Deep-brain stimulation selection decision-making algorithm, based on CAPSIT criteria.
Given that DBS benefits those symptoms that are predominately levodopa responsive, improvement from levodopa is a fundamental criteria for selection, and a 33% decrease in Unified Parkinson’s Disease Rating Scale (UPDRS)-III motor score between the off-medication and on-medication states is widely considered the threshold for predicting a good outcome from DBS. This is tested by performing an evaluation of the motor response to levodopa whereby a patient undergoes an assessment in the practically defined “off” period, i.e. following a 12 h washout from any dopaminergic medication, and again in the “on” state, after a therapeutic or sometimes supratherapeutic dose of levodopa [16].
A secondary purpose of such a levodopa challenge is to identify patients with a suboptimal response to levodopa, which may represent patients with atypical parkinsonian disorders, previously considered as PD, for whom DBS may lead to unsustained benefits at best or even accelerated decline at worst [19]. A thorough preoperative neurocognitive and behavioral assessment is performed in order to exclude patients with overt or emerging dementia, or with underlying psychiatric conditions that could be exacerbated by, or lessen the response to, surgery. Depression should be optimally treated prior to considering surgery. The presence or absence of depression could influence the choice of target, as some data suggest that STN stimulation may worsen depression, whereas GPi stimulation may alleviate it [20]. Psychosis is not an absolute contraindication to DBS but is frequently a harbinger of an emerging dementia; therefore extreme caution is needed. Mild cognitive impairment in one area, such as executive functioning, is common in PD and is not necessarily an exclusionary factor. However, involvement of multiple domains, particularly localizable to posterior cortical functioning, suggests an atypical parkinsonism or comorbid Alzheimer’s dementia. In either case, DBS can be associated with deterioration of cognitive function and the development of frank dementia. Finally, it is important to manage patient expectations by educating prospective surgical candidates on what DBS can and cannot accomplish, and on the potential risks. This includes ensuring that the goal for DBS (e.g. dyskinesias or reduction in “off” time) corresponds to what the patient finds troublesome and is worthy of assuming the surgical risks. Postoperative questionnaires have demonstrated a high degree of satisfaction, with 89% of patients believing that they made the right decision to undergo DBS, 78% making the same decision again and 72% recommending DBS to another patient in a similar situation [21].
In terms of the timing of STN-DBS, by the time medically intractable fluctuations and dyskinesias occur, patients often find themselves with impaired quality of life, and with social and professional disability [22]. For this reason, DBS is increasingly being considered earlier in the disease process, in an attempt to prevent disability from accruing. The EARLYSTIM trial demonstrated superiority of STN-DBS over medical therapy in patients with early motor complications [23], with quality-of-life measurements improving in parallel with motor improvements. However, several pitfalls in the design of this study, including a 7-year disease duration (which is not easily defined as “early” by most definitions) and the “lessebo” effect shown by those allocated to the best medical therapy arm (who had presumably become study subjects with the expectation of receiving surgery rather than oral medications) cast doubt on the generalizability of their conclusions [24]. Indeed, a recent study applying more stringent criteria for “early” DBS demonstrated no differences in motor function between the surgical and best medical therapy arms [25]. While there remains cautious reluctance in offering an invasive therapy to patients still adequately managed by oral therapies, the prevention of accumulating disability and the resultant improvement in quality of life are attractive possibilities that warrant further study. Currently, however, disease progression following STN-DBS appears to continue at the same rate as in those who are medically treated [26, 27]. The long-term effects of STN-DBS stimulation remain beneficial, and the improvements found from stimulation can persist for over a decade [10, 13], although nonmotor and axial motor features, which are less responsive to STN-DBS, dominate the clinical picture in advanced stages, detracting from its overall benefit [28, 29].
The procedure
The neurosurgical procedure of selection and implantation of the DBS electrodes has been well described elsewhere [30, 31]. Electrodes are typically implanted under local anesthesia, although some centers may use general anesthesia. Prior to the procedure, the neurosurgeon plans the surgery with targeting based on MRI localization of visual landmarks and brain atlases using brain-mapping software. Targeting is also compared with expected three-dimensional coordinates, established by a frame placed around the patient’s skull or alternatively with other markers if a frameless system is used. Once the coordinates and trajectory have been mapped, a burr hole is made for each respective electrode.
The microelectrode is channeled down the mapped trajectory and the STN target is confirmed via microelectrode recording, typically performed by an electrophysiologist or trained movement disorder specialist (Figure 19.2). After the target is confirmed, the microelectrode is removed and replaced with a stimulating macroelectrode. The stimulating macroelectrode is tested during surgery to determine optimal benefits and identify side effects and the threshold for their effect (Table 19.1). The final position of the macroelectrode is that at which the greatest efficacy on rigidity and motor symptoms is obtained with the lowest stimulation intensity and highest threshold for side effect. Quadripolar electrode models are frequently used, and bilateral placement in a single operative session is typically performed. Although unilateral stimulation may benefit a subset of patients [33], its benefit is typically limited to contralateral symptoms and may not provide maximal improvement in walking [34].
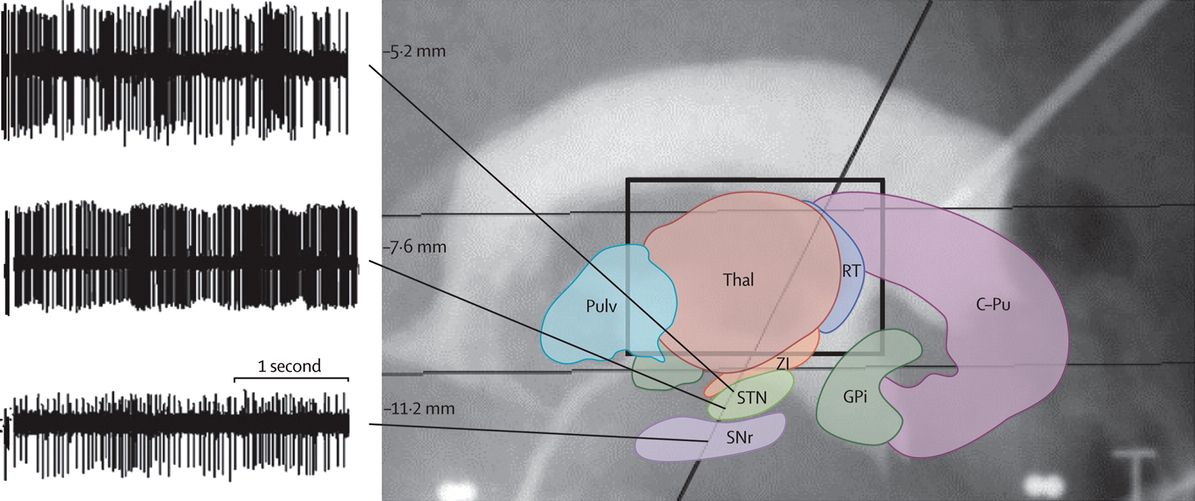
Stereotactic localization of the subthalamic nucleus using firing patterns from microelectrode recordings. The microelectrode trajectory (black oblique line) encounters the thalamus and then the zona incerta, followed by a rich, irregular burst of neural activity in the kinesthetic neurons of the subthalamic nucleus (STN; 5.2 and 7.6 mm below the commissural line in this patient), and high-amplitude, regular and unresponsive neural activity in the substantia nigra pars reticulata (SNr; 11.2 mm below the commissural line). C-Pu, caudate and putamen (striatum); GPi, internal globus pallidus; Pulv, pulvinar; RT, reticularis thalami; Thal, thalamus; ZI, zona incerta.
Possible intraoperative subthalamic nucleus stimulation-induced side effects
Stimulation-induced side effect | Localization of electrical stimulation |
---|---|
Paresthesias | Medial lemniscus (posterior) |
Photopsia | Medial lemniscus (posterior) |
Eyelid opening apraxia | Oculomotor basal ganglia loop |
Gaze deviation | Oculomotor basal ganglia loop |
Diplopia | Fascicles of CNIII or supranuclear oculomotor system |
Ataxia or vertigo | Red nucleus (medial) |
Face or arm muscle contraction | Internal capsule |
Dysphagia | Corticobulbar fibers |
Dysarthria | Corticobulbar fibers |
Sweating or flushing | Hypothalamus (anterior) |
Dyskinesias | Indicative of proper subthalamic nucleus placement |
After successful determination of the position, the macroelectrode is secured, the surgical site is closed and typically within a week or two the implantable pulse generator is surgically placed. Following surgery, patients may experience a “lesion effect” whereby symptoms are transiently improved due to the placement of the electrode alone. This effect generally disappears after several days to weeks.
Outcomes/proof of efficacy
Randomized controlled trials comparing STN-DBS with best medical therapy (levodopa) have consistently demonstrated that neurostimulation produces a greater improvement from baseline in the UPDRS and 39-Item Parkinson’s disease Quality of Life Questionnaire (PDQ-39) [10, 35]. A trial by Deuschl et al. [10] demonstrated PDQ-39 improvements of 24–38% compared with medication management alone when examining the subscales for mobility, activities of daily living (ADLs), emotional well-being, stigma and bodily discomfort (Table 19.2).
Clinical trials comparing deep-brain stimulation with best medical therapy The data below depict changes from baseline of each surgical group versus medical therapy
Study | Surgical site | Mean age (years) | Duration of disease | Mean decrease in “off” time | Mean increase in “on” time | UPDRS-III change (STN vs medical) | Dyskinesia scale or change | PDQ-39 summary index mean changea | Duration of follow-up |
---|---|---|---|---|---|---|---|---|---|
Deuschl et al., 2006 [10] | STN | 60.5 (±7.4 SD; maximum age 75) | Unknown.; mean stage 4 | 4.2 vs 0 h | 4.4 vs –0.5 h | 41 vs 1% improvement | Scale: 6.7 vs 0, a 54 vs 0% improvement | –9.5 (–13.1 to –5.9) vs +0.2 (2.4–2.9) | 6 months |
Weaver et al., 2009 [36] | STN or GPi | 62.4 (range 37–83) | 12.4 years (± 5.8 SD) | 2.4 h vs 0 h | 4.6 vs 0 h | 71 vs 32% improvement | Unknown | –7.7 (–9.7 to –5.6) vs 0.4 (–1.0 to 1.8) | 6 months |
Williams et al., 2010 [37] | STN or Gpi | 59 (range 37–79) | 11.5 years (2.0–32.2) | Unknown | Unknown | 17 vs −4% improvement | Unknown | –5.0 (± 14.1 SD) vs –0.3 (± 11.1 SD) | 12 months |
Schuepbach et al., 2013 [23] | STN | 52.9 (±6.6 SD). Maximum age 60). | 7.3 years (± 3.1 SD; minimum 4-year duration) | 17.5 vs 1.2 h | 3.2 vs −1.3 h | 26 vs −11% improvement | 2.1 vs 0.2 h improvement in dyskinesias | –7.8 (± 1.2 SD) vs +0.2 (± 1.1 SD), a 26% improvement vs 1% worsening | 24 months |
a PDQ-39 range is 0–100; higher scores = worse reported quality of life; a negative change represents an improvement.
One of the greatest effects from STN-DBS (unlike pallidal stimulation) is the ability to reduce dopaminergic medication postoperatively, with an average drug dosage reduction of 40–65% [11, 13, 38, 39]. “Off”-period impairment is also reduced; patients assessed on neurostimulation alone have demonstrated over a 40% improvement in their UPDRS score, as well as improvements in ADLs, as evidenced by improvements on the Schwab and England Scale [10]. Overall, the average change in ADL scores estimated by Kleiner-Fishman et al. [35] in a meta-analysis of outcomes was nearly a 50% improvement from baseline, although largely affecting motor aspects of function. Disease progression following STN-DBS appears to continue at the same rate as for those who are medically treated [26]. The long-term effect of STN-DBS stimulation remains beneficial, and the improvements found from stimulation do persist over several years [10, 13].
Dyskinesias are perhaps the source of disability exhibiting the greatest magnitude of improvement after STN-DBS [11, 12], with a 54% reduction in the neurostimulation group found by Deuschl et al. [10] compared with no changes in the medication group. These patients demonstrated a longer duration of “on” time without dyskinesias, gaining on average 4.4 h “on” and 4.2 fewer “off” hours [10]. Rigidity and tremor improved by 70% with STN stimulation, and bradykinesia was reduced by over 50% [13]. Speech and other axial symptoms such as postural reflexes and gait tend not to improve as much as appendicular motor symptoms with STN-DBS [11, 13]. Dysarthria may even be aggravated if stimulation affects the corticobulbar fibers [40]. Cognitive outcomes following STN-DBS remain relatively similar compared with best medical management, although verbal fluency scores are consistently decreased, whereas working memory and processing speed typically are stable [41].
The optimal anatomical location within the STN is still debated. Stimulation of the dorsolateral sensorimotor portion of the STN is more effective for improvement in motor symptoms, but attempts to separate out discrete localizations responsible for tremor, bradykinesia, rigidity and other symptoms have been fraught with inconsistent results. Ultimately, individual patient differences in response to stimulation are the driving force tailoring DBS programming.
Mechanism of action
The technique of STN-DBS requires high-frequency stimulation (greater than 130 Hz) to be effective [42]. Lower frequencies (60–80 Hz) have been utilized in some patients to treat freezing of gait [43], although results have been inconsistent. Low-frequency DBS below 20 Hz typically worsens bradykinesia [42, 44]. High-frequency stimulation of the STN is thought to exert its effects through changes in the pattern of neuronal firing [45], rather than simply changes in the firing rate, as was thought previously. Microelectrode recording in the STN during DBS reveals local field potentials with specific frequencies, which are the product of the synchronicity of neuronal oscillations. In PD, frequencies between 11 and 30 Hz (the “beta band”) have been found to be associated with worsened motor function [46]. These same oscillatory frequencies are attenuated by voluntary movement, dopaminergic medication and DBS [47], and are replaced by low-frequency oscillations (1–7 Hz) whose significance remains unknown; however, they are hypothesized to reflect the effect of electrical polarization around the stimulating electrode that may be normalizing STN hyperactivity [48–50].
Complications and side effects
Compared with medical management, DBS surgery produces a higher frequency of serious adverse events, 13% compared with 4% in the 2006 study by Deuschl et al. [10]. Serious adverse events include seizures in up to 3% of patients, ischemic stroke in nearly 1% of patients, fatal intracranial hemorrhage occurring in about 2–4% of patients and surgical-site infections occurring in up to 8% (Table 19.3) [20, 35, 37, 51]. Device failure is possible as well, with possible complications including lead breaks, dermal erosion, seromas, excessive battery consumption and pulse generator failure [52]. Patient discomfort can be seen, with tightness of the extension lead, scars and subdermal bumps along the duration of the lead [32]. Stimulation-related complications generally result from the unintentional spread of current into neighboring structures surrounding the STN, which can occur in up to 30% of patients [35]. These effects fortunately are often transient, reversible and adjustable with modification of the stimulation parameters (Figure 19.3). Another possible complication of STN-DBS surgery is weight gain, with reports varying from 8.4% [35] to an incidence approaching 100% in some small studies [53]. The cause of this is likely multifactorial and may be influenced by a decrease in dyskinesias or decreased serum cortisol [54], or potentially related to electrode location, with greater risk associated with closer proximity to more medial regions within the STN [55].
Common possible complications of subthalamic nucleus deep-brain stimulation
Complication | Relative risk (%) |
---|---|
Fatal intracranial hemorrhage | 3.9 |
Surgical or device infection | 3.6 |
Device dysfunction | 3 |
Migration of leads | 1.5 |
Seizures | 1.5 |
Ischemic stroke | 1 |
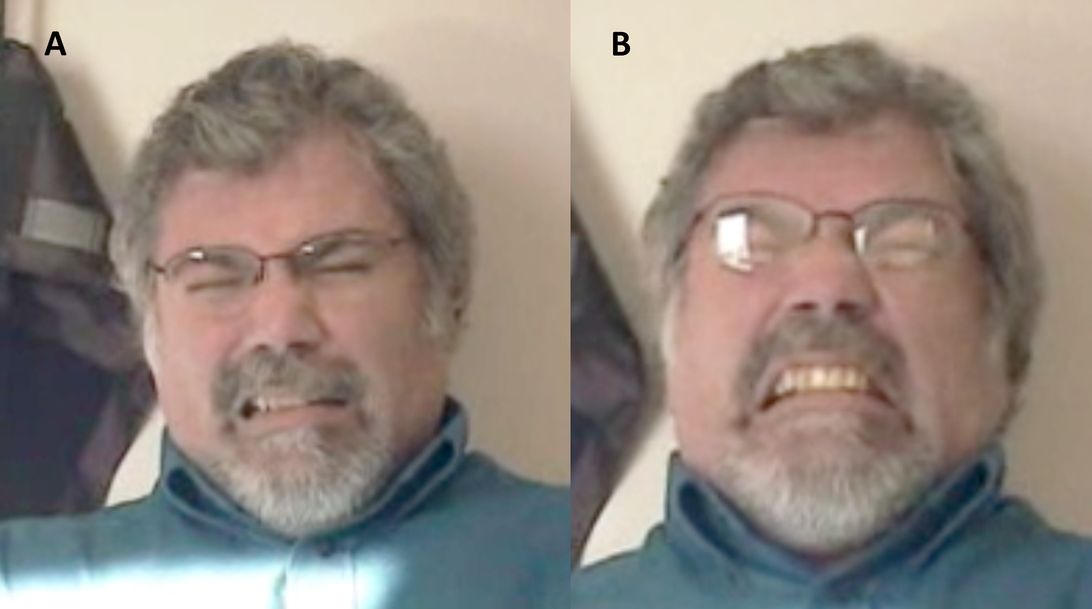
A man with facial dystonia as a stimulation-induced side effect from subthalamic nucleus deep-brain stimulation. Note that the contraction of facial muscles at rest (A) is magnified when attempting to speak (B), a typical expression of focal dystonias. Dystonia substantially improved with lowered stimulation amplitude.
The incidence of certain complications can be prevented or reduced by adhering to strict patient selection criteria, as outlined above. Neuropsychological evaluation is performed to avoid surgery in patients who have significant cognitive impairment or uncontrolled depression. Perioperative confusion and psychosis can be risk factors for further cognitive decline [56]. While uncommon, some patients undergoing STN-DBS have attempted or completed suicide [10, 57]. Rates of attempt have been seen at nearly 0.90% and rate of completion at 0.45% [58]. The reason for such behavior is unclear, although it may be associated with a decrease in the dopaminergic tone, associated with reductions in levodopa and dopamine agonist dosage made possible after surgery, which could contribute to the magnification of postsurgical behavioral disturbances, including depression. Neurosurgery may impact the patients’ perception of themselves and their body, experiencing apathy, a sense of loss of vitality, and strangeness [59]. Furthermore, “in spite of the excellent motor outcome, it is clear that the operation can result in poor adjustment of the patient to his or her personal, family, and socio-professional life” [59]. For this reason, a strong social support network is important to help care for the patient following surgery. For patients requiring a substantial decrease in levodopa dose, monitoring of behavioral “at-risk” changes is warranted. Other complications of STN-DBS exist, including dysexecutive syndrome [41] and psychiatric symptoms [60]. Certain complications have been associated with the location of stimulation within the STN area. Patients who have received stimulation being slightly too ventral, closer to the limbic system, have experienced postsurgical hypomania, typically transient or alleviated with a change in DBS programming [61, 62]. Generally, patients who experience cognitive decline postsurgically have been found to have electrode placements with deeper and more posterior/ventral contacts [56].
Future uses of subthalamic nucleus deep-brain stimulation
The future of STN-DBS may include a wider opportunity for patients to be considered eligible and more patient-specific programming. Animal data had provided optimism that STN-DBS could be neuroprotective [63, 64], although supportive data in humans have been so far elusive. Based on prior studies demonstrating that those with “early” motor complications receiving STN-DBS with best medical therapy versus medical therapy alone had an improved quality of life in the DBS group [23], future research endeavors will examine the extent to which early STN-DBS may have disease-modifying properties. The intermediate-frequency range between 60 and 80 Hz is being evaluated for possible improvements in freezing and gait [43]. With research into specific frequency-related treatments comes the potential for clinical trials into adaptive technology, such as an adaptive or “closed-loop” DBS [65], which has been demonstrated in primate models to be more effective than the standard open-loop treatment [66]. The ability to shape electrical fields to focus stimulation in desired structures and minimize side effects is being developed with the use of segmented electrodes [67, 68]. With the greater complexity of programming DBS electrodes that have an increased number of contacts or segmented contacts, it is likely that software will assist the neurologist with steering the DBS current in the intended direction and translating this into the appropriate parameters.
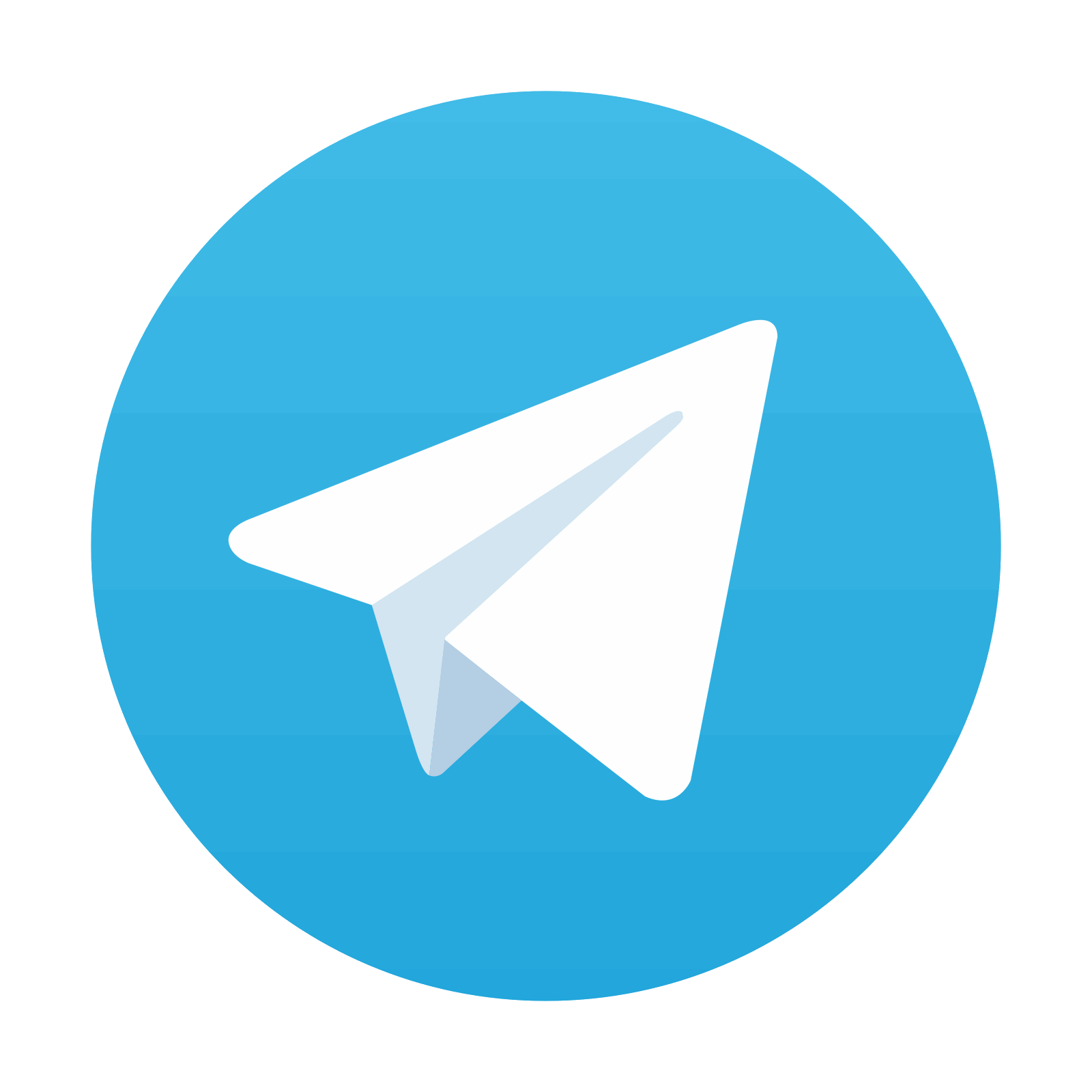
Stay updated, free articles. Join our Telegram channel

Full access? Get Clinical Tree
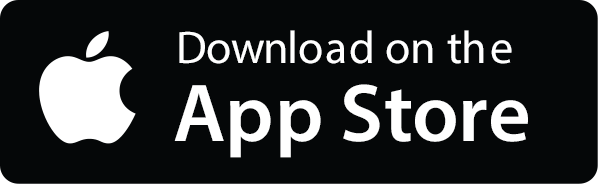
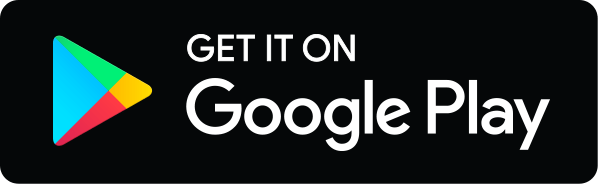