Fig. 11.1
Summary of main steps in the tryptophan–kynurenine pathway. In depression, the neurodegenerative pathway, leading to the synthesis of the neurotoxins quinolinic and picolinic acids, is increased while the neuroprotective pathway, in which kynurenic acid is the main end product, is decreased. The synthesis of nicotinamide adenine dinucleotide (NAD+) from quinolinic acid is an important co-factor for the electron transport system in mitochondria. Xantheuric and 3-hydroxyanthranilic acid are sources of reactive oxygen species which contribute to insulin insensitivity and further compromise energy metabolism (see Myint and Kim 2014; Oxenkrug 2013). IDO indoleamine 2,3 dioxygenase, TDO tryptophan dioxygenase, KAT kynurenine aminotransferase, KMO kynurenine monooxygenase, ICD isocitric dehydrogenase
The Tryptophan–Kynurenine Pathway and Deficits in Intermediary Metabolism
In recent years, attention has centred on the neurotoxic consequences of the increase in quinolinic acid and the intermediates formed from kynurenine in the tryptophan–kynurenine pathway. While such neurotoxins undoubtedly play a critical role in the neurodegenerative changes associated with chronic psychiatric disorders such as depression and schizophrenia, it is often overlooked that quinolinic acid is also an important substrate for the formation of nicotinamide adenine dinucleotide (NAD+). As NAD+ is a key component of the respiratory chain, chronic pathological changes that reduce its formation are liable to have adverse consequences for intermediary metabolism particularly in neurons that are critically dependent on high energy sources. It is estimated that approximately 99 % of tryptophan that is not used for protein and serotonin synthesis is metabolised to NAD+ via the tryptophan–kynurenine pathway, and therefore this pathway is important for the synthesis of this vital co-factor (Gal and Sherman 1980; Han et al. 2010). This situation would be compounded by a reduction in the availability of insulin, a key factor in the transport of glucose into neurons (Oxenkrug 2013). As there is evidence that insulin receptor resistance is a frequent feature of depression, and other major psychiatric disorders and with age-related pathology associated with the dementias (Lee et al. 2013), it seems reasonable to conclude that a chronic decrease in high energy substrates resulting from a deficit in glucose and essential co-factors may be of crucial importance in understanding the causes of increased neuronal apoptosis (Lee et al. 2013). This situation is further complicated by mitochondrial dysfunction in depression which results in a decrease in the synthesis of adenosine triphosphate (ATP) and related high energy molecules, combined with an increase in oxidative damage, while the synthesis of superoxide radicals resulting from a decrease in the respiratory chain increases the damage to the mitochondrial membranes by opening the permeability transition pores (Sas et al. 2007). In addition, oxygen free radical synthesis is enhanced by xanthenuric acid and 3-hydroxykynurenine which are formed in the brain as a result of the inflammation enhanced tryptophan–kynurenine pathway. This will be further discussed later.
An essential co-factor in the control of many of the intermediates in the tryptophan–kynurenine pathway is pyridoxal-5-phosphate (P5P), the active form of vitamin B 6. It is well established that vitamin B6, together with vitamin B12 and folate, is involved in the methylation reactions that contribute to the synthesis of the monoamine neurotransmitters, phospholipids and nucleotides, all of which are functionally compromised in depression. Thus a deficiency of dietary vitamin B6 could have an impact on depression and recent studies have demonstrated that low plasma P5P levels are inversely correlated with the severity of depressive symptoms particularly in the elderly (Merete et al. 2008). Other investigators have reported that the B vitamins reduced the symptoms of major depression in post stroke patients (Almeida et al. 2010) while, in a Japanese study, a higher vitamin B6 status was associated with a decreased risk of depression (Nanri et al. 2013). It should be noted however that not all epidemiological studies on the vitamin status have reported the beneficial effects of vitamin B 6 (Sanchez-Villegas et al. 2009).
In the tryptophan–kynurenine pathway, P5P is a key co-factor for kynureninase, kynurenine aminotransferase and kynurenine monooxygenase. A deficit in P5P would therefore result in a shift in the synthesis of 3-hydroxykynurenine from NAD+ synthesis to the synthesis of xanthenuric acid. Xanthenuric acid contributes to insulin insensitivity by forming a chelation complex with insulin, thereby resulting in a reduction in the function of insulin (Oxenkrug 2013). Other changes caused by xanthenuric acid also occur in the pancreatic islet cells (Hattori and Kotake 1984). Support for these findings has been provided by the early clinical studies in which the urinary excretion of xanthenuric acid was shown to be increased in patients with type 2 diabetes (Hattori and Kotake 1984), and by experimental studies which demonstrated that xanthenuric acid induced diabetes in rats (Kotaki et al. 1975). Such observations are of relevance to major depression where it has been reported that there is a 65 % increased risk of diabetes (Campayo et al. 2010).
The reduction in kynureninase and kynurenine monooxygenase associated with reduced P5P would lead to an increase in the kynurenine–kynurenic acid component of the pathway and thereby further contribute to insulin insensitivity. This is due to the fact that kynurenic acid, which acts as an agonist at N-methyl-d-aspartate glutamate receptors, has been shown experimentally to inhibit glucose metabolism (Lam et al. 2010).
The Metabolic Syndrome and Chronic Low Grade Inflammation
The metabolic syndrome in major psychiatric disorders such as depression and schizophrenia is characterised by central obesity, dyslipidaemia, hypertension and type 2 diabetes, changes which are linked to insulin insensitivity. Vascular induced cognitive impairment and late-life depression are also associated with the metabolic and inflammatory status that commonly occur in chronic depression (Dilman et al. 1979; Oxenkrug 2011). A common feature of all these conditions is chronic, low grade inflammation, in which the initiating factors are the pro-inflammatory cytokine and inflammatory chemokines. There are a substantial number of publications which demonstrate the importance of the cytokines in major depression (Kim et al. 2007; Leonard 2010; Maes 1995; Maes et al. 1999; Raison et al. 2006) and of these interferon-gamma appears to play a critical role by activating indoleamine dioxygenase (IDO) and thereby decreasing the synthesis of brain serotonin by increasing the activity of the tryptophan–kynurenine pathway (see Fig. 11.1). This results in an increase in the end products of the pathway, quinolinic and picolinic acids. These neurotoxins activate inducible nitric oxide synthase, thereby increasing the concentration of nitric oxide and the nitrosylation of macromolecules including nucleic acids (Cuzzocrea et al. 2001). This process would be enhanced by a deficiency of P5P.
The activity of interferon-gamma is reflected in the concentration of neopterin by the activation of guanosine triphosphate (GTP) cyclohydrolase to form dihydrobiopterin, the precursor of neopterin (Sucher and Schroecksnadel 2010; Oxenkrug 2011; Fuchs and Avanzas 2009).
The relationship between inflammation, tetrahydrobiopterin and nitric oxide synthesis is complex. Interferon-gamma, released from Th-I helper cells, natural killer cells and cytotoxic T-cells, induces GTP-cyclohydrolase, which facilitates nitric oxide synthesis via tetrahydrobiopterin (Wirleitner et al. 2005). In the presence of adequate tissue tetrahydrobiopterin, nitric oxide is the main end product. However, should there be a deficiency of tetrahydrobiopterin, which may occur in depression and contribute to a deficiency in monoamine neurotransmitters for which it is an essential co-factor (Moens and Kass 2007; Werner-Falmayer et al. 2002), nitric oxide synthase mainly produces superoxide anions which react with any nitric oxide present to form cytotoxic peroxynitrite (Sperner-Unterweger et al. 2014). The importance of tetrahydrobiopterin in inflammation and the consequences of its deficiency in the neurotoxic changes associated with chronic depression have been reviewed by Sperner-Unterweger et al. (2014) and serve to emphasise the importance of dietary co-factors as causative factors in major depression.
Nitric oxide plays a critical role in inflammation not only by activating the glutamatergic system, thereby contributing to neuronal apoptosis, but also by increasing the mobilisation of arachidonic acid in the neuronal membranes (Stewart 2007). An important inflammatory product of arachidonic acid is prostaglandin E2 (PGE2), formed by the action of cyclooxygenase-2 (COX-2).
COX-2 is induced by inflammation but also contributes to the inflammatory cascade by activating the EP2 receptors located on microglia (Jiang et al. 2011). Thus the activation of the tryptophan–kynurenine pathway and IDO by inflammatory mediators contributes directly to neuronal apoptosis and neurodegeneration. These effects are further complicated by the consequences of insulin insensitivity and mitochondrial dysfunction (Sas et al. 2007).
Depression, Inflammation and Dementia: The Metabolic Link?
While a history of depression, particularly in the elderly, is an important risk factor for dementia in later life (Jorm 2001; Chen et al. 1999; Olazaran et al. 2013), the precise mechanism linking depression with dementia is unclear. From the clinical perspective, chronic depression could be a prodrome of dementia and it seems possible that, as a consequence of low grade inflammation, damage to neuronal networks ultimately contributes to the onset of dementia. This has been reviewed by Leonard (2001a, b) and by Leonard and Myint (2006).
Several large epidemiological and clinical studies have demonstrated an association between chronic depression and Alzheimer’s disease. There is also evidence that depression increases the risk of Alzheimer’s disease (Byers and Yaffe 2011; Devanand et al. 1996; Ownby et al. 2006; Wilson et al. 2002). While the relationship between the pathology of depression and Alzheimer’s disease is undoubtedly multi-factorial, there is evidence from the Rotterdam Scan Study of Geerlings et al. (2000) that the risk of Alzheimer’s disease increased from 2.3-fold for late onset depression to 3.8-fold for early onset depression. Thus the longer the duration of the disorder, the greater the increased risk of Alzheimer’s disease. It is possible that while cerebrovascular complications may increase the risk of late-life depression (Lesser et al. 1996) this is less likely to be a precipitating factor in early life onset depression. One possible explanation for the association of early life depression with dementia involves an increase in the synthesis of beta amyloid plaque synthesis, as suggested by the study of Rapp et al. (2006) who showed that the post mortem beta amyloid plaque density of patients with Alzheimer’s disease was significantly increased in those who had chronic depression before they developed dementia. Other researchers have demonstrated that the plasma beta amyloid levels are increased in depressed patients which may provide a possible prodromal marker of Alzheimer’s disease (Sun et al. 2008). Further support for this view has been provided by Namekawa et al. (2013) who demonstrated that the serum beta amyloid (Abeta) 40/Abeta 42 ratio in early onset and in elderly depressed patients was significantly higher in depressed patients than in elderly non-depressed and that the ratio was negatively correlated with the age of onset of the depression. This not only demonstrates an important link between depression and Alzheimer’s disease but also identifies a possible blood marker as a risk factor for the disease in later life.
The association between physical ill-health and chronic depression also plays a role in predisposing the patient to dementia. Cerebrovascular disease, hypertension and type 2 diabetes are just some of the important changes that contribute to cognitive impairment in dementia and chronic depression in the elderly (Launer 2002; Barnes et al. 2006). Dotson et al. (2010) have also shown that recent depressive symptoms and the incidence of dementia are associated with mild cognitive impairment, changes which may be associated with hippocampal and pre-frontal cortical dysfunction. The link between cognitive decline and the metabolic syndrome has been demonstrated recently in the cohort study of participants in the Action to Control Cardiovascular Risk in Diabetes (ACCORD-MIND) trial in which it was shown that depression in patients with type-2 diabetes was associated with greater cognitive decline (Sullivan et al. 2013).
Inflammatory mechanisms undoubtedly play an important role in the pathophysiology of Alzheimer’s disease, and inflammatory cytokines in the vicinity of the beta amyloid deposits and neurofibrillary tangles are suggestive of a causal link between inflammation and the pathology of Alzheimer’s disease. Of the various pro-inflammatory cytokines that are increased in depression and Alzheimer’s disease, tumour necrosis factor (TNF)-alpha is known to be associated with amyloid plaques and to stimulate beta amyloid synthesis (Park and Bowers 2010). The activity of TNF-alpha is mediated through its two membrane bound receptors, TNF R1&2, which are detached from the cell surface by the TNF-alpha converting enzyme (TACE) into their soluble forms. The activity of TACE has been shown to increase in the CSF of patients with Alzheimer’s disease and also in those with mild cognitive impairment, a condition which is often a prelude to Alzheimer’s (Jiang et al. 2011); Zhang et al. (2013) also reported that TACE activity and the concentration of TNF receptors in the plasma are significantly increased in both these groups when compared to healthy controls. Zhang et al. (2013) suggest that TNF-alpha signalling may drive the pathology of Alzheimer’s disease and contribute to the neurotoxic changes induced by beta amyloid. In addition, the changes in TACE and the soluble TNF-alpha receptors correlate with the plasma apolipoprotein (apo) E4 allele concentration, an association which may be linked to higher systemic and brain concentrations of TNF-alpha and IL-6 (Lynch et al. 2003); both these pro-inflammatory cytokines are also elevated in patients with major depression (Maes 1999).
From these clinical observations, it seems reasonable to conclude that the metabolic changes associated with chronic inflammation in depression not only contribute to the physical disorders that are co-morbid with chronic depression but are also causally related to the neurodegenerative changes that are causally linked to dementia. While specific neurotoxins, such as the products of the tryptophan–kynurenine pathway, no doubt play a critical role in these changes, it is possible that the underlying metabolic changes associated with insulin insensitivity and mitochondrial dysfunction are responsible for the fundamental changes underlying dementia. Perhaps it is now time to consider ways in which these metabolic changes could be reversed in the early stages of cognitive decline before the neurodegenerative changes become dominant.
Could Diet Play a Role in Attenuating the Metabolic Changes in Depression?
The relationship between life-threatening infections (such as meningitis, sepsis and cerebral malaria) that involve the dramatic release of pro-inflammatory cytokines and other inflammatory mediators from central and peripheral immune cells are well known to cause profound metabolic changes (Douglas and Shaw 1989; Balkwill et al. 1990; Waage et al. 1989). However, the question arises if similar metabolic changes occur in patients in which chronic low grade inflammation occurs and which are not associated with life-threatening external causes of infection such as pathogenic viruses or bacteria. The chronicity of inflammation in major psychiatric disorders such as depression and schizophrenia, and the dementias, raises the possibility that the dietary content of anti-oxidants, anti-inflammatory lipids and vitamin co-factors which reduce inflammation may have a role to play in modulating low grade inflammation. There are a number of publications that have considered this possibility and the topic will only be briefly covered here (Gimble 1992, 1998; Warren et al. 1987; Grunfeld and Feingold 1992).
Role of anti-oxidants. As free radicals of oxygen and nitrogen are produced as part of the inflammatory cascade that are an important means of defence against invading microorganisms, endogenous anti-oxidants (such as vitamin E and other tocopherols, vitamin C, beta carotene, glutathione, caeruloplasmin and metallothiones) are also produced to counteract the damaging effects of the free radicals. In addition, there are numerous enzymes which destroy intracellular free radicals which include superoxide didmutase, catalase, glutathione peroxidise and reductase. These enzymes require selenium, copper and zinc as co-factors (Gimble 1998). Thus an adequate dietary source of anti-oxidant vitamins and trace metals to counteract any deficits induced by chronic inflammation could be an important adjunctive treatment to the psychotropic drugs that are required to treat the symptoms.
Role of polyunsaturated fatty acids. The lipid components of the neuronal membranes determine the types of inflammatory mediators that are released by an inflammatory response. The n-3 and n-6 fatty acids are important components of the neuronal membranes and the ratio of these fatty acids affects the relative proportion of inflammatory to the anti-inflammatory components. Thus the relative excess of n-6 fatty acids promotes the formation of arachidonic acid which acts as precursor for the prostaglandins and other eicosanoids involved in the inflammatory response (Calder 2008). By contrast, the eicosanoids produced from the n-3 fatty acids, such as eicosanopentaenoic acid (EPA) and docosahexaenoic acid (DHA), exhibit anti-inflammatory effects and inhibit the actions of pro-inflammatory cytokines such as IL-1 beta; IL-1 synthesis is increased in the ageing brain and even more extensively in the brain of the patient with Alzheimer’s disease (Griffin et al. 1985; Song et al. 2003, 2004). Such results suggest that the composition of dietary polyunsaturated fatty acids could influence the synthesis of PGE2 and related inflammatory mediators that are derived from arachidonic acid.
The potential importance of diet in inflammation is reflected in the evolutionary changes in human diet whereby the ratio of n-6 to n-3 fatty acids changed from approximately 1:1 in the early diet of mankind to 10:1 in the modern diet (Simopoulos 2003). In addition to the reduction in endogenous anti-inflammatory eicosanoids in depression (Maes et al. 1999), there is also an increase in pro-inflammatory mediators associated with many psychiatric and neurological disorders. In chronic depression, Alzheimer’s disease, chronic epilepsy and Parkinson’s disease, the over-expression of IL-1 has been implicated in the initiation and progression of the neuropathological changes, changes that are linked to the activation of microglia and to the synthesis of beta amyloid (Griffin et al. 1985, 1995). Furthermore, the increase in IL-1 is linked to a decrease in synaptic plasticity which in the hippocampus is reflected in a decrease in long-term potentiation and learning (Murray and Lynch 1998; Song and Horrobin 2004). Such changes may help to explain the cognitive impairment associated with chronic depression, ageing and dementia; in rats chronic EPA treatment prevents the impairment of memory caused by IL-1 (Song et al. 2004). While the precise mechanism for this anti-inflammatory effects of EPA is uncertain, there is evidence that the n-3 fatty acid, DHA, is converted by microglia to the potent anti-inflammatory 17-resolvins by cyclooxygenase-2 (Serhan et al. 2000) The resolvins block the synthesis of pro-inflammatory cytokines in microglia by inhibiting the activation of nuclear factor kappa-beta (NFκB) (Marcheselli et al. 2000). In addition to the effects of EPA on microglia induced inflammation, it is also evident that the fatty acids increase the concentration of the anti-inflammatory cytokine IL-10, which acts as an antagonist of inflammatory cytokines in vivo (Bluthe et al. 1999). These experimental observations of the effects of EPA have been extended by Song et al. (2009) to demonstrate that the subcellular inflammatory changes initiated by bilateral olfactory bulbectomy in the rat (a chronic model of depression; Leonard and Song 2002) were attenuated by chronic treatment with EPA.
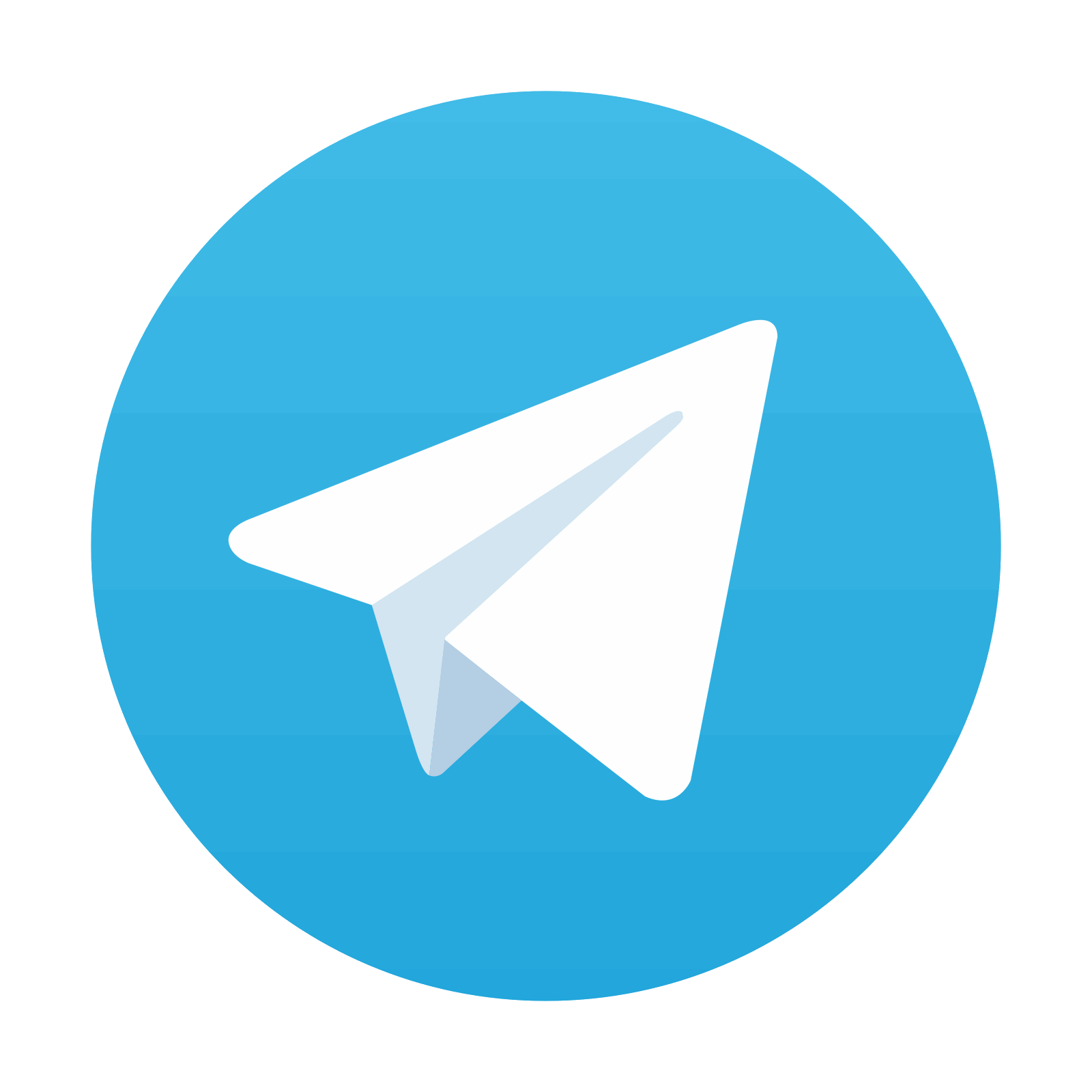
Stay updated, free articles. Join our Telegram channel

Full access? Get Clinical Tree
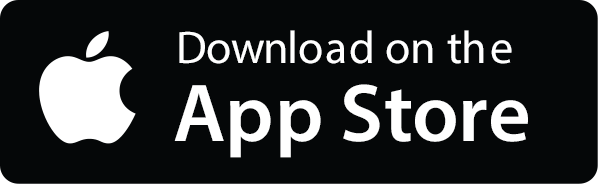
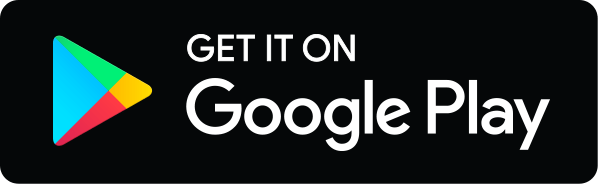