12 Deep Brain Stimulation for Dystonia Brian Harris Kopell, Craig I. Horenstein and Ali R. Rezai What is the basic feature of the disease I have detailed? We find no indication of paralysis and the patient can move about freely. However, there is a certain clumsiness, especially of the upper extremities, and the movements are neither graceful nor elastic but rather stiff and disjointed … there is no athetosis … if the patient is fully at rest, the extremities are also quiet…. If involuntary movements are present, they show rhythmic, clonic features…. Upon detailed inspection, it becomes obvious that isolated muscles have a tendency to a moderate tonic tension … we are surprised to encounter rather a hypotonia upon passive movement of the lower extremities…. The salient feature of this disease is an alteration of muscle tone.1 In 1911, Hermann Oppenheim, a German neurologist, introduced the term dystonia to designate the coexistence of muscular hypotonia and hypertonia.1 Dystonia is a heterogeneous group of disorders that encompasses a wide range of manifestations and etiologies. The hallmark of dystonia is involuntary twisting movements and postures, which may be exacerbated by voluntary movements. Most dystonias are treatable with medication and botulinum toxin. However, refractory dystonia may also cause severe functional handicap, debilitating pain, and progress to life-threatening stages. It is for these cases of intractable dystonia that deep brain stimulation (DBS) should be considered. Traditionally, dystonia was classified according to the distribution of symptoms, etiology, and age of onset. In focal dystonia a single body region is affected, which includes writer’s cramp (arm), blepharospasm (eyes), cervical dystonia/torticollis (neck), and spasmodic dysphonia or laryngeal dystonia (larynx). In segmental dystonia, two or more adjacent areas are affected. Examples include cranial–cervical dystonia, crural dystonia (one leg plus trunk or both legs), and brachial dystonia (one arm with trunk or both arms). Multifocal dystonia refers to cases where two or more noncontiguous body regions are affected. Generalized dystonia refers to crural dystonia with at least one other body part involved. When dystonia is confined to one side of the body, it is termed hemidystonia. Historically, age of onset was perhaps the most important classification feature because it was the most significant prognostic indicator of whether focal symptoms would spread to other body parts. Early-onset dystonias typically present around age 9. They begin focally, usually in an arm or leg, and often spread to the trunk, resulting in a generalized dystonia producing severe disability. Adultonset dystonia commonly presents in the fourth and fifth decades and manifests with focal symptoms of the cranial or cervical areas.2 Symptoms usually remain localized though they can still cause significant disability. Generalization nevertheless does occur in 15 to 30% of patients with adultonset primary facial, cervical, or upper extremity dystonia.3 Dystonic tremor is sometimes difficult to distinguish from other forms of tremor, especially essential tremor (ET). It is characterized as irregular and slow (4 to 7 Hz) tremorlike movements that are frequently associated with focal dystonia such as torticollis or writer’s cramp. As our understanding of the molecular genetics and pathophysiology of dystonia has increased, more clinically useful classification schemes have been developed. Fahn et al4 proposed classifying dystonias into one of four categories: primary dystonias, dystonia-plus syndromes, heredo-degenerative dystonias, and secondary dystonias. Primary dystonia refers to syndromes where there is no history of a brain injury (e.g., trauma or anoxia), brain imaging and laboratory studies do not suggest an etiology, and the symptoms are unresponsive to low dose L-dopa. The most common form of inherited primary generalized dystonia is DYT-1 dystonia. Dystonia musculorum deformans (Oppenheim’s dystonia) was the original name of what is now known as DYT-1 dystonia. With an autosomal dominant inheritance pattern and variable penetrance, it accounts for 90% and 60% of early-onset primary dystonia in Jewish and non-Jewish children, respectively. The gene in question encodes the protein torsion A and has been mapped to a GAG deletion on chromosome 9q34.1. Dystonia-plus category encompasses dystonic syndromes associated with clinical and laboratory findings suggestive of a neurochemical disorder without evidence of neurode-generation, such as the L-dopa responsive dystonias. Heredodegenerative dystonias include neurodegenerative disorders that often produce dystonia as a prominent clinical feature, but other neurological features are usually present. The class of heredodegenerative dystonias includes Parkinson disease (PD), Wilson disease, Huntington disease, and GM1 and GM2 gangliosidosis. The fourth category, secondary dystonia, refers to syndromes where dystonia occurs as a result of an environmental insult to the brain. Focal dystonia can occur after direct injury to an extremity and is not unique to brain insult. Common causes include perinatal cerebral injury/ anoxia, encephalitis, trauma, hypoxia, and L-dopa–induced dystonia. In the 1930s, Russell Meyers first performed selective basal ganglia lesions via craniotomy for the treatment of extrapyramidal movement disorders, including dystonia. Although he found that pallidal lesions abolished abnormal movements without causing weakness, his high rate of side effects resulted in pallidal targets being abandoned by the surgical community.5,6 In the 1950s, surgeons began revisiting Meyers’s pallidotomy using stereotactic techniques.7–10 Early stereotactic pallidotomies targeted the anterodorsal portion of the globus pallidus, and though the surgeries could be performed with less morbidity and mortality, the clinical outcomes were poor.11 For the next several decades, thalamic targets, including the ventral lateral thalamus [ventral intermediate (Vim), ventro-oralis anterior (Voa), ventro-oralis posterior (Vop), ventral caudal (Vc)], centromedian nucleus (CM), pulvinar, and subthalamic region were the preferred sites for surgical intervention in dystonia.12–15 Cooper performed thalamotomies on 226 patients with primary or secondary generalized dystonia between 1953 and 1976.12 He initially targeted the ventral lateral thalamus (Voa/Vop) and CM, but he refrained from simultaneous bilateral interventions due to the high risk of speech disturbances. If a further operation was required, Cooper lesioned the pallidal and cerebellar efferent fibers to the thalamus. If a patient was still disabled following this surgery, he performed a pulvinotomy. Cooper reports that improvement of dystonia was progressive and occurred up to 6 months postoperatively. Furthermore, a larger lesion was needed to treat dystonia than was necessary to suppress parkinsonian tremor. After an average follow-up of 8 years, 25% of Cooper’s patients had “good” and 45% had “moderate” improvement. There was no change in 18% of patients, and symptoms worsened in 12%. Cooper’s best results were in Jewish patients with early-onset hereditary dystonia. In 1952, Leksell noticed improved outcomes following pallidotomy for movement disorders when he modified the anterodorsal pallidotomy to a more posteroventral target, where the ansa lenticularis emerges.16 This work was largely overlooked until Laitinen’s efforts in the late 1980s.11 Leksell’s posteroventral pallidotomy (PVP) was particularly successful in treating tremor, rigidity, and bradykinesia, as well as on-period dyskinesias and off-period dystonias. The beneficial effects on dystonic symptoms in PD encouraged neurosurgeons to attempt the PVP for the treatment of dystonia, and in recent years, the PVP has been performed for the treatment of dystonia with good results.17,18,19,20,21 Although still controversial, bilateral PVP has been associated with increased risks of dysphagia, speech difficulty, and cognitive disturbance compared with unilateral interventions.22 This increased risk makes DBS for dystonia more attractive than PVP due to the often needed bilateral aspect of intervention. Peripheral denervation procedures for focal cervical dystonia have been largely replaced by botulinum toxin injections but may still be considered in medically refractory patients. Various selective peripheral denervation procedures have been used for treating cervical dystonia, including rhizotomy with intradural sectioning of anterior cervical roots C1–C3, posterior ramisectomy with extradural sectioning of the dorsal rami, microvascular decompression of the spinal accessory nerve, and myotomy. Krauss et al23 used a combination of denervation procedures tailored to each patient’s specific symptoms and reported mild to excellent improvement in 41 of 46 patients. In 1960, Hassler et al24 reported that low-frequency (4 to 8 Hz) stimulation of the pallidum in a patient with primary cervical dystonia and athetosis elicited abnormal movements, whereas higher-frequency stimulation eventually suppressed them. However, no other reports of electrical stimulation for the treatment of dystonia appear in the literature until 1977, when Mundinger25 reported encouraging short-term results for seven patients with cervical dystonia who underwent unilateral, low-frequency (2 to 12 Hz), intermittent (30-minute, several times per day) electrical stimulation of the thalamus (Voa, Voi, and sub-thalamic areas). However, no long-term results were ever published, and the technique was abandoned due to difficulty with the hardware. In the 1980s, several groups reexamined thalamic stimulation for dystonia. Andy, stimulating in the motor thalamus, demonstrated that intermittent 50 Hz thalamic stimulation improved symptoms in two patients with cervical dystonia.26 Targeting the sensory (Vc) thalamus, Siegfried27 reported a reduction of dystonic symptoms in four patients treated with 33 Hz stimulation, and Sel-lal et al28 showed a dramatic improvement in a patient with secondary hemidystonia with intermittent 60 Hz stimulation. The modern era of continuous high-frequency deep brain stimulation (DBS) began when Benabid et al29 stimulated ventral Vim in PD or ET patients. The procedure was later performed in the globus pallidus internus (GPi) and in the subthalamic nucleus (STN).30,31 The ensuing success of high-frequency DBS in treating ET, PD, and related L-dopa– induced on-dyskinesias and off-dystonias encouraged surgeons to expand the indications for DBS. Pollak et al32 first applied high-frequency DBS for generalized dystonia when they stimulated Vim in 12 patients with generalized dystonia. The following year, three groups reported short-term results of pallidal DBS in the treatment of dystonia.33–35 The positive outcomes encouraged other groups to attempt DBS in dystonic patients of varying etiologies, and GPi soon became the preferred target for DBS for dystonia. The indications for DBS for the treatment of dystonia are starting to emerge, though large, prospective, randomized trials are lacking.36,37 Patients who are considered for surgery must have severe motor symptoms and/or pain refractory to medical therapy and resulting in significant functional disability. A variety of medications are utilized before a patient is considered refractory. Patients with primary dystonia initially undergo a therapeutic trial with L-dopa. If this is unsuccessful, anticholinergic therapy with trihexyphenidyl is instituted, and benefit is typically seen in 40 to 50% of patients.38–40 Baclofen and benzodiazepines are generally considered useful as adjunctive therapies. Bac-lofen has been reported to be more effective in children and adolescents, and intrathecal baclofen is used in patients with dystonia associated with spasticity and pain.41,42 Clozapine, an atypical neuroleptic that primarily blocks D4 receptors, has shown a 30% improvement in subjective ratings and dystonia scales.43 Other commonly used medications for treating dystonia include mexiletine (an anti-arrhythmic related to lidocaine), anticonvulsants, muscle relaxants, and riluzole (a glutamine antagonist).41 Botulinum toxin injections have become one of the main medical therapies, with response rates of 70 to 100%, depending on the type of dystonia.44 Single injections range from $100 for focal dystonia to $2500 for generalized dystonia.45 There are many different subtypes of botulinum toxins, but only type A (BOTOX, Allergan, Inc., Irvine, CA) and type B (Myobloc/Neurobloc, Solstice Neurosciences, Inc., South San Francisco, CA) have been approved by the U.S. Food and Drug Administration (FDA) for use in dystonia.41 Approximately 4 to 10% of patients, however, do not respond to botulinum toxin injection, either primarily or secondarily, due to development of neutralizing antibodies.46 Clinical evaluation of dystonia is essential for patient selection prior to surgery and for documenting outcomes postoperatively. Currently accepted rating scales include the Global Dystonia Rating Scale (GDRS), the Burke-Fahn-Marsden (BFMDRS), and the United Dystonia Rating Scale (UDRS) for generalized dystonia, and the Toronto Western Spasmodic Torticollis Rating Scale (TWSTRS) for cervical dystonia.37,47,48 A large, multicenter study conducted by the Dystonia Study Group critically assessed the BFMDRS, GDRS, and UDRS, confirming their internal consistency, validity, interrater reliability, and ease of use, concluding that the BFM-DRS and GDRS were appropriate for use in clinical trials and the UDRS was appropriate for use in an office setting.47 Traditionally, only patients with generalized, segmental, or hemidystonia were considered for stereotactic neu-rosurgical procedures. Focal dystonia usually responds to botulinum toxin injections and, in most cases, does not produce significant enough disability to warrant the risks of surgery. Furthermore, published outcomes have shown that patients with primary generalized dystonia have the best surgical outcomes, and patients with appendicular symptoms are believed to respond better than patients with axial disease. The literature also suggests that the subset of patients with DYT-1 dystonia has the best results following stereotactic lesioning or DBS.49–51 However, the reported safety of bilateral GPi stimulation has encouraged various groups to attempt to expand the indications for DBS in dystonia. The results from the literature suggest that many patients with severe focal dystonia and secondary dystonia may also benefit from chronic DBS therapy. Stereotactic neurosurgery has traditionally been considered the last option in treating severe dystonia. However, recent reviews have shown bilateral DBS to be safe and highly effective in treating adults and children with various types of dystonia and PD. Because of its safety and reversibility, DBS is replacing brain lesioning as the preferred stereotactic procedure.52,53 Currently, thalamic and pallidal targets have been the focus for DBS intervention for dystonia, with pallidal stimulation dominating the investigative literature both by frequency performed and by efficacy. The pallidal target is the sensorimotor portion of the GPi as initially described by Leksell and popularized by Laitinen.11,16 Though the exact lead placement varies from institution to institution, it is generally agreed to be 18 to 21 mm lateral to midline, 2 to 3 mm anterior to the midcommissural point (MCP) and 3 to 6 mm ventral to the intercommis-sural plane. The DBS tip is ideally 4 to 5 mm anterior to the internal capsule border and 2 mm dorsal to the optic tract. Many authors favor placing the leads laterally within this territory to avoid complications secondary to DBS effects on the internal capsule. An additional variable has been the higher voltages used to achieve therapeutic benefits. This has necessitated a more anterior placement of the DBS lead to reduce the current spread to the internal capsule. Thalamic interventions have been mainly described in the Voa/Vop and Vim thalamus. Such coordinates have been as follows: Voa—2 mm anterior to the MCP, 10 mm lateral to the MCP, and 5 mm dorsal to the intercommis-sural plane54; Vim—6.0 to 6.5 mm anterior to the posterior commissure, 13.8 to 14.0 mm lateral to the MCP, and 1 mm dorsal to the intercommissural plane.55 Because most published reports for DBS for dystonia have focused on the internal pallidum, the following sections discuss physiology and localization methods from the perspective of the pallidal target. Key aspects of thalamic intervention are also given. Although DBS for dystonia shares many common features with DBS for other movement disorders, there are several unique considerations. The first issue concerns target acquisition. Preoperative target localization is performed utilizing magnetic resonance imaging (MRI). MRI sequences such as inversion recovery can directly visualize the borders of the GPi and allow modification of the initial target based on the anatomical variations of individual patients.56 These images are often merged with a concurrent computed to-mographic (CT) scan because CT presently produces less spatial distortion of localizing fiducials than does MRI. Vayssiere et al57 reported that accurate DBS lead placement with good clinical outcome can be achieved based solely on MRI localization. Postoperative MRIs, obtained immediately after surgery with the patient still under general anesthesia, should confirm precise lead placement. The actual lead placement was not statistically (mean 2 to 3 mm) different from the theoretical target chosen preoperatively. These authors also report a mean improvement of 83.8% in the BMFDRS score at a mean follow-up of 1 year. Another method utilizes “semimicroelectrode” guidance and tissue impedance monitoring to guide final targeting of the DBS electrode.58 Most institutions, however, utilize intraoperative micro-electrode recording (MER) to confirm accurate lead placement. MER is still highly recommended for several reasons.56 First, the borders of target nuclei are not always perfectly visualized on MRI. Despite the use of CT fusion, there may still be image distortion effects, and shifting of brain tissue may occur intraoperatively due to cerebrospinal fluid (CSF) leakage and air entering the cranial cavity. Second, there is not always a perfect correlation between structure and function, especially in patients with pathophysiological changes.56,59 Reports of DBS placement have shown that the first trajectory chosen does not usually result in optimal lead placement, and that based on the results of MERs, the optimal lead placement varies by 1.27 mm or greater from the initial target in 25% of cases.60 Finally, microstimulation enables the surgeon to confirm that the lead placement will allow stimulator settings to be increased to higher voltages without causing pyramidal side effects via activation of fibers in the internal capsule, intolerable paresthesias via activation of the Vc nucleus/medial lemniscus, or visual scotomas from optic tract activation. Anesthesia management is particularly important during DBS interventions for dystonia. Often patients with dystonia, especially severe generalized and cervical dystonias and the pediatric population, will present with challenges regarding airway management, headframe placement, and comfort during the procedure itself. Unlike PD patients, who in their off state are often rigid and immobile, the dystonic patient can have violent, uncontrolled movements. Initially this can make accurate headframe placement extremely difficult. In these patients, careful coordination with anesthesia colleagues to obtain propofol intravenous (IV) sedation or light general anesthesia with laryngeal mask airway (LMA) can be helpful during this phase. During the surgical implantation of DBS electrodes, IV sedation, especially propofol, can confound MER data by lowering the firing rate of the globus pallidus externus and internus (GPe, GPi).61 Although many adults can tolerate awake-surgery in this state, the pediatric population may not. Starr et al62 reported the use of ketamine and opiate sedation in pediatric dystonia patients that seemed to interfere least with MER data. Such combinations and others such as the 2 agonist, dexmedetomidine may prove useful in providing sedation and pain control for dystonia patients while allowing electrophysiological mapping to occur. Some of these challenges may be overcome with the use of frameless stereotactic equipment. A frameless stereotactic system may potentially obviate the need for the dystonia patients, with their attendant abnormal and sometimes violent movements, to be constrained by a headframe. This freedom of movement would necessarily afford the patient greater comfort and the anesthesiology personnel a greater degree of control over airway function. The issue of accuracy of these systems compared with the standard frame-based placement is still being evaluated by several centers.
Classification of Dystonia
History of Treatment for Dystonia
Pallidotomy and Thalamotomy
Peripheral Denervation Procedures
Electrostimulation
Deep Brain Stimulation for Dystonia
Indications and Patient Selection
Targets
Target Acquisition, Headframe Placement, and Anesthesia Management
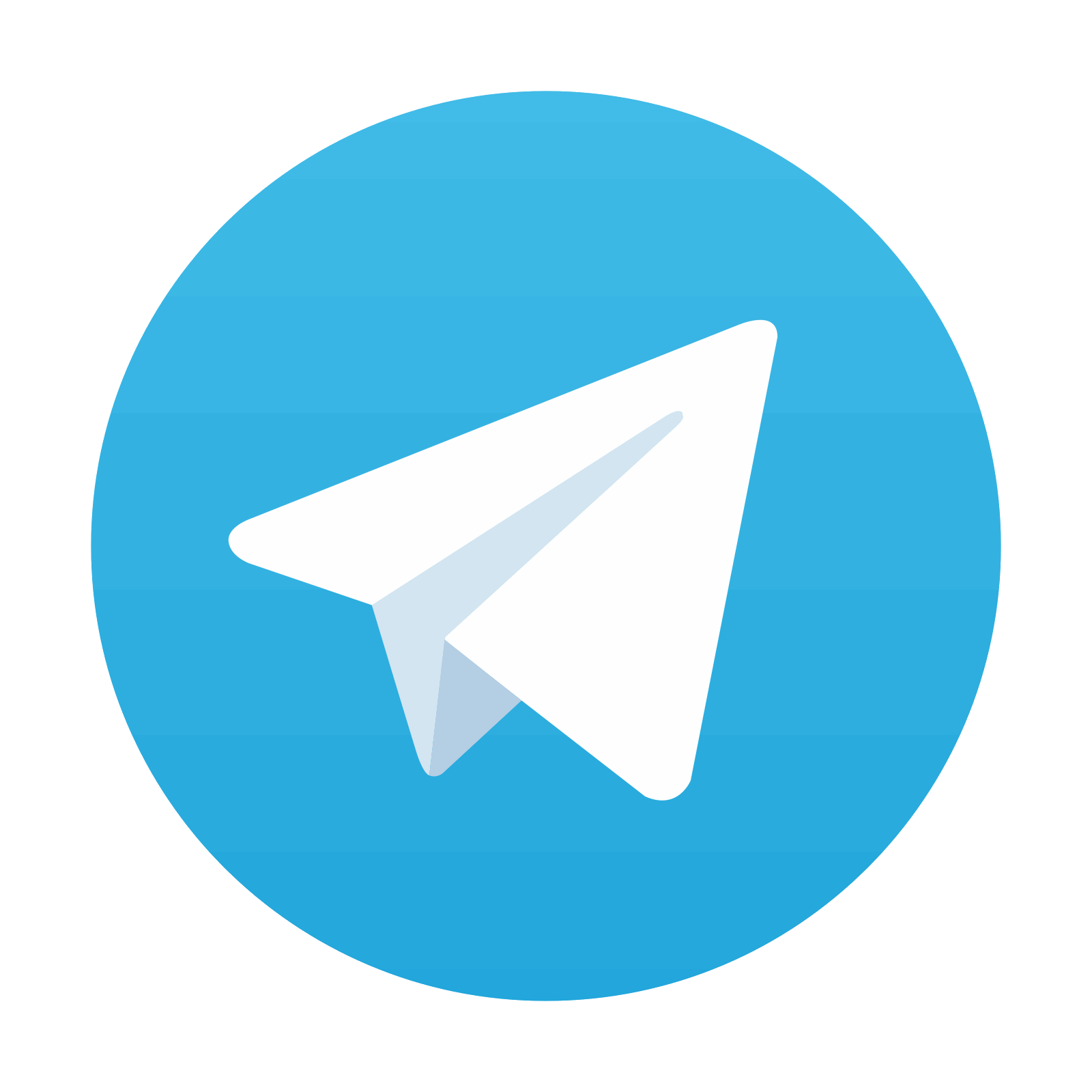
Stay updated, free articles. Join our Telegram channel

Full access? Get Clinical Tree
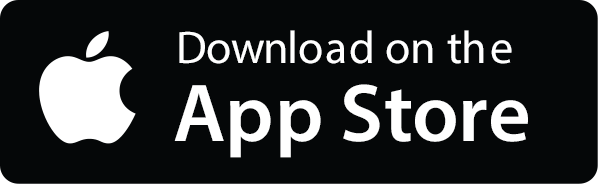
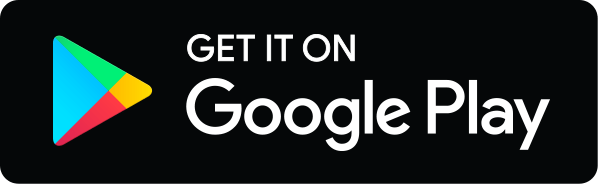