3 Depth Electrodes in Invasive Epilepsy Monitoring Since the inception of electroencephalography (EEG) by Berger,1 our understanding of the pathophysiological changes associated with neurological disorders has dramatically improved. EEG recordings have been particularly useful in helping clarify the electrophysiological basis of seizures. Seizures are abnormal neuronal discharges entrained by the synchronous and often oscillatory firing2,3 of large neuronal ensembles.4 Although theoretically many parts of the brain are capable of generating seizure-like discharges, the clinically relevant seizures are associated with the neocortex, the hippocampal archicortex, and corticothalamic networks. Epilepsy is a disorder of abnormal neuronal excitability. Possible electrophysiological mechanisms accounting for epileptogenesis include abnormal synaptic inhibition,4 aberrant ephaptic5 or electrotonic6,7 transmission, and deregulated ion channels.8–11 At the clinical level, the etiology of seizure generation is broad and includes lesions such as tumors, infections, vascular malformations, and hemorrhages; structural abnormalities, including mesial temporal sclerosis, cortical dysplasia, and neuronal ectopias; and genetic mutations that produce specific seizure phenotypes.8 In <30% of all cases, seizures cannot be attributed to a specific anatomical, functional, or genetic abnormality and are then considered idiopathic in origin. The first line of treatment for nonlesional seizure disorders is medical management with antiepileptic agents. The pharmacological approach, however, is ineffective in many patients. In medically intractable cases of nonlesional epilepsy where a single focal origin is suspected, multiple diagnostic modalities are used to help identify the pathogenetic area: magnetic resonance imaging (MRI), functional MRI (fMRI), positron emission tomography (PET), subtraction ictal single-photon emission computed tomography (SPECT) co-registered to MRI (SISCOM), magnetoencephalography (MEG), and surface EEG. MRI, for example, is useful in identifying anatomical abnormalities such as mesial temporal sclerosis or neuronal ectopias, wheras PET and SISCOM can point to metabolically aberrant brain areas that may generate seizures.12 MEG has been used in some centers to localize prominent synchrony and rhythmicity associated with epileptogenic foci.13 In some cases, scalp EEG points out a focal origin of seizures. These diagnostic tools can direct the surgeon to a confined resect able locus, if they are concordant with the clinical semiology. Scalp EEG electrodes, however, sit far from the underlying current sources and sinks, and their ability to localize ictal onset zones is limited by signal attenuation by the underlying skin and cerebrospinal fluid (CSF), as well as muscle artifact. For these reasons, scalp EEG often fails to identify the precise region of ictal onset. Invasive electrophysiological monitoring can help better localize ictal and interictal events, thus facilitating the identification of the epileptogenic focus and a possible therapeutic resection.14 Simultaneous scalp and subdural recordings demonstrate the power of implanted electrodes to identify the precise origin and propagation patterns of seizures that cannot be localized with scalp recordings.15 Invasive monitoring is achieved via placement of either subdural electrodes (e.g., strip and grid electrodes) or depth intraparenchymal electrodes.14,16 In this chapter, we will focus on depth electrodes. Although subdural electrodes can provide high-quality recordings from a large number of neocortical sites, several deep structures that are considered highly epileptogenic and surgically treatable17,18 can be monitored more efficiently with depth electrodes.16 Such structures are found in the medial temporal lobe and include the hippocampus, amygdala, entorhinal cortex, and parahippocampal gyrus.19 Although subtemporal strip electrodes can sample the mesial temporal lobe, they cannot always be placed reliably and can mislocalize the ictal onset zone compared with stereotactically placed depth electrodes.20 Recordings from the cingulate gyrus, which is buried within the interhemispheric fissure, can be greatly facilitated by the use of depth electrodes. Likewise the insula is most easily sampled with depth electrodes.21 Moreover, some institutions use depth electrodes to record from the depth of sulci or even from the neocortical layer.22 It has been postulated that the use of depth electrodes in stereoelectroencephalography22–24 can provide a three-dimensional map of the seizure focus and the spread of the discharge.25 Finally, depth electrodes can be used to electrophysiologically monitor the activity of ectopic neuronal tissue located deep within the cerebral hemispheres such as periventricular heterotopias and hypothalamic hamartomas.26 Depth electrodes are typically of low impedance and, thus, record local field potentials (LFPs), the compound activity of neuronal ensembles.14 However, there exist depthelectrodes capable of capturing activity from single neurons. More recently, microdialysis probes have been inserted intraparenchymally to monitor simultaneously neurotransmitter levels and neuronal activity.27 Depth electrodes are used not only to record electrophysiological activity during ictal states and interictally; they can be used to functionally map the brain areas where they are inserted, which is of paramount importance when epileptogenic foci are adjacent to eloquent brain.14 Moreover, singleunit recordings have been used to correlate neuronal activity to specific behavioral states, as well as to characterize oscillatory activity during seizure generation.2,3,28 Depth electrodes target deep structures that, by definition, are not visible to the surgeon. It is therefore essential to plan their trajectory using stereotaxy. The stereotactic placement of depth electrodes may be achieved by several means. An imaging modality, such as computed tomography (CT) or MRI, and the creation of stereotactic space, either frame-based or frameless, are essential components to a successful placement. In recent years, the advent of high-resolution gadolinium-enhanced MRI has rendered it the preferred imaging modality for surgical planning. Gadolinium-enhanced MRI has the advantage of not only differentiating well between gray and white matter, thus reliably delineating deep structures such as the amygdala and hippocampus from the adjacent white matter, but also identifying vascular structures that may be encountered in the electrode trajectory. The electrode path is carefully chosen so as to avoid injury to critical vascular structures, and, if possible, to not intersect the ventricular system. There are two main issues that arise from an intersection of the electrode trajectory and the ventricles, and the resulting CSF loss from the ventricle through the electrode track. First, an acute reduction in intraventricular volume can generate compensatory shifts in the topography of the brain parenchyma, which in turn may result in erroneous placement of the electrode away from its target. Second, if the depth electrodes are used for extended periods of time for invasive electrophysiological monitoring, a sustained CSF leak through the skin may predispose toward meningitis. It is thus preferred to plan trajectories that spare the ventricular system, although in certain cases this cannot be avoided. In fact, some authors recommend placement of the electrode within the ventricles to avoid damaging the hippocampus and potential “injury spikes.”29 We have not found this approach as reliable as intraparenchymal placement for providing reliable data. Although stereotactic frames are still used in many institutions for depth electrode implantation, frameless stereotaxy can also be used with good accuracy and results. At our institution, we have exclusively used frameless stereotaxy for depth electrode placement in the context of epilepsy the past few years and have found it to be reliable and accurate (Fig. 3.1). 30
Indications for Depth Electrode Placement
Surgical Approach to Implantation of Depth Electrodes
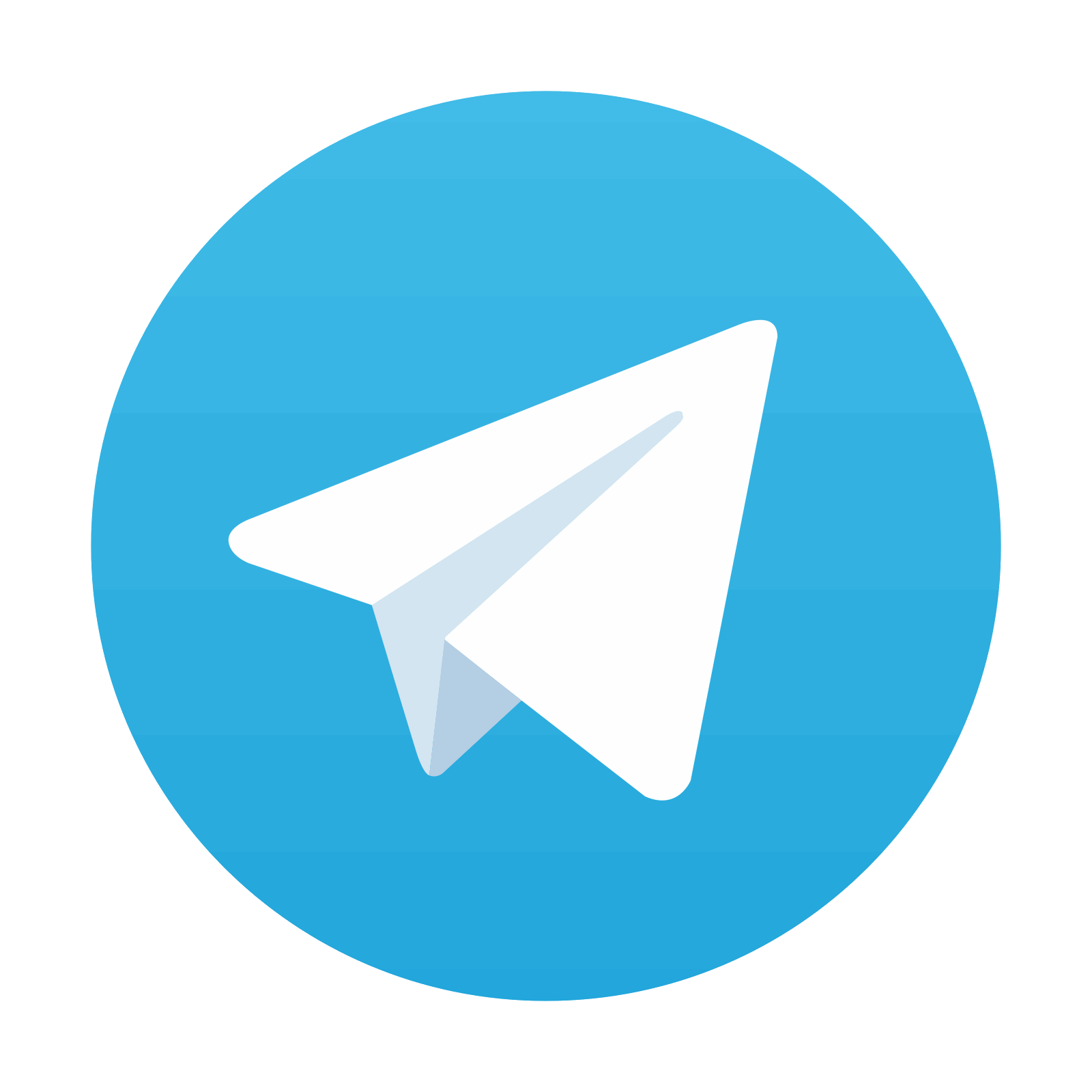
Stay updated, free articles. Join our Telegram channel

Full access? Get Clinical Tree
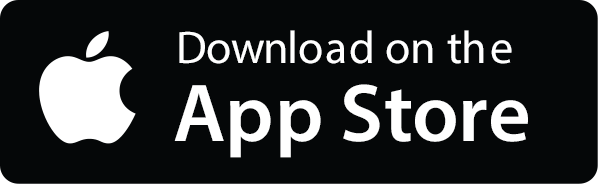
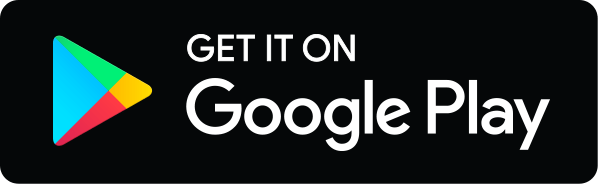