html xmlns=”http://www.w3.org/1999/xhtml” xmlns:mml=”http://www.w3.org/1998/Math/MathML” xmlns:epub=”http://www.idpf.org/2007/ops”>
Introduction
By 2020, the World Health Organization (WHO) has predicted that unipolar depression will be second only to cardiovascular disease in the leading causes of total disease burden worldwide. Women have a higher lifetime prevalence of depression in high-, middle- and low-income countries (WHO, 2008), and this higher prevalence is due to high risk of first onset of depression and not to persistence or recurrence (Kessler, 2003). The higher prevalence is not limited to depression, as women have higher rates of other stress-related disorders (Weich et al., 2001). The higher rates of depression and anxiety in women have implications for future generations as maternal depression has negative effects on perinatal outcomes (Grigoriadis et al., 2013).
Stressful life events are strongly associated with depression and the vast majority of first episodes of depression are preceded by such events (Kendler and Gardner, 2010). There is conflicting evidence regarding gender differences in exposure to stressful life events; however, it is clear that women are at an increased risk of experiencing physical and sexual abuse during their lifetime, and may be more likely to become depressed following stressful life events (Maciejewski et al., 2001). The cause for this is multifactorial, but could partially be explained by sex differences in stress responsivity and reactivity of the hypothalamic-pituitary-adrenal axis (HPA) (Kudielka and Kirschbaum, 2005). Sex hormones can modulate the HPA axis, and the evidence that women have twice the rate of depression only after the onset of puberty (Chapter 7) with an equalization after menopause suggests that the female sex hormones contribute to the female preponderance in rates of depression.
The concept of “reproductive depression” has been proposed to account for the association between hormonal changes and mood during the female reproductive cycle. Clinically, reproductive depression is manifested as premenstrual depression, postnatal depression and perimenopausal depression (Studd and Nappi, 2012). Although not a subcategory of depression in current classification systems, a subsection of women may be vulnerable to this type of depression (Craig, 2013). See also Chapters 8, 9, 10.
Clearly the etiology underlying gender differences in depression is complex and not completely understood. Reduction to a single explanatory paradigm would be overly simplistic. Rather, an understanding of the modulating role of the female sex hormones and the interaction between genetic configuration and environmental influences is necessary to advance our understanding of the gender differences in depression.
In this chapter we discuss sex differences in the serotonergic system, the HPA axis, neuroimaging, reproductive depression and finally the medical implications of depression as they relate to women.
Sex differences in serotonergic neurotransmitters
The serotonergic system develops early in the course of embryogenesis and modulates the development of specific brain areas (Kinast et al., 2013). In animal studies it has been shown to be involved in the modulation of anxiety-like behavior, conditioned fear, stress responses and reward-related behaviors (Asan et al., 2013). The serotonergic system projects from the dorsal raphe nuclei in the brainstem to the forebrain. The modulation of the circuit that connects the dorsal raphe nucleus, the prefrontal cortex and the amygdala is of particular importance in mood disorders.
Serotonin is synthesized from L-tryptophan and the rate limiting step in the pathway is controlled by tryptophan hydroxylase (TPH), which converts tryptophan to 5-hydroxytryptophan. The monoamine oxidases (MAOs) breakdown serotonin into 5-hydroxyindoleacetic acid (5-HIAA), a step that is inhibited by the monoamine oxidase inhibitor (MAOI) class of antidepressants. There are 15 subtypes of serotonin (5-HT) receptors, and in addition to the central nervous system serotonin is produced in the gastrointestinal tract and in platelets. The serotonin transporter (SERT) reuptakes 5-HT from the synaptic cleft into the presynaptic neuron, and selective serotonin reuptake inhibitor (SSRI) antidepressants act by inhibiting the SERT, resulting in an increase in 5-HT in the extracellular space. The presynaptic 5-HT1A autoreceptors detect serotonin in the extracellular space and modulate the activity of the serotonin neuron (Celada et al., 2004).
Under normal physiological conditions, approximately 99% of tryptophan is metabolized to kynurenine in the liver by tryptophan 2,3-dioxygenase (TDO). However, proinflammatory cytokines such as interferon-γ, C-reactive protein, IL-1, IL-6 and TNF-α, can induce the enzyme indoleamine-2,3-dioxygenase (IDO), which is found in macrophages and microglia cells. IDO is the first and rate limiting step in the kynurenine pathway of tryptophan catabolism. Kynurenine is metabolized to hydroxykynurenine (3-HK), 3-hydroxyanthranilic acid (3-HAA) and quinolinic acid (QUIN), which are neurotoxic. Additionally, this process diverts tryptophan from the synthesis of serotonin, and could potentially result in decreased availability of serotonin. In the competing kynurenine pathway, kynurenine is metabolized to kynurenic acid, which blocks the neurotoxic effects of QUIN (Geng and Liu, 2013). The kynurenine pathway has important implications for neuropsychiatric disorders, including depression, as kynurenine can cross the blood brain barrier to increase central levels, and when metabolized could result in neurotoxic metabolites (Myint and Kim, 2013).
There is a polymorphism in the 5-HTT gene promoter region, which has been extensively studied for its links with neuropsychiatric disorders. A recent meta-analysis showed a small but significant association between 5-HTTLPR genotype and HPA-axis reactivity to acute psychosocial stress. The homozygous carriers of the short allele had increased cortisol reactivity compared to individuals with the s/l and l/l genotype, though there was no association with gender (Miller et al., 2013).
The sex steroids can alter serotonin (5HT) homeostasis (Sanders, 2012) and therefore have important implications for the regulation of mood. The primary female sex hormones (estradiol and progesterone) regulate the serotonergic system and affect serotonin transporter promotion region expression (Michopoulos et al., 2011). Estrogens can increase the expression and activity of tryptophan hydroxylase (TPH) gene expression, thereby increasing 5HT availability (Smith et al., 2004) and can modulate 5HT1A and 5HT2A receptors (Moses-Kolko et al., 2003). Positron emission tomography (PET) studies have shown differences in the binding potential of the 5HT1A receptor in the raphe nuclei in women with premenstrual dysphoric disorder compared to controls (Jovanovic et al., 2006). Ovarian hormones also regulate serotonin reuptake transporter gene (SERT) protein expression (Lu et al., 2003) and modulate gene expression of adhesion molecules in serotonin neurons that are important for synapse assembly (Bethea and Reddy, 2012).
Estrogen can decrease MAO A gene expression, which is the primary enzyme involved in serotonin breakdown (Gundlah et al., 2002). A PET study (N=15) investigated the link between reduced levels of estrogen in the postpartum period and monoamine oxidase A levels in the prefrontal cortex, anterior cingulate cortex, anterior temporal cortex, thalamus, dorsal putamen, hippocampus and midbrain. MAO A levels were significantly elevated, which indicated a monoamine lowering effect was occurring postpartum (Sacher et al., 2010).
Sex differences in neuroendocrine system
Stress can be physical or psychological and has been defined as a state where homeostasis is threatened or perceived to be threatened (Bradley and Dinan, 2010). The hypothalamic pituitary adrenal axis (HPA) is the core endocrine stress system, and when the brain perceives a threat, the HPA in conjunction with the sympathetic adrenal medullary (SAM) axis is activated. The paraventricular nucleus (PVN) in the hypothalamus regulates the neuroendocrine response whereas the amygdala regulates the majority of the autonomic and behavioral stress reactions in the brain (Kovacs, 2013). At the molecular level corticotropin releasing hormone (CRH) and arginine vasopressin (AVP) regulate HPA activity, both of which are synthesized in the PVN. These hormones act on the anterior pituitary gland and cause the release of adrenocorticotropic hormone (ACTH). ACTH stimulates the release of glucocorticoids (cortisol) from the adrenal cortex, which bind with specific intracellular receptors called the mineralocorticoid and glucocorticoid receptors, which are located in multiple tissues in the body. This system works as a negative feedback loop, whereby glucocorticoids modulate their own secretion by acting at various levels of the HPA axis, such as the hypothalamus and pituitary.
The HPA axis has been extensively studied and there is robust evidence that dysfunction of the HPA axis is associated with stress-related disorders including depression (Stetler and Miller, 2011). Indeed, this HPA axis dysfunction may extend beyond the mood disorders into psychotic disorders, and a recent finding from the North American longitudinal study (N=256) showed higher cortisol levels in subjects who meet clinical high risk (CHR) criteria for psychosis (Walker et al., 2013).
Animal studies have shown that females have higher glucocorticoid levels after HPA axis stimulation (Yoshimura et al., 2003), but in humans the effect is inconsistent. Overall the evidence suggests that there are higher cortisol responses in young men than in young women after exposure to acute real-life psychological stress whereas females tend to display an enhanced stress response to pharmacological challenge tests (Kudielka and Kirschbaum, 2005). This appears contradictory given the higher rates of stress-related disorders in women, but there are several hypothetical reasons for the differences.
The discordance may be attributable to the different pathways that lead to the activation of the paraventricular nucleus (PVN) in the hypothalamus. Psychological stress activates the PVN via the prefrontal cortex, hippocampus and amygdala (limbic circuit), whereas physiological and pharmacological stress may bypass the limbic circuit to activate the PVN directly (Herman and Cullinan, 1997). Interestingly the sex differences in the HPA axis reactivity persist even under induced hypogonadal conditions (Roca et al., 2005).
It is postulated that different types of psychological stress affect women and men differently. It has been shown that women have a greater cortisol response to rejection stress (Stroud et al., 2002), whereas men react more to achievement challenges. The Trier Social Stress Test (TSST) is a 15-minute psychosocial stress protocol involving 5 minutes of anticipatory stress, 5 minutes of public speaking and 5 minutes of mental arithmetic performed in front of a panel of evaluators. It therefore contains elements of uncontrollability and social evaluative threat and it elicits a greater ACTH and free cortisol response in men compared to women (Allen et al., 2013). Marin et al. (2012) randomly assigned 30 women and 30 men to either real neutral news or real negative news excerpts for 10 minutes. Then all subjects were exposed to the TSST. Only in women did the reading of negative news result in a significant increase in salivary cortisol levels in the TSST. One day later a free recall of the news was performed and women were better able to remember the negative news excerpts compared to men (Marin et al., 2012).
Early traumatic events, such as sexual or physical abuse increase the risk of psychiatric disorders in later life (Dube et al., 2001, Banyard et al., 2001, Faravelli et al., 2012). Childhood sexual abuse is more common in girls (Stoltenborgh et al., 2011). These traumatic early life events can adversely affect the development of the brain and HPA axis. Although not the only factor involved, dysregulation of the HPA axis associated with childhood trauma is an important mechanism underlying the increased rate of depression experienced by women who have been abused during childhood (Weiss et al., 1999). Women who experience childhood abuse may be more susceptible to alterations in HPA axis reactivity, and this may have implications for the increased vulnerability to depression in later life (DeSantis et al., 2011, Heim et al., 2000, Bremner et al., 2003). Traumatic events in adulthood do not have the same impact on the HPA axis, indicating that traumatic events during the period of brain development may result in persistent changes in the reactivity of the HPA axis (Klaassens, 2010, Klaassens et al., 2009). Furthermore, the persistent alteration in the HPA axis reactivity of women with a history of childhood sexual abuse can have implications for their offspring. Infants born to mothers with a history of childhood abuse have altered HPA reactivity in the postpartum period (Brand et al., 2010). Early life trauma can therefore be transferred to the next generation via HPA axis reactivity and thus highlights the profound negative effect of childhood trauma on the future development of stress-related disorders.
In addition to HPA axis dysregulation, studies show brain changes in women with a history of childhood abuse. The most consistent finding in this group of women is of smaller hippocampal volumes (Stein et al., 1997, Vythilingam et al., 2002, Rao et al., 2010), although other brain changes have also been demonstrated. A recent study found decreased gray matter volumes in the right middle cingulate gyrus in healthy young adults with a history of childhood trauma, and this was associated with increased levels of awakening cortisol (Lu et al., 2013).
HPA axis dysfunction has been linked to other stress-related disorders. The functional somatic disorders (FSDs), which compromise chronic fatigue syndrome, fibromyalgia and irritable bowel syndrome (IBS), are more common in women (Lovell and Ford, 2012). IBS is characterized by an overactivity of the HPA axis and an increase in proinflammatory cytokines (Dinan et al., 2006) and it is well established that these disorders are associated with higher rates of depression and anxiety (Henningsen et al., 2003). Recent evidence suggests that cognitive performance is also affected in IBS patients (Kennedy et al., 2013). However, a recent meta-analysis of 85 studies investigating HPA axis activity and functional somatic disorders reported that there was insufficient evidence to suggest that IBS was a hypocortisolemic disorder, but did find a significant reduction in basal cortisol in females with fibromyalgia (Tak et al., 2011).
Sex differences in neuroimaging
The Human Connectome Project (HCP) is a major project that aims to construct a map of the complete structural and functional neural connections in vivo within and across individuals (Van Essen and Ugurbil, 2012). This will certainly add to the evidence that there are significant differences in the structure and functioning of female and male brains and more specifically in those brain regions that control emotion.
Sex differences in functional magnetic resonance imaging (fMRI), diffusion tensor imaging (DTI) and positron emission tomography (PET) studies have recently been reviewed (Sacher et al., 2013). In structural MRI studies, women have smaller total brain volumes (Cosgrove et al., 2007), a higher ratio of grey to white matter thickness (Luders et al., 2005) and increased cortical depth (Im et al., 2006). They have increased grey matter volume in dorsal anterior, posterior and ventral cingulated cortices and in the right inferior parietal lobe (Chen et al., 2007) with greater regional density and volume in dorsal and ventral stream regions (Keller and Menon, 2009).
It is hypothesized that sex steroids may account for the sex differences in grey matter volumes (Witte et al., 2010). In addition to chromosomal makeup, sex steroids are involved in sex differentiation in early development (Tokarz et al., 2013) and have an organizational role in the structure and function of the developing human brain (Peper et al., 2009).
Diffusion tensor imaging has shown gender differences in white matter connectivity between many brain regions, including the thalamus, cingulum, corpus callosum (Menzler et al., 2011), frontooccipital fasciculus, parahippocampal gyrus, bilateral internal capsule, medial frontal gyrus, hippocampus, insula, post central gyrus, frontal and temporal lobes (Sacher et al., 2013). A diffusion tensor imaging study of 8–22-year-old males and females (N=949) demonstrated sex specific differences in connectivity during the course of development. Male brains are structured to facilitate connectivity between perception and coordinated action, and female brains are structured to facilitate communication between analytical and intuitive processing (Ingalhalikar et al., 2013).
In MRI resting state functional studies there are hemisphere-related differences (Tian et al., 2011) and differences within the dorsolateral prefrontal cortex, the amygdala (Zuo et al., 2010) and periaqueductal grey (Kong et al., 2010) areas, which are involved in mood and emotion regulation.
Most PET studies show a higher rate of global glucose utilization in females, particularly in the orbital-frontal area (Andreason et al., 1994). This can change with different phases of the menstrual cycle (Reiman et al., 1996). PET studies investigating gender differences in the serotonergic and dopaminergic systems are inconsistent (Sacher et al., 2013) so it is difficult to draw firm conclusions.
A meta-analysis demonstrated that women have greater activation than men in the left amygdala, left thalamus, hypothalamus, mammillary bodies, left caudate and medial prefrontal cortex in response to negative emotions. This valence specificity was most marked for the amygdala (Stevens and Hamann, 2012). This is in keeping with earlier studies showing that women experience the remembering of emotional events in more detail and with greater intensity than men (Seidlitz and Diener, 1998). Yuan et al. (2009) compared event-related potentials in men and women in response to images of different emotional valence (rated high negative, moderately negative or neutral) and found that females are more susceptible to negative stimuli of lesser salience and this susceptibility was not seen for positive stimuli.
In an fMRI study using a working memory task and an emotional distraction, women had increased sensitivity to emotional distraction in brain areas associated with “hot” emotional processing, whereas men showed increased sensitivity in areas associated with “cold” executive processing. There was a sex-related dorsal-ventral hemispheric dissociation in the lateral prefrontal cortex (PFC) related to coping with emotional distraction, with women showing a positive correlation with working memory performance in the left ventral PFC and men showing similar effects in the right dorsal PFC (Iordan et al., 2013).
In a recent study by Holsen et al. (2013), 15 women with recurrent major depressive disorder (rMDD) and 15 healthy controls were MRI scanned during neutral and negative stimuli. Women with rMDD demonstrated higher anxiety ratings, increased cortisol levels and hyperactivation in the amygdala and hippocampus. Amygdala activation was negatively related to cortisol changes and positively associated with the duration of remission.
Reproductive depression
Prepubescent boys have slightly higher rates of depression than prepubescent girls (Twenge and Nolen-Hoeksema, 2002). This pattern reverses during mid-puberty and rates of depression remain higher in women until after menopause (Cyranowski et al., 2000). Pubertal changes in sex hormones contribute to this divergence in mid-puberty, as they modulate the HPA and may sensitize girls to stressful life events and therefore potentially result in higher rates of depression during this period (Young and Altemus, 2004). That stress responses are influenced by female sex steroids is now beyond doubt.
A recent study using the Avon Longitudinal study of parents and children cohort in the United Kingdom demonstrated that early menarche is associated with an increase in depressive symptoms in early to mid-adolescence (Joinson et al., 2013). Indeed the menstrual cycle continues to be problematic for a subsection of women who experience premenstrual syndrome (PMS). This includes a wide variety of physical, psychological and cognitive symptoms that occur recurrently and cyclically during the luteal phase of the menstrual cycle and disappear soon after the onset of menstruation (Allais et al., 2012). Up to 20% of women with premenstrual symptoms are severe enough to require treatment (Halbreich, 2004).
At the cognitive level there are changes in the integration of emotional and cognitive processing in women with PMS. A recent pilot study demonstrated an increase in the mean reaction time for resolving emotional conflict from the follicular to the luteal cycle phase in all women, but only women with PMS showed an increase in physiological (salivary cortisol) and subjective stress measures during the luteal phase (Hoyer et al., 2013). Salivary cortisol is an easily accessible and reliable measure of HPA activity.
Five percent of women of reproductive age will experience a severe form of PMS called premenstrual dysphoric disorder (PMDD) (Brown et al., 2009), which has a heritability of approximately 50% (Kendler et al., 1998). Although symptoms are not associated with defined concentrations of any specific sex or nonsex hormone (Rapkin and Akopians, 2012), hormonal changes during the late luteal phase of the menstrual cycle are thought to contribute to this disorder. Interestingly these symptoms are effectively eliminated by ovarectomy or by treatment with a gonadotropin releasing hormone antagonist (Eriksson et al., 2012) – again supporting a link between female sex steroids and depression.
The underlying pathophysiology of PMS, although not fully clear, is thought to be linked to the interaction between the sex hormones and the GABAergic and serotonergic systems with involvement of the HPA. Progesterone is produced in the ovaries in the corpus luteum and its metabolites 3α,5α-tetrahydrodeoxycorticosterone (THDOC) and 3α-hydroxy-5α-pregnane-20-one (allopregnanolone) are neuroactive steroids and modulators of GABA-A receptors (Zorumski and Mennerick, 2013). Allopregnanolone can augment the function of GABA-A and has similar effects to benzodiazepines, barbiturates and alcohol (Akk et al., 2007, Paul and Purdy, 1992), substances known to induce mood effects at low dosages in humans and animals. In addition to GABAergic modulation, certain neurosteroids modulate glutamate receptors particularly N-methyl-D-aspartate receptors (Zorumski et al., 2013). Although meta-analysis has shown that progesterone is not an effective treatment for PMS (Ford et al., 2012), its derivatives acting as GABAergic and glutamatergic modulators may have potential as future treatments in certain subtypes of mood disorders.
In addition to modulating GABA and glutamate NMDA receptors, sex hormones interact with the serotonergic system and may contribute to altering mood during the menstrual cycle. Indeed the lowering of serotonin can give rise to PMS-like symptoms (Rapkin and Akopians, 2012) and meta-analysis has demonstrated that SSRIs are effective in the treatment of PMS if taken only in the luteal phase or continuously throughout the cycle (Marjoribanks et al., 2013).
The menstrual cycle is associated with inflammatory changes (Berbic and Fraser, 2013). Innate immune inflammatory responses are associated with depression, with most consistent findings for elevations in the cytokines interleukin-6 (IL-6), tumor necrosis factor-a (TNF-a), and C-reactive protein in those diagnosed with depression (Dowlati et al., 2010). Cytokines can activate HPA and induce the production of CRH, ACTH and cortisol (Pariante and Miller, 2001). Increased levels of cytokines in the periphery can affect the brain, via permeable or leaky blood brain barrier, activation of vagal fibers or active transport by cytokine specific transporters (Miller et al., 2009). One part of the innate immune system is comprised of pattern recognition receptors (PRR), a subtype of which are the Toll like receptors (TLRs) (McCusker and Kelley, 2013). Activation of the TLRs can result in the production and release of cytokines. A study investigating the TLRs during the menstrual cycle demonstrated that the responsivity of these receptors change during the course of the menstrual cycle (Dennison et al., 2012). Although increased levels of cytokines are found in certain subgroups of depressed patients, the implications of inflammatory changes during the menstrual cycle for mood are not fully elucidated.
The next stage in the female reproductive cycle is the perinatal period (see also Chapter 10). Mild depressive symptoms are common in the postpartum period, with up to 50–80% of women experiencing such symptoms in the early postpartum period (Workman et al., 2012). There is an increased risk of depression and psychiatric admission during the postpartum period (Munk-Olsen et al., 2006) with up to 20% of women meeting the criteria for a depressive episode in the first postpartum year (Wisner et al., 2013) with most of the episodes occurring in the first 3 months postpartum (Gavin et al., 2005).
For those women with a history of mood disorders, the perinatal period is a time of significant relapse risk. The risk of having a major affective episode if there is a history of recurrent major depression is approximately 40% and in those women with a history of bipolar disorder it is 50% (Di Florio et al., 2013).
There are considerable changes in biological parameters during the postpartum period, particularly in the neuroendocrine system. During delivery estriol, progesterone and CRH levels decrease rapidly, with modest decreases in cortisol. It has been shown that depressive symptom scores positively correlate with ACTH and negatively correlate with estriol and CRH levels (O’Keane et al., 2011). Although depressive symptoms may be associated with neuroendocrine changes, estrogen replacement alone is not effective in the treatment of postpartum depression (Craig, 2013) highlighting the multifactorial nature of this condition.
Due to the high risk of affective disorders during the perinatal period, thorough screening of women with a particular emphasis on monitoring those women with a history of mood disorders is necessary. The optimal management of mood disorders during pregnancy and the postpartum period has even greater consequence, as it may affect mother-child bonding with resultant negative effects on brain development in the offspring (Chapter 11). Preclinical studies highlight the detrimental impact of these early events and explore potential mechanisms. Mice subjected to chronic and unpredictable maternal separation in the postpartum period show depressive-like behaviors as adults. Epigenetic alterations in separated mice affect the germline, which has implications for the transgenerational transfer of stress-related disorders (Franklin et al., 2010). Furthermore, recent evidence shows that maternal care can result in alterations in the epigenetic regulation of hormone receptor levels (Pena et al., 2013). Preclinical and clinical studies indicate that the provision of adequate maternal care during the critical postpartum period is necessary for the normal development of brain circuits involved in emotion regulation.
The perimenopausal period is marked by a decline in ovarian function with associated fluctuations in estrogen levels (Wharton et al., 2012) and changes in the estrogen–androgen balance (McConnell et al., 2012). Clinically this stage consists of psychological, psychosomatic, sexual and vasomotor symptoms, and it is well established that perimenopausal women are at an increased risk of developing a depressive disorder and are more vulnerable to cognitive decline compared to premenopausal women (Weber et al., 2013). Interestingly, in addition to the SSRI’s effectiveness in treating perimenopausal depression, a recent meta-analysis of 11 randomized controlled trials showed that SSRIs also result in a modest improvement in vasomotor symptoms (Shams et al., 2013), which can affect up to 39% of perimenopausal women (Grigoriou et al., 2013).
The sex differences in depression equalize in the elderly population (Chapter 20). However, there may be higher rates of anxiety in elderly females (Vadla et al., 2013). In a recent cross-sectional analysis of survivors in the population-based Faenza study that included 359 subjects aged 74 years or older, an overall prevalence of depression of 25.1% was found with no evidence of gender differences (Forlani et al., 2013). Like postpartum depression, studies have shown estradiol to be ineffective in the treatment of depression in the postmenopausal stage (Morrison et al., 2004). Although hormone replacement is not an effective therapeutic agent in the treatment of depression, it is clear that female sex hormones play an important role in mood regulation. They accomplish this by modulating the HPA and the neurotransmitter systems involved in mood.
Interestingly, in contrast to the biological theories for the epidemiological sex differences in rates of depression, it has been hypothesized that women and men experience depression differently (Addis, 2008). Men are more likely to react with anger or self-destructive behavior, and engage in substance abuse with lower levels of impulse control compared to women (Winkler et al., 2005). In a recently published secondary analysis of the National Comorbidity Survey Replication, men reported higher rates of anger, aggression, substance use and risk taking compared to women, and when this is taken into account men and women met criteria for depression in equal proportions: 30.6% of men and 33.3% of women (Martin et al., 2013). Although an interesting prospect, further research would be required to validate these findings.
Implications of depression in women
Depression has a detrimental impact on quality of life and ability to function, but is also associated with a number of medical comorbidities. Cardiovascular disease (CVD) is the leading cause of mortality in women, and although rates of CVD have decreased in men in recent decades, similar reductions in women have not occurred. Men develop heart disease earlier than women, though the rates in women exceed those in men by the mid-40s (Go et al., 2013). Although the cardiac risk factors for men and women are the same, sex hormone changes during menopause and their impact on hypertension, lipid concentrations and central adiposity may contribute to the increased risk of cardiovascular disease in women during this period (Banos et al., 2011).
Depression is an independent risk factor for cardiac morbidity and mortality (Versteeg et al., 2013, Larsen et al., 2013), and the prevalence of depression in cardiac disease is 15–20% (Celano and Huffman, 2011). The relationship between cardiac disease and depression is bidirectional, as depression can increase the risk of cardiovascular disease and cardiovascular disease can increase the risk of depression (Plante, 2005). The etiology is multifactorial and involves the interaction of the autonomic nervous system, the neuroimmune, the neuroendocrine and the vascular systems. Depression is associated with overactivity of the HPA and SAM axes, both of which can result in vascular endothelial cell damage (Joynt et al., 2003). Inflammation is the principal pathophysiological process that occurs in cardiovascular disease, and oxidative stress and neuroinflammation have been linked to neurovascular dysfunction in depression (Najjar et al., 2013). Chronic stress can result in a pro-inflammatory state, and the increase of pro-inflammatory cytokines can result in platelet aggregation and contribute to atherosclerosis (Elsenberg et al., 2013). As mentioned earlier, cytokines can decrease the availability of serotonin postsynaptically, and enhance IDO activity resulting in decreased levels of tryptophan and potentially lead to serotonin deficiency (Halaris, 2013).
Several large trials have investigated the role of SSRIs in the treatment of depression in cardiovascular disease. The Sertraline treatment of major depression in patients with acute myocardial infarction or unstable angina (SADHART) demonstrated that sertraline was a safe and effective in the treatment of depression in CVD (Glassman et al., 2002, Glassman et al., 2009). The Enhancing Recovery in Coronary Heart Disease (ENRICHD) trial showed that there was an association with a lower risk of cardiac morbidity and mortality in the subgroup of patients taking SSRI antidepressants, though the effect was found in men only (Taylor et al., 2005, Jaffe et al., 2006). The Canadian Cardiac Randomized Evaluation of Antidepressant Psychotherapy Efficacy (CREATE) trial showed that citalopram was effective in the treatment of depression in CVD, and found that the addition of interpersonal therapy did not improve outcomes further (Lesperance et al., 2007). The Women’s Ischemia Syndrome Evaluation (WISE) study investigated subcategories of depressive symptoms in women with suspected myocardial ischemia as predictors of adverse cardiac outcomes, and found that somatic symptoms but not cognitive or affective symptoms predicted worse outcomes (Linke et al., 2009).
Although a direct causal link between depression and CVD has not been established, screening for depression in women with CVD is important, as depression should be viewed as an additional modifiable risk factor for the prevention of adverse cardiac events in women of all ages, but particularly in the perimenopausal stage.
An additional medical comorbidity associated with depression of particular importance in women is osteoporosis. Female gender, advancing age, estrogen and calcium deficiencies, limited physical activity, glucocorticoid treatment and smoking are established risk factors for this disorder. An estimated 22 million women in Europe have osteoporosis, which is 3 times higher than the rate in men, and is a major risk for fractures (Svedbom et al., 2013). A meta-analysis of 23 studies involving 2,327 depressed patients and 21,141 nondepressed subjects, showed that women that were diagnosed as depressed by a psychiatrist using DSM criteria had significantly lower bone mineral densities (BMD) (Yirmiya and Bab, 2009).
Recent evidence has raised concerns regarding antidepressant use and associated bone loss and fractures (Rizzoli et al., 2012). A meta-analysis of 12 observational studies showed a higher risk of fractures in those using SSRI medication (Eom et al., 2012), and another study showed an increase in fractures in elderly women using SSRIs (Diem et al., 2011). A recent study investigating a 12-week course of the serotonin and noradrenaline reuptake inhibitor venlafaxine in an elderly group of depressed patients found an increase in serum C-terminal cross linking telopeptide of type I collagen (ß-CTX), (used as a marker of bone resorption) in the venlafaxine group. However, the increase in ß-CTX was only significant in patients whose depression did not remit, suggesting attribution to venlafaxine is overly simplistic (Shea et al., 2013). Indeed, in a multicenter prospective cohort trial involving 1,972 middle-age women, antidepressants were not associated with an increased rate of bone loss, suggesting the risk is age dependent (Diem et al., 2013).
The interaction between depression, serotonin and bone metabolism is complex. Depression can result in lower bone mineral densities and fractures, and SSRIs can result in similar effects, particularly in elderly women. High-risk groups may require BMD screening and appropriate treatment to reduce the risk of fractures. Treating psychiatrists should take these factors into account when managing depression in this subgroup of women.
Conclusion
It is established that women experience more depression than men. However, the precise reasons for this are not fully understood. The interaction between genes and the environment is further influenced by the female sex hormones, which impact on the development of the brain and result in structural and functional brain differences as demonstrated by neuroimaging. The sex hormones modulate neurotransmitters and the HPA axis, which play important roles in emotional regulation. Fluctuations in the sex hormones during the female reproductive cycle and the related mood and cognitive changes can result in reproductive depression in a certain subgroup of women. Depression has medical implications for women as it is associated with an increased risk of comorbidities such as cardiovascular disease and osteoporosis. Indeed, the detrimental effects of depression in women are not limited to the individual as maternal depression in the perinatal period has important implications for the development of stress-related disorders in the next generation. Clinicians should take these important factors into account when managing depression in women.
References
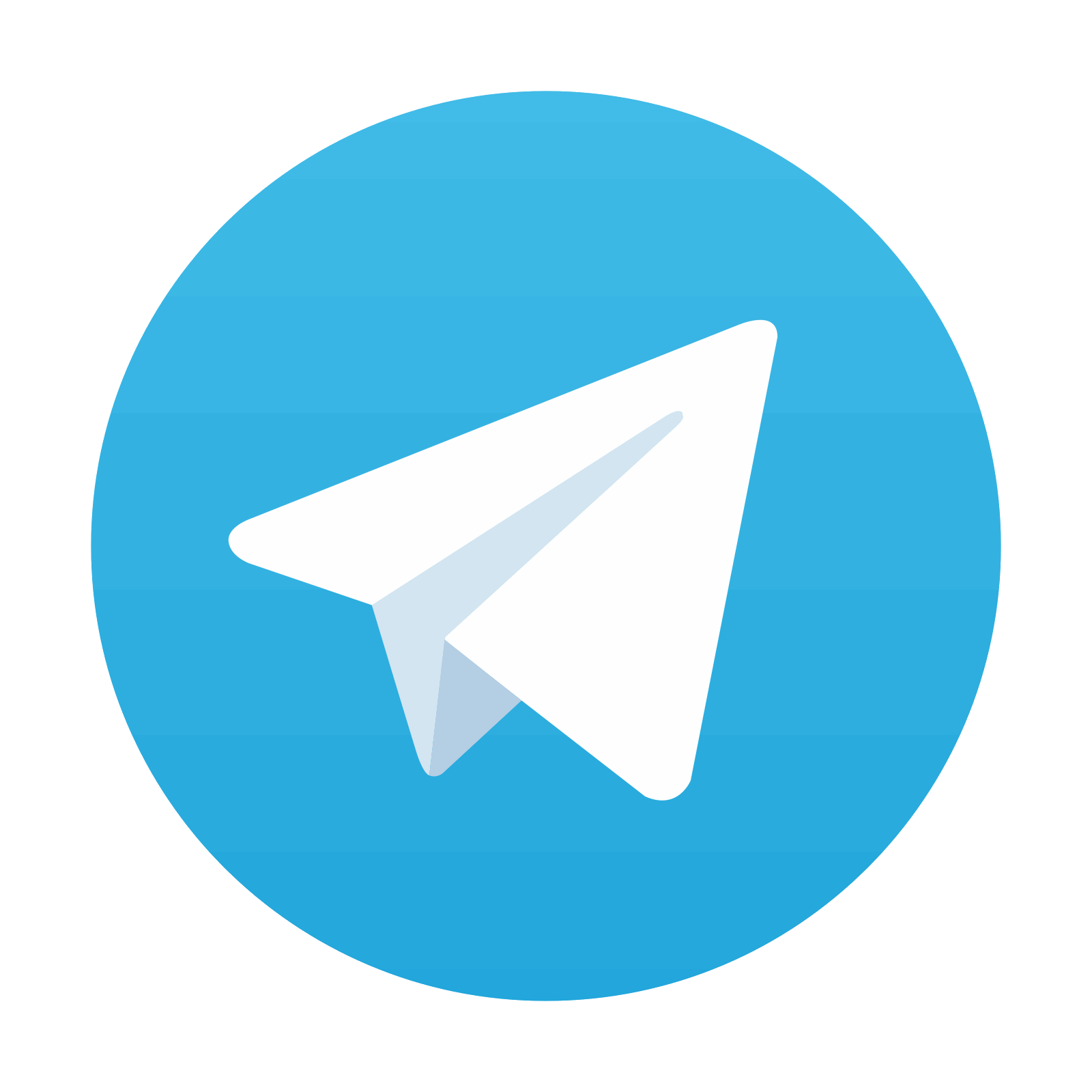
Stay updated, free articles. Join our Telegram channel

Full access? Get Clinical Tree
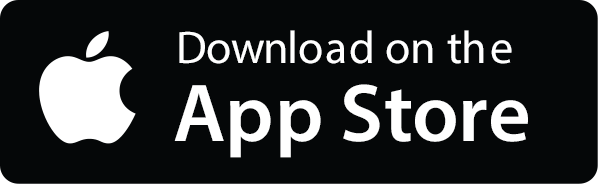
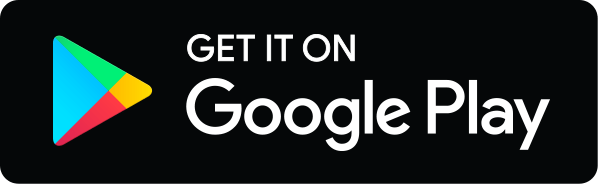