermittent nystagmus with cycling stimulation, auditory hallucinations, anorexia, and lethargy.35,37 Complications due to the surgical procedure or hardware include a small, right frontal hemorrhage without any permanent neurological deficit, scalp erosion necessitating removal of the implanted system, infection of the right anterior chest, and accidental turning-off of the stimulator.32,34,42 Although the SANTE trial will only be evaluating ATN DBS, other neural targets are worth discussing to provide a more thorough review of the potential for DBS in epilepsy. Some open-label clinical trials have reported on DBS of various targets to treat pharmacoresistant seizures, demonstrating marginal efficacy in stimulating the centromedian nucleus of the thalamus (CMT), caudate nucleus, cerebellum, and STN.
Deep Brain Stimulation for Epilepsy: Evidence from Animal Studies
Deep Brain Stimulation of the Anterior Nucleus of the Thalamus
Rationale
Indications32–35
Targeting and Surgical Procedure32–37
Pilot Studies
Complications
Other Anatomical Targets for Deep Brain Stimulation for Epilepsy
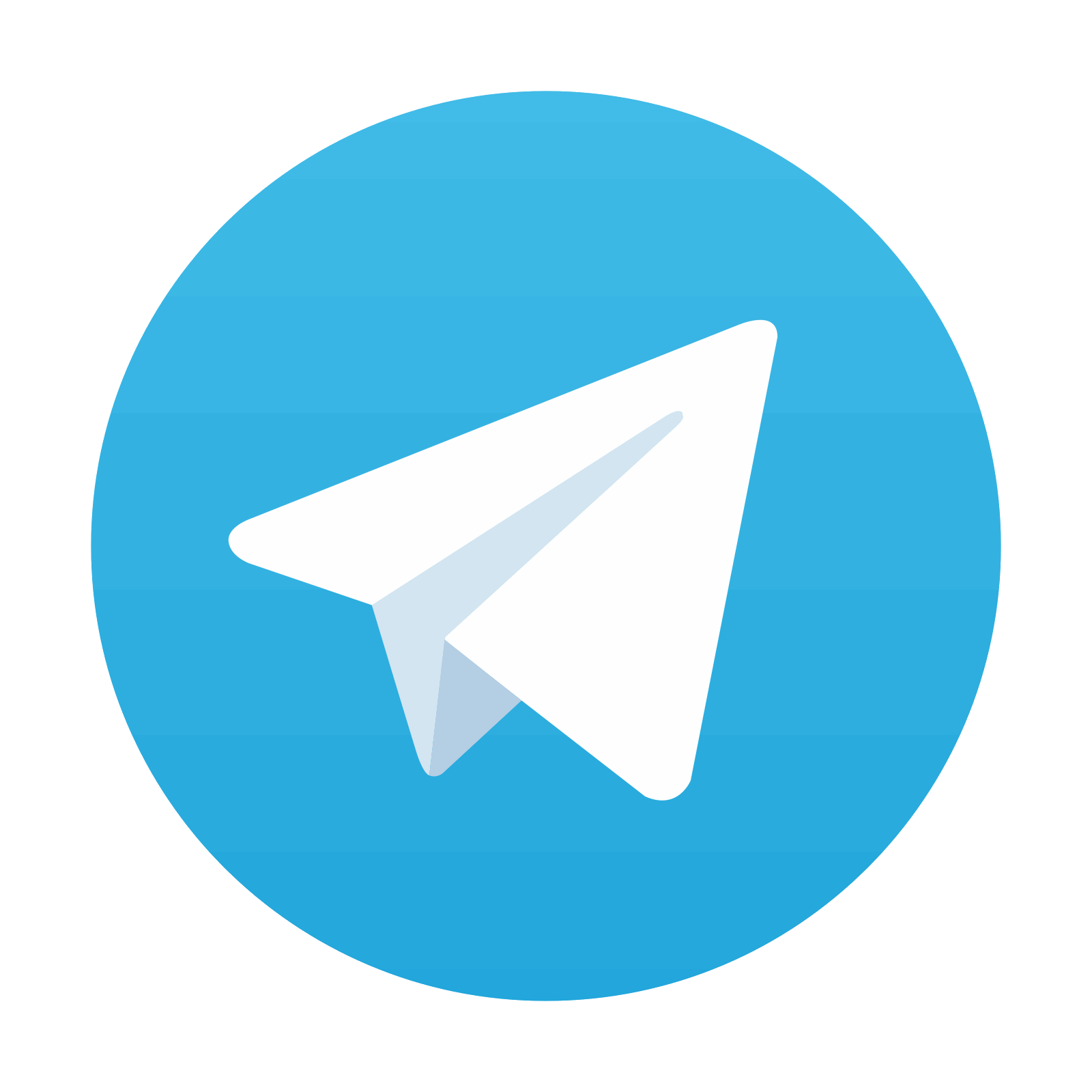
Stay updated, free articles. Join our Telegram channel

Full access? Get Clinical Tree
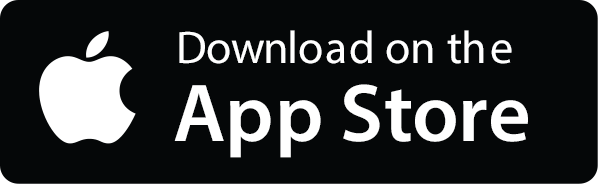
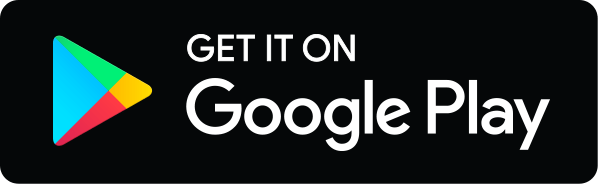