Abstract
Degenerative disorders of the newborn are relatively rare. Nonetheless, they are important to recognize because a few are treatable when diagnosed early. Dividing these disorders into those that primarily affect gray matter, white matter, or both is useful. Disorders of gray matter are characterized by seizures, myoclonus, spikes, or sharp activity on electroencephalogram, failure of cognitive development, and retinal disease. Some are accompanied by visceral storage including GM 1 gangliosidosis, GM 2 gangliosidosis, Niemann-Pick disease, Gaucher disease, Farber disease, and infantile sialic acid storage disease. Other gray matter disorders such as Tay-Sachs disease, congenital neuronal ceroid-lipofuscinosis, Alpers disease, and Menkes disease lack visceral storage. White matter disorders are characterized by marked motor deficits and slow activity on electroencephalogram and include Canavan disease, Alexander disease, Krabbe disease, Pelizaeus-Merzbacher disease, and Aicardi-Goutieres disease. Disorders that affect both white and gray matter often target subcellular structures (mitochondria, peroxisomes), target amino acid or neurotransmitter synthesis pathways, and can have regional selectivity for the cerebellum, pons, or basal ganglia. Examples include neonatal adrenoleukodystrophy, Zellweger syndrome, Leigh syndrome, mitochondrial encephalopathies, congenital disorders of glycosylation, pontocerebellar hypoplasias, neurotransmitter defects including serine synthesis deficiency, and Rett syndrome in males. We discuss each of these disorders briefly, focusing on clinical features, diagnosis, genetics, neuropathology, neuroimaging findings, current treatments, and emerging therapies.
Keywords
neurodegenerative, Tay-Sachs disease, congenital neuronal ceroid-lipofuscinosis, Alpers disease, Menkes disease, GM 1 gangliosidosis, GM 2 gangliosidosis, Niemann-Pick disease, Gaucher disease, Farber disease, infantile sialic acid storage disease, Canavan disease, Alexander disease, Krabbe disease, Pelizaeus-Merzbacher disease, Aicardi-Goutieres disease, peroxisomal disorders, neonatal adrenoleukodystrophy, Zellweger syndrome, mitochondrial disorders, Leigh syndrome, mitochondrial encephalopathies, congenital disorders of glycosylation, pontocerebellar hypoplasia, neurotransmitter defects, serine synthesis deficiency, Rett syndrome, glucose transporter deficiency
Certain degenerative disorders of the developing nervous system may be clinically manifested in the neonatal period. Because most of these disorders are related to a disturbance in the metabolism of a lipid or some other compound, they are discussed most appropriately in this series of chapters concerned with metabolic disorders. Indeed, clinical overlap of some of these degenerative disorders with some of the metabolic disorders discussed in previous chapters will be apparent. Nevertheless, the diseases discussed in this chapter are best considered as a more or less distinct group. Early diagnosis is important for the delineation of prognosis, genetic counseling, and, in a few instances, the institution of specific therapy. Because these are relatively rare disorders, discussion of each entity is brief.
Major Disorders
From the clinical standpoint, we find it useful to separate the degenerative disorders into those that primarily affect gray matter and those that primarily involve white matter ( Table 29.1 ). (At later ages, disorders that affect specific regions of the brain [e.g., basal ganglia in Huntington or Wilson disease, or cerebellum in ataxia telangiectasia], so-called system degenerations, comprise a third major category.) In general, disorders of gray matter are characterized by the appearance early in the disease of seizures, myoclonus, spikes, or sharp activity on the electroencephalogram, failure of cognitive development ( dementia ), and retinal disease; whereas disorders of white matter are characterized by the appearance early in the disease of marked motor deficits and slow activity on the electroencephalogram. Although overlap in the clinical features and even in the topography of the neuropathology between the two broad categories is considerable, we retain the separation. There are a few disorders involving specific subcellular organelles (e.g., peroxisomes, mitochondria) or other, still-to-be-defined defects that affect both gray and white matter prominently, and these must be considered separately (see Table 29.1 ). This chapter focuses only on those diseases for which the recording of more than a few cases with neonatal manifestations is available. The salient features of the disorders are outlined in Tables 29.2 to 29.5 . Many of these disorders are abnormalities of degradation of sphingolipids ( Fig. 29.1 ), as noted in the individual discussions later.
Gray matter |
No visceral storage |
Tay-Sachs disease (GM 2 gangliosidosis) |
Congenital neuronal ceroid-lipofuscinosis |
Alpers disease |
Menkes disease |
With visceral storage |
GM 1 gangliosidosis |
GM 2 gangliosidosis (Sandhoff variant) |
Niemann-Pick disease |
Gaucher disease |
Farber disease |
Infantile sialic acid storage disease |
White matter |
Canavan disease |
Alexander disease |
Krabbe disease |
Pelizaeus-Merzbacher disease |
Leukodystrophy with cerebral calcifications and cerebrospinal fluid pleocytosis (Aicardi-Goutieres disease) |
Gray and white matter |
Peroxisomal disorders: neonatal adrenoleukodystrophy, Zellweger syndrome |
Mitochondrial disorder: Leigh syndrome, other mitochondrial encephalopathies |
Disorders with cerebellar ± pontine hypoplasia
|
Neurotransmitter defects
|
Rett syndrome (males) |
DISEASE | CLINICAL FEATURES | METABOLIC-ENZYMATIC FEATURES | PATHOLOGY |
---|---|---|---|
Tay-Sachs disease (GM 2 gangliosidosis) | Stimulus-sensitive myoclonus, irritability, hypotonia, weakness, cherry-red macula (virtually all); later seizures, blindness, and macrocephaly | GM 2 ganglioside accumulation in brain; hexosaminidase A deficiency | Neurons distended by GM 2 ganglioside throughout the CNS |
Congenital neuronal ceroid-lipofuscinosis | Neonatal seizures (severe), apnea, microcephaly, developmental arrest, followed by regression and vegetative state | Cathepsin D deficiency | Marked brain atrophy; diffuse neuronal loss with gliosis, autofluorescent granular material in neurons, glia, and macrophages, electron microscopy—osmiophilic granules |
Alpers disease | Myoclonus (often stimulus-sensitive), seizures (severe), developmental arrest followed by regression, visual and auditory deficits common, evidence of hepatic dysfunction late | Elevated lactate/pyruvate levels in cerebrospinal fluid (and blood); deficient catalytic subunit of mitochondrial DNA polymerase gamma (mutated gene POLG ) with mitochondrial DNA depletion and impaired function of electron transport chain at multiple sites; POLG-negative cases (with apparent defect in mitochondrial tRNA metabolism) now recognized especially in the perinatal period | Marked brain atrophy; severe neuronal loss with astrocytosis, spongiosis, and capillary proliferation, especially in the cerebral cortex, and particularly occipital (striate) cortex |
Menkes disease (kinky hair disease) | Hypothermia, hypotonia, poor feeding, poor weight gain, seizures, developmental arrest and then regression; cherubic face; colorless, friable, steely hair | Low serum copper and ceruloplasmin levels; cultured fibroblasts: reduced efflux of copper, elevated copper content; deficient activity of many copper-containing enzymes; molecular defect of a cation transporting ATPase | Neuronal loss with gliosis in cerebral cortex and cerebellum, marked proliferation of dendritic tree (especially of Purkinje cells), focal axonal swellings ( torpedoes ); myelin loss with gliosis and arterial changes |
Epileptic syndromes (see Chapter 12 ) |
Early infantile epileptic encephalopathy (Ohtahara syndrome) |
Early myoclonic epilepsy |
Pyridoxine-dependent seizures/folinic acid-responsive seizures |
Pyridoxal-5-phosphate-responsive seizures |
DEND syndrome |
Hyperinsulinism/hyperammonemia syndrome |
Malignant migrating partial seizures of infancy |
Metabolic disorders |
Glucose transporter deficiency a |
Serine synthesis deficiency b |
Nonketotic hyperglycinemia (see Chapter 27 ) |
Sulfite oxidase deficiency: molybdenum cofactor deficiency (see Chapter 28 ) |
Multiple carboxylase deficiency: biotinidase deficiency (see Chapter 28 ) |
Multiple acyl-coenzyme A dehydrogenase deficiency (glutaric aciduria type II) (see Chapter 28 ) |
a Described in this chapter in the section on neonatal seizure disorders that mimic gray matter degeneration at presentation.
b Described in this chapter later among diseases affecting gray and white matter (see Table 29.7 ).
PARAMETER | DISEASE |
---|---|
Cells (increased) | Aicardi-Goutiere leukodystrophy |
Glucose (decreased) | Glucose transporter defect |
Protein (increased) | Krabbe disease |
Lactate (increased) | Mitochondrial disease, organic acid disorder, fatty acid disorder ( Chapters 27 and 28 ) |
Glycine (increased) | Nonketotic hyperglycemia ( Chapter 27 ) |
Serine (decreased) | Serine synthesis deficiency |
Neurotransmitter metabolites (variably increased) | Neurotransmitter defects |
AASA (increased) | Pyridoxine-dependent seizures ( Chapter 12 ) |
Folinic acid metabolites (increased) | Folinic-acid responsive seizures ( Chapter 12 ) |
Pyridoxal-5-phosphate (increased) | Pyridoxal-5-phosphate dependent seizures ( Chapter 12 ) |
DISEASE | CLINICAL FEATURES | METABOLIC-ENZYMATIC FEATURES | PATHOLOGY |
---|---|---|---|
GM 1 gangliosidosis | Sucking and swallowing impairment, hypotonia, decreased movement; edema, coarse facies, hepatosplenomegaly, cherry-red macula (50%) | GM 1 ganglioside accumulation in brain, beta-galactosidase deficiency | Neurons distended by GM 1 ganglioside throughout the CNS |
GM 2 gangliosidosis (Sandhoff variant) | See Tay-Sachs disease; also hepatosplenomegaly | See Tay-Sachs disease; also globoside accumulates in viscera, hexosaminidase A and B deficiency | See Tay-Sachs disease |
Niemann-Pick disease (type 1A, infantile ) | Feeding impairment, failure to thrive, developmental arrest and then regression, cherry-red macula (50%), hepatosplenomegaly | Sphingomyelin (and cholesterol) accumulate in brain; sphingomyelinase deficiency | Neurons distended with sphingomyelin throughout the CNS; foam cells in leptomeningeal and perivascular spaces |
Gaucher disease (type 2, acute neuronopathic or infantile ) | Retrocollis, strabismus, trismus, dysphagia, aspiration, spasticity, hepatosplenomegaly, hydrops fetalis | Glucocerebroside accumulates in brain; glucocerebrosidase (beta-glucosidase) deficiency | Gaucher cells (lipid-laden histiocytes) in perivascular spaces and in parenchyma; neuronal death and neuronophagia throughout the CNS, especially in the brain stem |
Farber disease (lipogranulomatosis) type 1, classic | Painful swelling of joints, (later periarticular nodules), hoarse cry, feeding disturbance, failure to thrive, hypotonia, muscle atrophy, areflexia or hyporeflexia, and hepatomegaly (50%) | Ceramide accumulates in tissues; ceramidase deficiency | Neurons distended by glycolipid (ceramide?), especially in anterior horn cells, less prominently in brain stem, basal ganglia, and least in cortex |
Infantile sialic acid storage disease (also sialidosis type II, and galactosialidosis) | Fetal ascites/hydrops, neonatal hypotonia, impaired feeding, developmental arrest followed by regression; ascites, coarse facies, white hair, and hepatosplenomegaly | Sialic acid accumulates in tissues, including the brain, and in body fluids; defect of sialic acid transport across lysosomal membrane; similar phenotype with sialidosis secondary to deficiency of alpha-neuraminidase (sialidosis type II) or of alpha-neuraminidase and beta-galactosidase (galactosialidosis) | Neurons distended with sialic acid, especially in the diencephalon, brain stem, and spinal cord; prominent axonal spheroids; myelin deficiency |

Disorders Primarily Affecting Gray Matter
Disorders primarily affecting gray matter are best discussed according to the presence or absence of accompanying visceral storage. Visceral storage is clinically identified by hepatosplenomegaly and is often accompanied by abnormalities of the long bones and by coarse facial features.
No Visceral Storage
Tay-Sachs Disease
Tay-Sachs disease is the prototype of a degenerative disease of gray matter in infancy (see Table 29.2 ). Onset in the first few weeks of life, although uncommon, may occur. (More commonly, onset is at approximately 3 months.) The principal initial clinical features are irritability and hypersensitivity to auditory and often other sensory inputs. A cherry-red spot, caused by ganglioside storage in retinal ganglion cells imparting a yellowish tint around the normally red fovea, is apparent on funduscopic examination. This abnormality has been identified as early as 2 days of age. The subsequent course is characterized by myoclonic seizures, motor deterioration, hypotonia, blindness, macrencephaly, and death in the third or fourth year of life, which are the features confirmed in a recent natural history study of more than 200 infantile Tay-Sachs patients. (A clinical variant with organomegaly and bony abnormalities similar to those in generalized GM 1 gangliosidosis [see later discussion] is termed a Sandhoff variant of Tay-Sachs disease. ) The diagnosis is established by identification of the enzymatic defect in white blood cells or fibroblasts, which involves hexosaminidase A (see Fig. 29.1 ). In cases where enzyme testing is indeterminate molecular analysis may be necessary. (In the Sandhoff variant, both hexosaminidase A and B are deficient.) The disorder is inherited in an autosomal recessive manner and is especially common in Ashkenazi Jews, although the majority of cases in North America are now of non-Jewish ancestry. Neuropathology is characterized by generalized neuronal storage of the GM 2 ganglioside and by markedly dilated neuronal processes (meganeurites). Neuroimaging (magnetic resonance imaging [MRI]) shows an abnormal signal in the thalamus and, subsequently, marked atrophy of the cerebral cortex and deep nuclear structures; cerebral white matter shows an increased T2 signal. Although no known therapy exists, genetically engineered neural progenitor cells have been shown to correct the enzymatic defect in co-cultured human Tay-Sachs fibroblasts and to secrete active enzyme throughout the fetal and neonatal mouse brain after cellular transplantation.
Recent gene therapy studies are also encouraging. A single intravenous (IV) injection of recombinant adeno-associated virus 9 encoding the hexosaminidase B gene administered to the neonatal Sandhoff disease mouse reduces GM 2 ganglioside storage and inflammation, corrects motor function, and prolongs long-term survival. Similar beneficial findings have been shown with intracranial viral injections in a feline model. The serendipitous finding of a naturally occurring mutation in the hexosaminidase A gene in Jacob sheep offers a unique opportunity to study gene therapy for Tay-Sachs disease in a large animal model. Because clinical trials could follow in humans, the urgency is increased for early diagnosis. Prenatal diagnosis can be made readily by enzymatic analysis for hexosaminidase A in cultured amniotic fluid cells or chorionic villus samples. DNA analysis of the hexosaminidase A gene is also possible if disease-causing mutations have been identified in both parents. Attempted therapy with bone marrow transplantation has not altered the course of the disease.
Congenital Neuronal Ceroid-Lipofuscinosis
Neuronal ceroid-lipofuscinosis consists of a group of neuronal degenerative disorders characterized by an accumulation of the lipopigments ceroid and lipofuscin. At least 13 mutant genes and 6 clinical forms are now recognized. The form of most relevance in this context is congenital neuronal ceroid-lipofuscinosis (Norman-Wood disease, CLN-10). This form should be distinguished from the more common and well-known early infantile disorder (Haltia-Santavuori disease, CLN-1). In the latter disorder, the usual age of onset is 6 to 18 months. The rarer congenital form is apparent at birth (see Table 29.2 ), and at least 11 cases have been reported. The clinical phenotype is dramatic. Postnatal respiratory insufficiency, severe neonatal seizures, and microcephaly are nearly consistent clinical features. Failure of neurological development and the development of a vegetative state, usually with recalcitrant seizures, are followed by death, usually in the first days or weeks of life. The absence of electroretinographic responses and the development of an isoelectric electroencephalogram are characteristic. Neuroimaging (MRI) shows marked and progressive atrophy of the cerebral cortex, thalamus, and striatum ( Fig. 29.2 ). The diagnosis should be considered in a newborn with severe seizures and microcephaly of unknown origin. Diagnosis is initially based on the identification of autofluorescent lipopigments in lymphocytes, skin, or rectal mucosa, which present as granular material on an electron microscopic examination. DNA sequencing is available to confirm the diagnosis. Neuropathological findings are characterized by diffuse neuronal loss ( Fig. 29.3 ) with an accumulation of the lipopigment granules of ceroid-lipofuscin in neurons, glia, and macrophages. Marked infiltration with astrocytes and microglia is apparent. The molecular defect involves the cathepsin D gene, which is present in a homozygous form in patients. The encoded protein, cathepsin D, is a lysosomal protease, which is important for proper degradation and clearance in lysosomes. Although the infantile form is caused by mutations in the CLN1 gene-encoding palmitoyl-protein thioesterase-1, there is a secondary deficiency in cathepsin D, thereby biochemically linking the infantile and congenital forms of the disease.


Alpers Disease
The term Alpers disease , inappropriately applied in the past to a heterogeneous group of disorders, refers to those relatively uncommon examples, usually familial and consistent with autosomal recessive inheritance, of a progressive degenerative disease of gray matter without neuronal storage or other pathognomonic cytological features, and with subsequent hepatic disease. Affected infants exhibit the clinical hallmarks of gray matter disease, seizures, and myoclonus (often stimulus sensitive) in the first weeks and months of life (see Table 29.2 ). Hypotonia and vomiting are also prominent features. In one series, 4 of 26 infants had clear onset within the first 2 months of life. Hepatic disease becomes apparent usually after 9 months of age (mean age, 35 months), but a more frequent assessment of serum transaminase levels before the appearance of hepatomegaly demonstrates hepatic dysfunction earlier. Most infants die by 3 years of age. MRI shows extensive cerebral atrophy ( Fig. 29.4 ).

Neuropathological study shows striking cortical neuronal loss with spongy change and gliosis, which is worse in the deeper cortical layers and is especially prominent in the striate cortex ( Fig. 29.5 ). Frequently, capillary proliferation is apparent, and the constellation of pathological change resembles that of Leigh disease, a mitochondrial disorder (see later discussion). Earlier data suggested that Alpers disease was a mitochondrial disorder because of the findings in several study series of patients of elevated blood and cerebrospinal fluid (CSF) lactate and various abnormalities of the electron transport chain. More recently, some cases have been shown to involve mutations in the gene encoding the catalytic subunit of mitochondrial DNA polymerase γ ( POLG ). The result is mitochondrial DNA depletion and impaired function of the electron transport chain at multiple sites. However, POLG1-negative cases exist and, notably, these appear to have especially a perinatal onset characterized by seizures, spasticity, progressive microcephaly, and severe intellectual disability. The genetic etiology of the POLG1-negative cases remains largely unknown, although whole exome sequencing has identified mutations in mitochondrial tRNA synthetases, which are critical for efficient mitochondrial protein synthesis. Most recently, it has been suggested that the use of the term Alpers syndrome be confined to the POLG1-negative infantile form, and that cases with POLG1 mutations, hepatic dysfunction, and typically later onset be better termed Alpers-Huttenlocher syndrome .

Menkes Disease
Menkes disease (kinky or steely-hair disease, trichopoliodystrophy) is an X-linked disorder of copper metabolism with onset in the severe form of the disease characteristically in the neonatal period. Premature delivery, a cherubic face, hypothermia, and hyperbilirubinemia are common neonatal clinical features (see Table 29.2 ). Hypotonia, lethargy, poor feeding, neurological deterioration, and seizures develop promptly. In the neonatal period, the hair is usually fine and colorless, but shortly thereafter, the more characteristic, friable, kinky appearance (feeling like fine sandpaper) develops ( Fig. 29.6 ). We have noted the sandpaper feel in the neonatal period, however. Recalcitrant seizures and neurological deterioration lead to death, usually in the second year. Diagnosis is confirmed by the finding of low serum copper and ceruloplasmin levels; in the early neonatal period, serum values may be normal or elevated but decline over the ensuing weeks, whereas in normal infants, serum values increase postnatally. Because of the partial deficiency of dopamine-β-hydroxylase in Menkes disease, the measurement of plasma neurometabolites (dopamine, norepinephrine, dihydroxyphenylacetic acid, and dihydroxyphenylglycol) can be diagnostic in the neonatal period. Definitive molecular diagnosis is now available, supporting the clinical and biochemical results, particularly in neonatal cases where interpretation sometimes can be challenging. Studies of cultured fibroblasts show increased retention and reduced efflux of labeled copper, which are features that can be used for prenatal diagnosis by the study of cultured amniotic fluid cells (second trimester) or chorionic villus samples (first trimester). However, the preferred method is molecular testing for specific mutations identified in the parents.

Neuropathological examination shows striking cortical neuronal loss, gliosis, and subcortical myelin loss associated with severe axonal degeneration (see Table 29.2 ). Axonal changes are especially marked in cerebellum. The evolution of the cerebral parenchymal changes is followed best by MRI scans ( Figs. 29.7 and 29.8 ). Intracranial arterial abnormalities are characteristic (see Fig. 29.8 ). The latter have been identified as striking tortuosities around the circle of Willis by MR angiography as early as 5 weeks of age. The essential biochemical defect in the disease involves copper transport across specific cellular compartments (i.e., the placenta, gastrointestinal tract, and blood-brain barrier), with a resulting failure of formation of copper-containing enzymes. The latter include tyrosinase (causing depigmentation of hair), lysyl oxidase (causing defective elastin-collagen cross-linking, and arterial intimal defects), superoxide dismutase (causing vulnerability to free radicals), cytochrome oxidase (causing impaired energy production), and dopamine-beta-hydroxylase (causing impaired catecholamine synthesis). The latter three defects perhaps are most important for the neurological phenomena. The responsible gene is located on the X-chromosome (Xq13), and the mutant protein is a cation transporting adenosine triphosphatase (ATP7A). More than 300 unique mutations have been identified thus far ( http://www.LOVD.nl/ATP7A ). Attempts to correct the copper deficiency in brain have included parenteral administration of copper histidine. Clinical response, although inconsistent, has been occasionally promising. Response to therapy depends on the ATP7A genotype and the initiation of treatment in the neonatal period enhances survival and neurodevelopmental outcome. However, severe ATP7A mutations still have a poor prognosis even when therapy is initiated in utero.


Disorders Mimicking Gray Matter Degeneration
Several neonatal disorders in which seizures are a prominent manifestation may mimic onset of a gray matter degeneration (with no visceral storage) (see Table 29.3 ). It is critical to recognize these disorders partly because early management may require specific interventions. The group is best divided into recognized epileptic syndromes and certain metabolic disorders (see Table 29.3 ). The epileptic syndromes are reviewed in Chapter 12 , and the metabolic disorders are primarily discussed in Chapters 27 or 28 . One metabolic disorder, the glucose transporter defect, will be discussed here. Another metabolic disorder, serine synthesis deficiency, exhibits prominent white matter abnormalities, in addition to seizures, and thus is discussed later in this chapter (see Disorders Affecting Gray and White Matter ).
Glucose Transporter Defect
Defects in the glucose transporter 1 cause inadequate shuttling of glucose from the blood to the brain, resulting in hypoglycorrhachia (low CSF glucose) in the setting of adequate serum glucose, ultimately leading to energy failure in the brain (see Table 29.4 ). The majority of patients present in the first few months of life with seizures, and notably, diagnosis can be considerably delayed without measuring fasting CSF and blood glucose concentrations. Initial clinical features include intractable seizures, eye movement abnormalities, changes in muscle strength or tone, and breathing abnormalities. In the absence of treatment, the disorder progresses to spasticity, dystonia, ataxia, intellectual disability, and acquired microcephaly with a broad phenotypic spectrum. In addition to measuring CSF glucose, diagnosis now can be confirmed by DNA sequencing of the SLC2A1 gene encoding Glut1 protein. Brain imaging is not usually informative, although it may be useful in monitoring improvements in myelination after the initiation of treatment. Treatment with the ketogenic diet is effective at controlling seizures and may also have a neuroprotective effect leading to improved developmental outcomes. By converting brain energy metabolism to ketosis, the need for glucose is circumvented. Thus, this metabolic disorder can mimic many features of gray matter degeneration, but is largely reversed with the early initiation of the ketogenic diet.
Gray Matter Degenerations With Visceral Storage
Six neuronal degenerations with infantile onset are accompanied by prominent visceral storage (see Tables 29.1 and 29.5 ). Although these disorders may eventually exhibit the hallmark of a neuronal process (seizures), this feature often appears later in the course of the disease or even not at all. Thus, in this group of disorders, it is often difficult in the neonatal period or in early infancy to recognize the disease as one primarily affecting neurons. However, other clinical features are usually distinctive enough to lead to a high degree of suspicion of the correct diagnosis (see later discussions).
GM 1 Gangliosidosis
Manifestations of the generalized form of GM 1 gangliosidosis commonly appear in the first weeks of life (see Table 29.5 ). Clinical features include abnormalities of sucking and swallowing, hypotonia, and a cherry-red spot. Seizures, the hallmark of gray matter disease, are usually not present until after 1 year of age. Generalized edema is a striking early feature. Because of the systemic storage of mucopolysaccharide, coarse facies, subperiosteal bony abnormalities, and hepatosplenomegaly are present. Dermal melanocytosis also can be observed because the accumulation of the GM 1 ganglioside in neural crest cells causes the aberrant migration of melanocytes into the dermis ( Fig. 29.9 ). Progression to death in the second year is characteristic. Diagnosis is based on the identification of the enzymatic defect in white blood cells or fibroblasts, which involves beta-galactosidase (see Fig. 29.1 ). The gene is located on chromosome 3, and an increasing number of mutations have been identified, but no clear genotype–phenotype relationship has been established. The disorder is inherited in an autosomal recessive manner. Neuropathology is characterized by the generalized neuronal storage of the GM 1 ganglioside and by the meganeurites noted for Tay-Sachs disease. Neuroimaging (MRI) , as for Tay-Sachs GM 2 gangliosidosis, initially shows an abnormal signal in the thalamus, and subsequently marked cerebral cortical and deep nuclear atrophy; cerebral white matter shows an increased T2 signal. Neuronal storage and elevated brain ganglioside content have been observed as early as 22 weeks of gestation. This observation has important implications concerning the need for intervention during fetal life with enzyme or gene replacement therapy, when such therapy is further developed. Intracranial gene therapy in a feline model of GM 1 gangliosidosis increases survival and normalizes neurological symptoms when administered before disease onset, and similar results have been observed in adult mouse models with systemic viral injections. Recently, a small prospective study of six patients suggested that combined therapy with miglustat (a small molecule that reduces GM 1 and GM 2 gangliosides) and the ketogenic diet may have a small benefit on survival but will need to be replicated. Prenatal diagnosis of the enzymatic defect is possible by the analysis of cultured amniotic fluid cells or by the DNA sequencing of previously identified mutations.

GM 2 Gangliosidosis (Sandhoff Variant)
GM 2 gangliosidosis , or the Sandhoff variant of Tay-Sachs disease, is clinically similar to Tay-Sachs disease except for the addition of visceromegaly. The major features were reviewed earlier in relation to Tay-Sachs disease. In this disorder, not only is GM 2 ganglioside stored in the brain because of the defect in hexosaminidase A, but also globoside is stored in the viscera, because the enzymatic defect involves both hexosaminidase A and B. Both isoforms are required for the removal of the terminal N -acetylglucosamine from globoside (see Fig. 29.1 ).
Niemann-Pick Disease
The acute infantile form of Niemann-Pick disease may be noted in the first weeks of life (see Table 29.5 ). Feeding difficulties are common, and a cherry-red spot is apparent in nearly 50% of cases. Hepatosplenomegaly, caused primarily by the storage of sphingomyelin, is prominent. Neurological features are often not pronounced until several weeks or months of age, when developmental arrest and then regression occur. Seizures are not a common feature early in the disease. Death occurs most frequently in the first several years. This form of disease is classically referred to as type A. Diagnosis is established by identification of the enzymatic defect in white blood cells or fibroblasts, which involves a diminution of acid sphingomyelinase (ASM) activity to less than 10% of normal (see Fig. 29.1 ). The disorder is inherited in an autosomal recessive manner. Neuropathology is characterized by neuronal storage of sphingomyelin, which is most prominent in the cerebellum, brain stem, and spinal cord. Foam cells, laden with lipid and representing macrophages, are prominent in the meninges and perivascular spaces. Prenatal diagnosis is possible by the identification of the enzymatic defect in cultured amniotic fluid cells or chorionic villus samples or by DNA analysis if pathogenic mutations have been previously identified in the parents. Attempted therapy with bone marrow transplantation has not altered the course of the disease. Recent studies suggest that recombinant heat shock protein (HSP) 70 stabilizes the lysosomal membrane and increases the activity of sphingomyelinase, but preliminary work needs validation in an animal model.
Gaucher Disease
The infantile neuronopathic form of Gaucher disease (type 2) is noted in the neonatal period in 10% of cases. The most common presenting clinical features are retrocollis (hyperextension of neck), strabismus (or other oculomotor abnormalities), and spasticity (see Table 29.5 ). Other brain stem signs, dysphagia and trismus, are common. Hepatosplenomegaly secondary to the storage of glucocerebroside is present. Dermatological findings, particularly thin, reflective skin characterized as collodion, cellophane, or ichthyosis, are common in neonatal cases ( Fig. 29.10 ). A particularly severe form with hydrops fetalis and arthrogryposis has been recognized. The subsequent course of infants with neonatal Gaucher disease generally fulminates with death by 2 or 3 months of age. Diagnosis is based on identification of the enzymatic defect in the white blood cells, fibroblasts, or liver, which involves beta-glucosidase (see Fig. 29.1 ), produced by mutations in the glucocerebrosidase gene (GBA). DNA sequencing is now available, with more than 300 GBA mutations identified. The disorder is inherited in an autosomal recessive manner. Neuropathology is characterized by little or no neuronal storage of glucocerebroside, but neuronal loss is present, especially in the brain stem. The basal ganglia and the cerebellum also are particularly involved. Gaucher cells, lipid-laden histiocytes, often with the cytoplasmic appearance of wrinkled tissue paper, are present in perivascular spaces and are free in brain parenchyma. Neuronal death with microglial nodules and gliosis appears to occur in proximity to these cells, a finding suggesting the possibility of a toxic effect from the stored lipid or a product thereof (glucosylsphingosine?). Prenatal diagnosis is possible by the identification of the enzymatic defect in cultured amniotic fluid cells or chorionic villus specimens. Molecular genetic testing is also available when both disease-causing mutations in a family are known. The possibility of therapy in less severely affected infants is suggested by successes in patients with the nonneuronopathic forms of Gaucher disease who were treated with recombinant human macrophage-targeted glucocerebrosidase. Other approaches in nonneuronopathic cases have included drugs that decrease glucocerebroside biosynthesis, bone marrow transplantation, or the introduction of somatic cells (e.g., fibroblasts) into the bone marrow or other sites into which the normal gene for glucocerebrosidase was introduced by retrovirally mediated gene transfer. Theoretically, the correction or arrest of the neurological disease can be accomplished because this disorder involves the reticuloendothelial system, particularly the bone marrow, and thus entry of the transplanted cells into the central nervous system (CNS) (i.e., the major limitation of gene therapy of other lysosomal disorders) may not be required. However, a novel mouse model of this disorder suggests that while the microglia of hematopoietic origin might influence disease progression, a deficient enzyme in CNS cells underlies the neuronopathic disorder. Bone marrow approaches are therefore unlikely to be curative. Gene therapy directed at the brain will be necessary and has been successful in mouse models. A recent study has also suggested the involvement of programmed necrosis (necroptosis), which is dependent on receptor-interacting protein kinase-3 (RIPK3) as a primary mechanism of neuronal death in animal models of Gaucher. The autopsy of a single patient with infantile-onset type 2 showed increased RIPK3 in neurons, suggesting that targeted inhibition of RIPK3 may be a novel therapeutic approach. Chemical chaperone drug therapy is another area of active investigation involving the drug binding of misfolded glucocerebrosidase, allowing proper function and trafficking to lysosomes.

Farber Disease
In the classic type 1 form of Farber disease , as with most other neuronal degenerations with visceral storage, determining that gray matter is primarily involved may be difficult. Thus, the initial clinical features are primarily painful swelling of joints, subcutaneous nodules, and hoarse cry (see Table 29.5 ). Subsequently, feeding disturbance leading to failure to thrive is prominent. Perhaps the most pronounced neurological features are hypotonia, weakness, muscle atrophy, and areflexia or hyporeflexia, which probably reflect the involvement of anterior horn cells and peripheral nerve roots. Consistent with the latter involvement is the finding of elevated CSF protein levels in nearly all cases, and electromyographic findings of denervation. Hepatomegaly is prominent in approximately 75% of cases. Disturbances of swallowing, aspiration, and pulmonary disease lead to death at 1 to 2 years of age. Ceramide accumulates in tissue. Neuronal storage occurs, especially in the anterior horn cells and the brain stem. Diagnosis is established by detection of the marked defect in ceramidase in cultured fibroblasts (see Fig. 29.1 ). Genetic characterization of mutations in the ASAH1 gene (which codes for ceramidase) are under way and have primarily identified missense mutations, although deletions have also been found. Interestingly, mutations in this gene have also been found in patients with spinal muscular atrophy with myoclonic epilepsy, raising the possibility that there may be more Farber disease patients than previously recognized. Prenatal diagnosis is possible by the analysis of cultured amniotic fluid cells or by molecular genetic testing. Attempted therapy by bone marrow transplantation has provided some benefit for the regression of nodules (which contain ceramide-laden macrophages) and associated joint pain, but has no apparent neurological benefit.
Insertion of a human ASAH1 mutation into mice has created a model of Farber disease that recapitulates many characteristics of the human disease. Studies with this model demonstrated that systemic (but not CNS) manifestations of disease could be corrected by neonatal IV injection of a lentiviral vector expressing human acid ceramidase. This novel tool may provide new avenues to study mechanisms and interventions for the neurological symptoms. Finally, recombinant acid ceramidase is currently under development by Plexcera Therapeutics but requires validation in planned clinical trials and is not expected to ameliorate disease in the CNS.
Infantile Sialic Acid Storage Disease (and Sialidoses)
Sialic acid is a critical component of the oligosaccharide portion of many glycoproteins. Two varieties of disturbance of sialic acid metabolism may result in neonatal disease with neuronal storage. One of these is related to a disorder of sialic acid transport and is sometimes referred to as infantile sialic acid storage disease or sialuria . The other disturbance is related to a defect in the lysosomal enzyme that degrades the oligosaccharides containing sialic acid (i.e., neuraminidase or sialidase) and is often referred to as sialidosis . The prototypical clinical presentation of both infantile sialic acid storage disease and sialidosis with onset in utero or the neonatal period includes generalized edema (including fetal ascites/hydrops), feeding disturbance, marked hepatosplenomegaly, hypotonia, coarse dysmorphic facies, and radiographic abnormalities of the long bones ( Fig. 29.11 ). In infantile sialic acid storage disease, thin, white hair is a consistent feature (see Fig. 29.11 ), not noted in the sialidoses. Severe anemia, failure to thrive, developmental arrest, and then regression subsequently develop. Diagnosis of the infantile sialic acid storage disorder or sialuria (i.e., the disorder of lysosomal transport of sialic acid) is made by the identification of large increases in free sialic acid in plasma and urine, and normal activity in fibroblasts of alpha-neuraminidase activity. The responsible gene is SLC17A5 and most patients identified thus far harbor a missense mutation. The diagnosis of sialidosis can be suspected by identification of sialic acid–containing oligosaccharides and glycoproteins in urine, but it is made definitively by the demonstration of deficient alpha-neuraminidase activity and/or genetic sequencing. In a subtype of sialidosis, galactosialidosis, beta-galactosidase activity is also impaired, and the defect involves another lysosomal protein necessary for the activity of both enzymes (cathepsin A). Neuropathology of these disorders is characterized by neuronal storage of either free sialic acid in infantile sialic acid storage disease or sialic acid-containing oligosaccharides and glycoproteins in the sialidoses. Hypomyelination is also a prominent feature, and a SLC17A5 knockout mouse demonstrated an increased oligodendrocyte apoptosis and a decreased number of mature myelinating oligodendrocytes, which suggests that transporter function may be necessary for proper oligodendrocyte maturation. Prenatal diagnosis is made by the identification of elevated free sialic acid levels in cultured amniotic fluid cells in the infantile sialic acid storage disorder and of deficient alpha-neuraminidase activity in the sialidoses. DNA analysis is also possible for previously identified familial mutations. (Other disorders of glycoprotein degradation [i.e., mannosidosis and fucosidosis] have their onset beyond the neonatal period.)

Disorders Primarily Affecting White Matter
Many neurological disorders affect developing white matter (see Fig. 1 in van den Bosch et al. ) but are not degenerative disorders. The principal MRI differences between leukodystrophies and other white matter abnormalities are that the former usually exhibit prominent and confluent T2 hyperintensities on MRI. The discussion here is confined to progressive disorders that principally affect myelin.
Canavan Disease
Canavan disease , the spongy degeneration of the white matter, is an autosomal recessive disorder that commonly begins in the first days and weeks of life; approximately 20% of patients exhibit first signs at birth, an additional 10% in the first month, and a further 10% to 20% by the end of the second month. Clinical features documented in the first days and weeks of life include poor visual fixation, irritability, and poor suck (see Table 29.6 ). These features are followed over the ensuing weeks by marked hypotonia, weakness, nystagmus, and failure to attain motor milestones. Macrocephaly becomes apparent in the first 6 to 12 months of life in more than 50% of cases and ultimately in 90% of affected infants. As the disease progresses, hypotonia gives way to spasticity, decorticate posture, intellectual failure, optic atrophy, and then death in early to late childhood. Visual evoked responses are abnormal early in the disease, and these neurophysiological abnormalities precede the onset of blindness. Diagnosis is suspected by the clinical features. Although the computed tomography (CT) scan shows decreased attenuation of the white matter, MRI is preferable and shows a strikingly increased signal on the T2-weighted images ( Fig. 29.12 ). The particular involvement of subcortical white matter, especially early in the disease, distinguishes Canavan disease from Krabbe disease (see later discussion). Also characteristic is the involvement of the thalamus and especially the globus pallidus with relative sparing of the putamen (see Fig. 29.12 ). Elevation of N -acetylaspartate (NAA) levels in brain is detected by proton MR spectroscopy. Diagnosis is established by the demonstration of increased levels of NAA in urine and of decreased activity of N-acetylaspartoacylase (ASPA) activity in cultured fibroblasts . Elevated levels of NAA have been documented in Canavan disease early in infancy, in the urine of a mother with an affected 4-month fetus, and in the brain of 5- and 8-month-old fetuses. Deficient aspartoacylase activity has been detected in brain postmortems. NAA is normally present in high concentration in the neurons, whereas the acylase activity is predominantly localized in the oligodendrocyte. Studies in a mouse model of Canavan disease indicated that NAA is synthesized in the neurons and is transported to the oligodendrocytes, where the action of ASPA generates acetate critical for myelin lipid synthesis ( Fig. 29.13 ). Consistent with this notion is the demonstration that the developmental increase of ASPA in oligodendrocytes parallels cerebral myelination, and deficiency in ASPA impairs proper oligodendrocyte maturation. The hypothesis of acetate starvation is supported by observations that dietary acetate supplementation improves motor function and partially reverses myelin vacuolation in a rat model of Canavan disease. A second major hypothesis of oligodendroglial/myelin disease proposes that the accumulated NAA in oligodendrocytes acts as an osmolyte to lead to intramyelinic edema. Thus, studies in a newer Canavan disease mouse model, APSA nur7/nur7 (harboring a clinically relevant nonsense mutation in the ASPA gene), which also lacks the NAA synthase gene, suggest that NAA-derived acetate is not essential for myelin production. Complete ablation of NAA synthesis by deleting the NAA synthase Nat8L gene (see Fig. 29.13 ) does not disrupt normal myelination and is protective against leukodystrophy in the APSA nur7/nur7 model of Canavan disease, further adding to the NAA osmotic toxicity hypothesis. A second group of investigators using identical animals (deletion of Nat8L in APSA knockout mice) similarly demonstrated that the ablation of NAA production prevents myelin and axonal degeneration; however, they unexpectedly found that the survival time of the mice is not prolonged. Interestingly, they showed that the knockdown of Nat8L (using heterozygotes for the mutation) develops less severe pathology, behavioral improvements, and nearly normal survival time. Taken together, these recent mouse results add further support to the NAA toxicity hypothesis and suggest that partial inhibition of NAA synthase could be a therapeutic strategy with the development of CNS-permanent Nat8L inhibitors. The major neuropathological features are strikingly deficient in myelin and the widespread vacuolization (spongy degeneration) of white matter, especially in the subcortical regions.
DISEASE | CLINICAL FEATURES | METABOLIC-ENZYMATIC FEATURES | PATHOLOGY |
---|---|---|---|
Canavan disease (spongy degeneration of the white matter) | Macrocephaly with rapid head growth, poor visual fixation, hypotonia and later spasticity, developmental arrest, seizures and then regression, spasticity | N -acetylaspartic acid accumulates in brain and in urine and plasma; N -acetylaspartoacylase deficiency detectable in fibroblasts | Myelin deficiency, spongy vacuolation of white matter, especially subcortical, with involvement of globus pallidus and thalamus |
Alexander disease (leukodystrophy with diffuse Rosenthal fiber formation) | Macrocephaly with rapid head growth, developmental arrest, seizures, and then regression, spasticity | De novo, dominant gain-of-function mutation of GFAP | Myelin deficiency, Rosenthal fiber formation in fibrillary astrocytes, especially in subpial, perivascular, and subependymal loci |
Krabbe disease (globoid cell leukodystrophy) | Irritability, poor feeding, stimulus-sensitive tonic spasms, hypertonia, opisthotonos, developmental arrest and later regression, blindness, decerebration | Galactosylsphingosine accumulates in brain; galactosylceramidase I deficiency (a beta-galactosidase) | Myelin deficiency, multinucleated globoid cells (macrophages), and diminished oligodendroglia; peripheral neuropathy (segmental demyelination) |
Pelizaeus-Merzbacher disease | Abnormal eye movements ( nystagmus ), laryngeal stridor, head titubation, jerky movements, hypotonia (later spasticity), developmental arrest (later deterioration), seizures (2/3); X-linked recessive (PMLD; autosomal recessive) | Defect in gene (duplication, mutation or deletion) encoding myelin proteolipid protein (PMLD; defect in genes encoding connexin 47 and monocarboxylate transporter 8) | Severe myelin deficiency, usually total but occasionally with preserved islands of myelin; decreased number of mature oligodendrocytes; gliosis, occasional sudanophilic material |
Leukodystrophy with cerebral calcifications and cerebrospinal fluid pleocytosis (Aicardi-Goutieres syndrome) | Irritability, poor feeding, ocular abnormalities, hypertonia or hypotonia, weakness, jitteriness, dystonia, oral-facial dyskinesias, occasionally seizures, developmental arrest, microcephaly, and spasticity | Defect in TREX1 , gene encoding a DNA exonuclease, and at least six other genes involved in nucleic acid metabolism (see text) | Severe myelin deficiency, marked fibrillary gliosis, diffuse/focal calcifications, focal collections of inflammatory cells, microangiopathy |


More recent attempts at therapy have included virally and nonvirally mediated gene transfer methods and lithium (which causes a decrease in elevated brain NAA levels by unknown mechanisms). The initial findings are encouraging, but more data are needed. Long-term follow-up (up to 10 years) in 13 patients treated with an adeno-associated viral vector carrying the ASPA gene demonstrated reductions in elevated brain NAA levels, less brain atrophy, improvement in seizure frequency, and stabilization of clinical status. Newer viral constructs, some specifically targeting oligodendrocytes, show promise after single IV injections in animal models. A less complex approach may be acetate supplementation, in view of the importance of NAA as an acetate source in the oligodendrocyte/myelin unit, although the recent mouse studies discussed earlier cast some doubt on the utility of this approach. Enzyme replacement therapy has been hampered by blood-brain barrier and immunological limitations, but a modified version of ASPA appears to overcome these barriers in mice. Prenatal diagnosis has been made by the detection of elevated NAA levels in amniotic fluid and deficient ASPA activity in cultured amniotic fluid cells or chorionic villus samples. However, the most reliable method of diagnosis is DNA analysis.
Alexander Disease
Alexander disease may have its onset in the first weeks of life (≈30% of infantile cases), and the most frequent initial clinical features are macrocephaly, failure to attain early motor milestones and, interestingly, seizures (see Table 29.6 ). Progressive spastic quadriparesis, seizures, and failure of neurological development later become prominent, and death after a median survival of 3.5 years is most common. Diagnosis is suggested by the clinical features and brain imaging findings. CT shows decreased attenuation especially in frontal white matter, and after a contrast injection, the areas of increased attenuation (most notably subependymal) are noted early in the disease, particularly around the tips of the frontal horns. a
a References .
Cranial ultrasonography has been reported to show increased echogenicity of cerebral white matter early in the disease. MRI study is preferable and characteristic, showing an increased signal in the frontal white matter, with posterior extension, with abnormalities of the basal ganglia also, especially caudate heads and anterior putamina, and of the brain stem tegmentum and the periaqueductal regions ( Fig. 29.14 ). The brain stem changes coupled with the occasional finding of elevated lactate on MR spectroscopy may initially suggest Leigh disease. MRI changes can be evident even before the development of significant clinical symptoms. The major neuropathological features are a severe deficiency of myelin and eosinophilic deposits within the fibrillary astrocytes (Rosenthal fibers). These deposits have been shown by electron microscopy to be associated with an accumulation of disordered glial filaments. The Rosenthal fibers in Alexander disease are enriched in a protein—crystallin—which is characteristic of the eye lens, but is also synthesized in astrocytes. The crystallin molecules are ubiquitinated, and this modification appears to have altered their biophysical properties to result in insoluble aggregates of abnormally large ubiquitinated crystallin molecules. Interestingly, the TAR DNA binding protein of 43 kDa (TDP-43), a major pathological protein in the neurodegenerative diseases amyotrophic lateral sclerosis and frontotemporal lobar degeneration, becomes mislocalized to the cytoplasm of astrocytes in human and mouse Alexander disease brains. It is then pathologically phosphorylated and becomes increasingly insoluble, which is coincident with an increase in ubiquitinated proteins. The burden of phosphorylated TDP-43 is particular high in the youngest, most severely affected patients, suggesting that the pathophysiology of Rosenthal fibers may be similar to some adult neurodegenerative disorders. The fibers represent the intermediate filaments of astrocytes, which are composed of glial fibrillary acidic protein (GFAP). The molecular defect has been shown to be a de novo, dominant (i.e., heterozygous), missense mutation of the gene for GFAP. The latter results in a so-called toxic gain of function. Mutations continue to be identified (see http://www.waisman.wisc.edu/alexander-disease/ ) but nearly one-third involve two amino acids (R79 and R239). With the increasing diagnostic accuracy provided by GFAP sequencing, there is now the ability to correlate the age of onset, the genotype, and the clinical outcome. A recent analysis of 215 patients indicates that there are likely two classes of Alexander disease: type 1 with early onset, seizures, macrocephaly, encephalopathy, paroxysmal deterioration, failure to thrive, developmental delay, and classic radiologic features; type 2 with ages across the life span, autonomic dysfunction, bulbar symptoms, ocular movement abnormalities, palatal myoclonus, and atypical radiological features. Notably, cases with the R239 mutation had the most aggressive course. The finding of elevated levels of GFAP in the CSF and blood of affected infantile patients is consistent with the molecular defect and suggests potential value for diagnosis.
Exciting experimental studies have suggested two new avenues for potential treatment. A Drosophila model of Alexander disease has suggested that increased nitric oxide synthase (iNOS) in astrocytes leads to oxidative stress in glia and neurons, which could be blocked with oral pharmacological inhibitors of nitric oxide production. Importantly, similar upregulation in iNOS was observed in Alexander disease mouse models and patient brains. An in vivo pharmacological screen using the same model identified the upregulation of muscarinic cholinergic receptors that were contributing to GFAP toxicity, which was also observed in mouse models and postmortem patient tissue. Importantly, the muscarinic antagonist glycopyrrolate could block oxidative stress observed in the models and slightly reduce the number of seizures in the flies. Further validation of these drug candidates is necessary, but promising nonetheless.
Krabbe Disease
Krabbe disease , an autosomal recessive disorder , has its onset in the first weeks of life, before the median age of onset at 4 months of age, in approximately 25% of cases. The early clinical features are characteristic and consist of irritability, hypersensitivity to stimulation, startle responses, and increased tone (see Table 29.6 ). Poor feeding and unexplained, recurrent fever are common. Marked spasticity, severe stimulus-sensitive tonic extensor spasms, and decerebration then develop. Although macrocephaly has been reported to occur, small head size is more common. The CSF protein level is markedly elevated, which is consistent with the accompanying peripheral neuropathy, and may aid in the initial diagnosis (see Table 29.4 ). The neuropathy may be detected in the neonatal period by the measurement of nerve conduction velocities. As a result, deep tendon reflexes may be absent in advanced disease. A recent World-Wide Krabbe Registry has confirmed these features and the natural history of the disorder with a median survival of 25 months. Diagnosis is based on identification in white blood cells or fibroblasts of the enzymatic defect, which involves a beta-galactosidase, galactosylceramidase I (see Fig. 29.1 ). Neuroimaging findings, by CT, include increased attenuation in the thalamus relatively early in the disease. MRI is preferable and notable for showing particular involvement of cerebellar white matter and periventricular and central cerebral white matter, sparing subcortical myelin (in contrast to Canavan disease) ( Fig. 29.15 ). Abnormalities on diffusion tensor imaging correlate with the neurodevelopmental outcome and are important biomarkers for assessing the efficacy of the interventions.

Suzuki and co-workers clarified the basic biochemical nature of this disease ( Fig. 29.16 ). Because brain galactosylceramide (galactocerebroside) concentrations were found not to be elevated in Krabbe disease, it became apparent that the degradation of galactosylceramide is not the essential defect. However, psychosine (galactosylsphingosine) is elevated in the brain in Krabbe disease. Suzuki and co-workers showed that the enzyme missing in infantile Krabbe disease is galactosylceramidase I, which catalyzes the removal of galactose from galactosylsphingosine (psychosine) as well as from galactosylceramide (see Fig. 29.16 ). Psychosine is highly toxic to oligodendroglia, and injections of psychosine into the brain lead to the neuropathology of Krabbe disease. Galactosylceramidase II, which is not defective in Krabbe disease, can degrade galactosylceramide but not psychosine, thus explaining the failure of galactosylceramide concentrations to increase (see Fig. 29.16 ). The major neuropathological features include severe deficiencies of oligodendroglia and myelin, and collections of large, multinucleated globoid cells ( Fig. 29.17 ). These cells are essentially macrophages. The deficiency of galactosylceramidase has been demonstrated in the brain. Prenatal diagnosis is accomplished by assay of the enzyme in cultured amniotic fluid cells or chorionic villus samples. DNA analysis is also available if both pathogenic alleles in an affected family are known. Recent work has suggested a major advance in therapy of this disorder. Previous research showed that bone marrow transplantation is beneficial in the late-onset disease but not in the early-onset infantile disease. Because of the importance of very early initiation of therapy in the infantile disease, transplantation of umbilical cord blood was carried out after myeloablative chemotherapy in asymptomatic newborns ( n = 11; 12 to 44 days) and symptomatic infants ( n = 14; 142 to 352 days) with Krabbe disease. Donor cell engraftment and survival were 100% and 100%, respectively, in the asymptomatic newborns, and 100% but only 43% in the symptomatic newborns, respectively. The surviving infants had restoration of blood galactocerebrosidase levels. In the asymptomatic infants, over a median follow-up of 3 years, progressive cerebral myelination and normal cognitive development occurred, and mild to moderate motor disturbances were present. Children who underwent transplantation after the onset of symptoms had minimal neurological improvement. The findings indicate the importance of transplantation as early as possible and the value of umbilical cord blood . New York State now includes Krabbe as part of the newborn screen, and measurement of psychosine may make this more reliable and cost effective. However, the benefits and potential harms from false positives remain vigorously debated. Experimental studies that suggest a combination of bone marrow therapy with CNS-directed gene modalities and substrate reduction may have additive benefits that will likely inform future clinical trials.


Pelizaeus-Merzbacher Disease
One form of the three basic varieties of Pelizaeus-Merzbacher disease (PMD) typically has its onset in utero or during the first weeks of life. This so-called connatal form is distinguished from classic disease primarily on the basis of rate of progression (faster in the connatal cases), age at death (first decade in connatal cases vs. second decade or later in classic cases), and in severity of the neuropathology (total demyelination in connatal cases vs. partial demyelination with a tigroid appearance in the classic cases). Transitional cases are intermediate in severity. Because the molecular genetics and the clinical features of these three forms considerably overlap, distinction among them can be difficult, especially in infants with onset in the first weeks of life. At least 50 neonatal-onset, apparent connatal cases have been reported.
The most consistent initial clinical features have been nystagmus, horizontal or rotatory, and inspiratory stridor (see Table 29.6 ). Head titubation, jerky movements of the head or limbs, and hypotonia are prominent. Seizures occur in approximately 75% of patients with neonatal-onset cases, thereby suggesting a gray matter disorder and a confusing establishment of diagnosis. Visual evoked responses are usually abnormal, which is consistent with the disturbance of the central myelin. Diagnosis is suspected on clinical grounds. MRI shows a striking absence of myelin , with increased signal on T2-weighted images ( Fig. 29.18 ). CT may show decreased attenuation of cerebral white matter, but findings are not as consistently abnormal as with MRI. MR spectroscopy is distinctive; notable findings include the elevation of choline-containing compounds, NAA and N -acetylaspartyl glutamate, glutamine, and myoinositol. The findings are consistent, respectively, with reduced oligodendroglia with dysmyelination, increased axonal packing, and astrogliosis. Neuropathology is striking; myelin is virtually absent, with diminished oligodendroglia and gliosis ( Fig. 29.19 ). Small amounts of sudanophilic material, reflecting myelin breakdown products, may be present. X-linked recessive inheritance is consistent. The defect involves the gene on the X-chromosome encoding proteolipid protein (PLP) , a crucial structural protein of myelin accounting for 50% of myelin protein. PMD in all its forms is caused by mutations in PLP , with approximately 60% to 70% of cases related to duplications of the gene, 15% to 20% with point mutations, and the remainder being deletions. The neonatal-onset cases have been associated with duplications and deletions. The result of the former is multiple copies of PLP, and of the latter, deletion of both PLP and its post-transcriptional alternatively spliced protein product DM20. While the clinical phenotype of this disorder correlates with the degree of dysmyelination observed on MRI, specific mutations or size of duplications do not appear to impact the phenotype.


There are no therapies for PMD, but there are multiple areas of active investigation. A possible role for dietary cholesterol is suggested by recent studies in mouse models of PMD showing that increased dietary cholesterol prevented disease progression. The mechanism involved the promotion of normal PLP trafficking, and thereby oligodendrocyte differentiation and myelination. Preliminary data following implantation of neural stem cells in human patients with early onset disease suggest production of donor-derived myelin. Longer-term benefits are less clear, and the need for immunosuppression is a limitation. Umbilical cord blood transplantation after myeloablative chemotherapy performed in two young boys with PMD appears to have prevented disease progression. The two patients have been followed for 1 year and 7 years, respectively. Although initial results are encouraging, longer-term follow-up is needed.
A disorder phenotypically closely related to PMD, with a hypomyelinative leukoencephalopathy but autosomal recessive inheritance , has been termed Pelizaeus-Merzbacher-like disease (PMLD). Like connatal PMD, PMLD manifests with nystagmus during the newborn period. However, the subsequent course in PMLD differs from that of connatal, X-linked PMD (i.e., exhibits slower progression with a greater preservation of cognitive functions and the development of at least partial myelination of the corticospinal tracts by MRI). One molecular defect in PMLD involves the GJA12 gene, which encodes connexin 47, although mutations in this gene account for a minority of patients. The connexins are a family of proteins that form intercellular channels of gap junctions, between multiple cell types, including oligodendrocytes. Mutations in the monocarboxylase transporter 8 gene (MCT8) encoding a thyroid hormone transporter have also been identified in PMLD, suggesting that screening T3 and T4 concentrations may be useful in patients with the PMLD phenotype. The exact molecular mechanism of the transporter deficiency remains to be elucidated, but thyroid hormone is essential for proper oligodendrocyte differentiation, and hypothyroidism leads to motor and cognitive disabilities that are attributed to deficits in both gray and white matter.
Other rare sudanophilic hypomyelinative/dysmyelinative leukodystrophies may be related to PMD and perhaps may be secondary to defects of other structural proteins of myelin. The best established of these is Cockayne syndrome , an autosomal recessive disorder with the neuropathological finding of patchy tigroid demyelination, as in classic PMD. In the classic form, Cockayne syndrome type I, the development of postnatal growth failure, a cachectic , elfin , progeroid facial appearance, pigmentary retinopathy, impaired neurological development, sensorineural hearing loss, and cutaneous photosensitivity become apparent after the first months of life. In the less common severe form, Cockayne syndrome type II, newborns have already exhibited growth failure in utero, have markedly impaired postnatal somatic and head growth, and have poor to absent neurological development. Congenital cataracts and more rapid development of auditory and cutaneous complications are features of this severe form. The disturbance of myelination in Cockayne syndrome affects the peripheral nervous system as well as the CNS, and is manifested by elevated CSF protein levels, slow nerve conduction velocities, and nerve biopsy specimens showing segmental demyelination. In the CNS, in addition to the myelin disturbance, imaging studies show the calcification of basal ganglia, which is a helpful diagnostic feature. The basic biochemical disturbance in the disease involves DNA metabolism and ultrasensitivity to ultraviolet light, with abnormal DNA repair mechanisms detectable in cultured skin fibroblasts. Infants with the severe form of the disease usually die by the age of 6 or 7 years.
Leukodystrophy With Cerebral Calcifications (Aicardi-Goutieres Syndrome)
Leukodystrophy with cerebral calcifications , a rare autosomal recessive disorder initially clearly described by Aicardi and Goutieres, has been identified in approximately 100 cases.
Approximately one-third of patients have had the onset of the disease within the first postnatal month (see Table 29.5 ). Clinical features include irritability, poor feeding, ocular abnormalities (especially ocular jerking ), hypertonia more than hypotonia, weakness, jitteriness, dystonia, oral-facial dyskinesias, and, occasionally, seizures. Developmental arrest, microcephaly, and spastic quadriparesis follow, with death often in the first several years of life. Persistent CSF pleocytosis has been consistent (see Table 29.4 ). CSF protein levels are slightly elevated in fewer than 50% of patients. Elevated CSF levels of interferon-alpha also are a consistent finding. Brain imaging is distinctive. Ultrasonography shows echogenicity of periventricular white matter, a frequent finding in early infantile white matter degenerations. CT demonstrates decreased attenuation of cerebral white matter and calcifications, which may be diffuse, periventricular, or in basal ganglia ( Fig. 29.20 ). Characteristically, the calcification mostly affects the putamen and cerebellar dentate nuclei. MRI shows diffuse hyperintensity of cerebral white matter on T2-weighted images (see Fig. 29.20 ). Cerebellar atrophy is a common accompaniment. Severity of frontal and temporal lobe leukoencephalopathy correlates with the early age of onset and is associated with TREX1 mutations. Specific findings of temporal lobe swelling or temporal horn dilation, with progressive frontal lobe atrophy, and evidence of calcifications may also help differentiate infantile Aicardi-Goutieres syndrome from other leukodystrophies. Neuropathology consists of a marked decrease of myelin, striking fibrillary gliosis, and the calcifications, which are located primarily in the walls of the blood vessels. Perivascular collections of inflammatory cells have been noted, but no viral particles or other evidence of viral infection has been detected. The microangiopathy may be accompanied by thromboses and microinfarction. Large vessel arteriopathy has also been observed in patients with Aicardi-Goutieres syndrome caused by SAMHD1 mutations and may lead to stenosis, aneurysms, thrombosis, and intracerebral hemorrhage. This genetic subclass of patients (see later) may require unique intracranial arteriopathy screening and treatment. The elevated CSF levels of interferon-alpha raise the possibility that the cytokine is causally related to the cerebral disorder, and a recent case control study confirmed that six interferon-stimulated genes could differentiate cases from controls. Transgenic animals with astrocyte-targeted interferon-alpha showed neuropathological features mimicking those found in Aicardi-Goutieres syndrome. Further supporting a direct pathogenetic role of this cytokine, interferon-alpha can directly produce calcifying microangiopathy in smooth muscle cells in vitro. Some infants with the syndrome exhibit cutaneous vascular lesions similar to those observed in patients treated with interferon-alpha. Interferon-alpha treatment of young infants with vascular malformations has been associated with the development of spastic diplegia, a finding that suggests injury to developing white matter by the cytokine. Recent work shows mutations in Aicardi-Goutieres syndrome involving at least seven different genes, including TREX1, RNASEH2A, RNASHE2B, RNASEH2C, SAMHD1, ADAR, and IFIH1, involved in nucleic acid metabolism and signaling, which lead to an interferon-mediated innate immune response. Other proinflammatory cytokines and chemokines may have distinct patterns of abnormality and be useful biomarkers for diagnostic and therapeutic monitoring. A variety of systemic and brain-reactive autoantibodies also have been identified, further suggesting a likely autoimmune mechanism and supporting current studies of immunosuppressive and immunomodulatory therapies.

Disorders Affecting Both Gray and White Matter
Several disorders with neonatal onset exhibit prominent features of both gray matter and white matter disease ( Table 29.7 ; see also Table 29.1 ). Two of these affect subcellular structures present in all cells; that is, disorders of peroxisomes and of mitochondria. The peroxisomal disorders are represented in the newborn primarily by Zellweger (cerebrohepatorenal) syndrome and neonatal adrenoleukodystrophy , and the mitochondrial disorders in this context are represented primarily by Leigh syndrome (and related encephalopathies). (The other mitochondrial disorder with possible neonatal presentation, Alpers disease, manifests as a gray matter degeneration, as discussed earlier.) Another disorder in this broad category involves synthesis of an amino acid, serine, which is important in all cells, and includes prominent gray matter (e.g., seizures) and white matter (neuroimaging appearance of periventricular leukomalacia ) signs (see Table 29.7 ). Two disorders exhibit a strong regional predilection for cerebellar and brain stem structures; that is, congenital disorders of glycosylation and pontocerebellar hypoplasia (see Table 29.7 ). A regional predilection involving basal ganglia and related connections is apparent in neurotransmitter defects, which, in the newborn, are accompanied by both gray and white matter signs (see Table 29.7 ). Finally, Rett syndrome in males—a rare fulminating disorder with seizures and a variety of other neurological signs—involves a transcriptional mechanism present in both neuronal and glial cells (see Table 29.7 ).
DISEASE | CLINICAL FEATURES | METABOLIC-ENZYMATIC FEATURES | PATHOLOGY |
---|---|---|---|
Zellweger syndrome (cerebrohepatorenal syndrome) | Craniofacial dysmorphism, hypotonia, weakness, impaired feeding, seizures, optic atrophy, renal cysts, calcific stippling, later blindness, deafness, relative macrocephaly, moderately rapid progression to vegetative state | Evidence of deficiency of multiple peroxisomal functions: elevated very-long-chain fatty acids, pipecolic acid, and bile acid intermediate levels, deficient synthesis of plasmalogens; a disorder of peroxisomal biogenesis; molecular defect affecting proteins involved in peroxisomal biogenesis (peroxins), encoded by specific genes (PEX) , or rarely single enzyme defects (ACOX1, HSD17B4) | Severe neuronal migrational defects; major features include pachygyria (especially parasagittal region) and microgyria (especially lateral convexity), with heterotopias also involving the cerebellum and inferior olivary nuclei; myelin disturbance as described next for neonatal adrenoleukodystrophy, although less severe |
Neonatal adrenoleukodystrophy | Similar to Zellweger syndrome (above), but less severe, course is less rapid, and absence of renal cysts and calcific stippling | Similar to Zellweger syndrome (above), except findings are generally less severe; molecular defect affects proteins involved in peroxisomal biogenesis (peroxins) encoded by specific genes (PEX) | Myelin deficiency and loss, diffuse but especially marked in cerebellum; sudanophilic material, perivascular mononuclear cells, trilamellar inclusions in macrophages; neuronal migrational defects, although less prominent and consistent than in Zellweger syndrome |
Leigh syndrome | Hypotonia, weakness, respiratory abnormalities, impaired feeding, oculomotor abnormalities, facial weakness, seizures, developmental arrest with development later of movement disorders and regression | Elevated lactate-pyruvate in cerebrospinal fluid, variably in serum; deficiency in pyruvate dehydrogenase complex, cytochrome c oxidase (complex IV), reduced nicotinamide adenine dinucleotide-coenzyme Q reductase (complex I), or adenosine triphosphatase subunit 6 (complex V) | Focal, symmetrical, spongiform necrosis especially in diencephalon and brain stem, particularly surrounding third ventricle, aqueduct, and fourth ventricle, characterized by necrosis (relative sparing of neurons), demyelination, vascular proliferation, and astrocytosis |
Other mitochondrial encephalopathies | Various combinations of the above manifestations | Elevated lactate-pyruvate as for Leigh syndrome; enzymatic defects overlap (see text) | Cellular pathology similar to Leigh syndrome, but regions affected differ; may be principally cerebral white matter and various combinations of the regions involved in Leigh syndrome |
Serine synthesis deficiency | Congenital microcephaly, intrauterine growth restriction, refractory seizures, psychomotor delay | Global atrophy and enlarged ventricles | |
CDG type 1a (CDG1a): carbohydrate-deficient glycoprotein syndrome | Facial dysmorphisms, abnormal subcutaneous fat, abnormal eye movements, hypotonia, joint contractures, cardiac-liver-renal abnormalities; subsequent developmental arrest, seizures, ataxia, strokelike episodes | Abnormally glycosylated transferrin, coagulation factors, hormones, lysosomal enzymes, other glycoproteins; phosphomannomutase deficiency (CDG1a) | Cerebellar hypoplasia; often with pontine hypoplasia (i.e., pontocerebellar hypoplasia); neuronal loss and gliosis, especially in the cerebellum but also in the cerebral cortex, basal ganglia, thalamus, pons, inferior olives, and spinal cord; polyneuropathy |
Pontocerebellar hypoplasia type 2 | Impaired sucking and swallowing, jitteriness; later progressive microcephaly, extrapyramidal dyskinesias, epilepsy, severe mental retardation | Unknown | Neuronal loss and gliosis, especially in ventral pons and cerebellum, less so in inferior olivary nuclei; moderate cerebral cortical neuronal degeneration and atrophy |
Neurotransmitter Disorders | Encephalopathy, epilepsy, pyramidal and extrapyramidal motor features, autonomic changes, and later neuropsychiatric features |
Peroxisomal Disorders
Peroxisomal functions include both catabolic activities (e.g., beta-oxidation of very-long-chain fatty acids, degradation of hydrogen peroxide by catalase, pipecolic acid metabolism, oxidation of phytanic acid) and anabolic activities (e.g., biosynthesis of plasmalogens and of bile acids). Peroxisomal disorders are divided into two broad groups: disorders of peroxisomal assembly, in which there is a decrease in the number or structure of peroxisomes with multiple peroxisomal enzymes affected (group I); and disorders of single peroxisomal enzymes, with normal peroxisomal number and structure (group II) ( Table 29.8 ).
Group I: Disorders of peroxisomal assembly: activities of multiple peroxisomal enzymes deficient |
Zellweger cerebrohepatorenal syndrome |
Neonatal adrenoleukodystrophy |
Infantile Refsum disease |
Rhizomelic chondrodysplasia punctate |
Group II: Activity of only one peroxisomal enzyme deficient: peroxisomes normal in number and structure |
X-linked adrenoleukodystrophy |
D-bifunctional protein deficiency |
Acyl-coenzyme A oxidase deficiency |
beta-Ketothiolase deficiency |
Other |
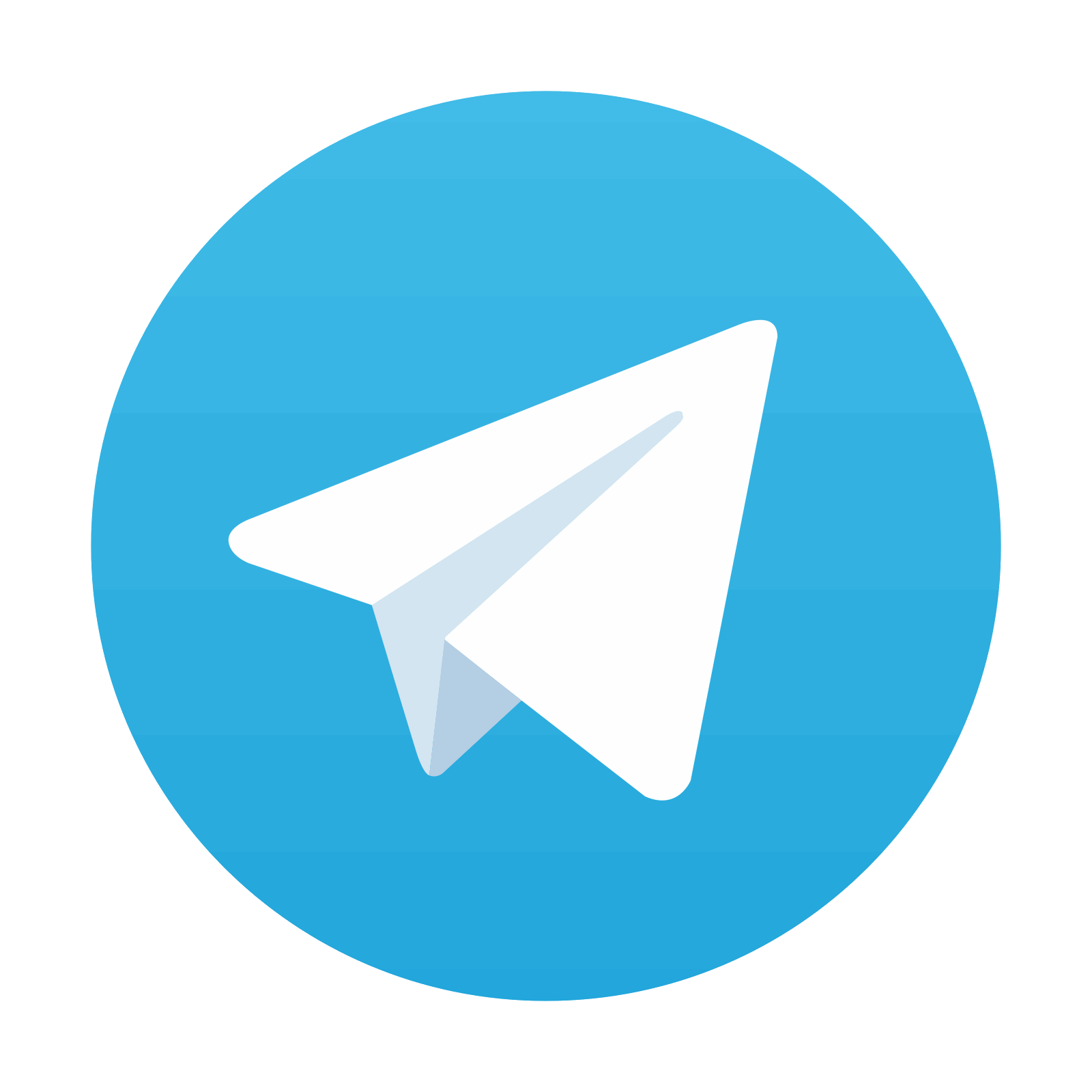
Stay updated, free articles. Join our Telegram channel

Full access? Get Clinical Tree
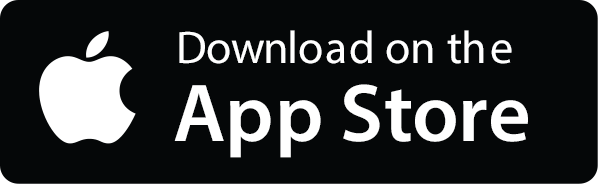
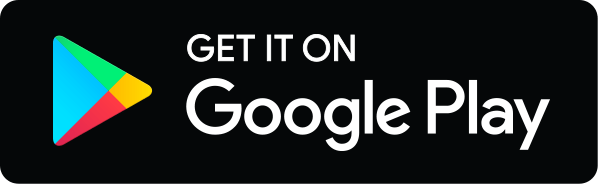
