Stage
Treatment threshold
Treatment
Response criterion
Prophylaxis
Periodic discharges lasting ≥1 min
Start maintenance therapy with ASD #1
No progression to seizures
1st line therapy
Single seizure lasting ≥1 min (with any anatomical extent)
Loading dose of ASD #1, increase maintenance dose of ASD #1
Seizure burden remains below next treatment threshold for ≥24 h
2nd line therapy
Single seizure lasting ≥2 min, or 3 or more seizures within 1 h each lasting ≥1 min
(anatomical extent ≥2 channels)
Loading dose of ASD #2, start maintenance therapy of ASD #2
Seizure burden remains below next treatment threshold for ≥24 h
3rd line therapy initiation
Single seizure lasting ≥2 min, or 3 or more seizures within 1 h each lasting ≥1 min
(anatomical extent ≥2 channels)
Start infusion of anesthetic drug #1
Seizure burden remains below next treatment threshold for ≥24 h
3rd line therapy escalation
Single seizure lasting ≥2 min, or 3 or more seizures within 30 min each lasting ≥1 min
(anatomical extent ≥2 channels)
Increase infusion rate of anesthetic drug #1, up to a pre-defined maximum
Seizure burden remains below next treatment threshold for ≥24 h
4th line therapy initiation
Single seizure lasting ≥5 min, or 3 or more seizures within 30 min each lasting ≥2 min
(anatomical extent ≥2 channels)
Start infusion of anesthetic drug #2
Seizure burden remains below next treatment threshold for ≥24 h
4th line therapy escalation
Single seizure lasting ≥5 min, or 3 or more seizures within 30 min each lasting ≥2 min
(anatomical extent ≥2 channels)
Increase infusion rate of anesthetic drug #2, up to a pre-defined maximum
Seizure burden remains below next treatment threshold for ≥24 h
Accounting for the Influence of Seizure Etiology
One of the greatest challenges in designing effective interventional studies for acute repetitive seizures and SE is accounting properly for the contribution of the underlying seizure etiology. Etiology has long been recognized as an important determinant of both the refractoriness of acute seizures to treatment and long-term outcome. Therefore, studies must be carefully designed to take underlying seizure etiology into account. The seizure etiology may directly worsen outcome through a direct brain injury that is independent of seizures (e.g., stroke, hypoxic ischemic brain injury, or traumatic brain injury), or etiology may modify the deleterious effects of seizures, or both. For example, in the context of traumatic brain injury, seizures have been shown to be associated with a state of local “metabolic crisis” [24]. Perhaps the simplest solution to this problem is to limit study enrolment to patients with only a single etiology. This strategy, however, poses other challenges: the etiology is not always known at the time of seizure onset and study enrolment, and restrictive enrolment criteria can severely reduce recruitment rates. Furthermore, even when studying a single etiology, variations in etiology-specific injury severity must still be measured (e.g., neuroimaging measures of infarct volume or traumatic brain injury severity) and factored into analyses of the relationship amongst seizure burden, treatment, and outcomes. Studies that do include patients with multiple seizure etiologies must either stratify the analysis of outcomes by the underlying etiology or, if pooling the analysis of multiple etiologies, consider the possible effect modification of etiology on seizure burden (Fig. 29.1) [25]. In certain cases, the influence of etiology on outcome will likely overwhelm the effects of seizures (e.g., anoxic brain injury) or may mitigate their effects (e.g., epilepsy of presumed genetic origin). Therefore, careful consideration of the influence of seizure etiology is crucial to successful study design and execution.


Fig. 29.1
Schematic illustration of potential relationships between seizure burden and outcome. The potential deleterious effects of seizures in the context of acute brain injury are likely to depend on the underlying etiology. The probability of poor outcome may increase linearly or exponentially with increasing seizure burden, or a threshold may exist, above which seizures are harmful. (From Hahn and Jette [25], with permission)
From Clinical Trials to Individualized Therapy
Although much attention has been paid to shortening the time to seizure cessation by optimizing the use of conventional ASDs and coma-inducing drugs, the most important factor determining outcome remains the underlying etiology [26]. Rapid identification and treatment of underlying etiologies that are amenable to intervention would likely optimize outcome further.
While the search for an underlying etiology is often straightforward in patients with known cerebral structural abnormalities such as a brain tumor, stroke, or traumatic brain injury, when no such known condition is present the search may be more vexing. Nevertheless, persistence in the search for an etiology can reap significant rewards. In a study of patients with new onset refractory status epilepticus (NORSE) in whom an underlying etiology was not identified within 48 h of admission, subsequent investigations identified an autoimmune etiology in 19% and a paraneoplastic etiology in 18% [27]. Still, in more than 50% of these patients no etiology was found despite extensive investigations, indicating substantial room for improvement in current diagnostic approaches [27]. Identifying the underlying etiology of patients with cryptogenic SE will be of paramount importance in enabling etiology-specific therapies that have the potential to improve outcome.
The search for the underlying etiology for cryptogenic SE is often difficult, largely due to the fact that a large number of illnesses may manifest as SE, possibly via a common terminal mechanism. Cases of SE that remain cryptogenic after initial investigation are likely due to a variety of underlying causes, rather than to some common as-yet unknown cause. Therefore, strategies to identify etiologies that affect only a small number of patients or even unique patients should be implemented. Such rare causes of SE include mitochondrial disease and other inborn errors of metabolism [28, 29].
The identification of autoimmune diseases as an important cause of cryptogenic SE (and epilepsy in general) is an example of the remarkable successes that can be achieved by etiology-specific diagnosis and therapy. These findings serve as a model for identifying other SE etiologies. The recognition that extreme delta brushes on EEGs are found in patients with anti-NMDA receptor encephalitis has been instrumental in the early identification of these patients, allowing rapid initiation of immunomodulatory therapy even before the specific autoantibodies are confirmed [30]. The discovery of extreme delta brushes was made possible by a multicenter collaborative effort, demonstrating the importance that collaborative research has for the characterization of cryptogenic SE etiologies.
Although an autoimmune etiology may be suspected quite early, it can take months or even years to identify specific causative autoantibodies [31]. To address this challenge, we propose the establishment of a centralized repository for banking of serum from patients with cryptogenic SE, with the goal of facilitating substantial advances in diagnosis. In addition to serum samples, this centralized repository would include detailed clinical information, imaging data, EEG tracings, and cerebrospinal fluid samples when available. In addition, genotyping and metabolomics analyses could be performed centrally or through a bio-specimen core. A careful curation process would need to be put in place to assure the integrity of the collected data.
The power of such a repository would be increased markedly by making it publicly available through an easy to navigate web-based interface. For example, the Cancer Genome Atlas is a repository of carefully curated datasets that, due to its publicly available nature, has benefitted nearly every aspect of cancer research by identifying 10 million new mutations that represent key drivers for a number of cancers, leading to more effective targeted therapies. The Epilepsy Phenome/Genome Project (EPGP) is another model of a multi-institutional study in which detailed phenotypic characteristics and DNA samples were bio-banked. Key to the success of both of these bio-banks is their core-based organizational structure, in which a number of institutions each serves distinct administrative or scientific functions [32].
The challenges of creating and maintaining such a repository are formidable. Refractory SE remains a relatively rare entity, so data will have to be collected by a large number of sites. Multifactorial data, often large and complex in nature due to high throughput genomic methods, continuously recorded physiologic and electrographic data, and high-resolution multimodal imaging technologies, must be collected and integrated in a standardized manner, addressing security and patient confidentiality [33, 34]. Such a repository would require a substantial investment in infrastructure. Technologic advances may present opportunities for research studies performed using such a dataset. As high-fidelity DNA and RNA sequencing is becoming more standardized and more widely available, novel methods of sharing such data are being developed [35]. High-quality institutional bio-banks may also facilitate this process and reduce costs [34]. Finally, it is increasingly recognized that strong financial incentives to identify, enroll, and complete study procedures are an essential driver for study completion [32]. Poorly funded registries that depend on investigator volunteerism are less likely to produce meaningful advances.
Recently, it has become clear that large collaborative efforts can drive significant advances in the field of epilepsy [36]. Although multicenter research entails certain logistical and technological challenges, maintaining clear lines of communication is often the biggest challenge. Therefore, the most important piece of infrastructure is the establishment of methods of clear, transparent communication that is inclusive of a large number of disparate centers, so that repository and study goals can be established efficiently. With this goal in mind, the Critical Care EEG Monitoring Research Consortium (CCEMRC) developed a common terminology to describe EEG patterns in patients undergoing CEEG monitoring [37]. Although initially developed for research purposes, this has evolved into the American Clinical Neurophysiology Society’s standard critical care EEG terminology. It is widely used now in clinical practice and was recently incorporated into a multicenter research and clinical database [38]. In addition, several multicenter observational SE registries have been established, underscoring the importance of large collaborative efforts [39, 40].
Status Epilepticus in Developing Countries
It is estimated that over 85% of the global burden of epilepsy is borne by resource-poor countries, defined as low- or middle-low-income countries [41]. Although the global burden of epilepsy and SE is difficult to estimate accurately, the incidence and prevalence of epilepsy in developing countries is likely to be markedly higher than in the developed world [42]. Mortality in convulsive status epilepticus is higher across all age groups in resource-poor regions [43, 44]. Furthermore, sequelae from SE such as focal neurologic deficits and cognitive deficits are more frequent in developing regions [42].
Modern operational definitions of SE may not be practically applicable in resource-poor countries due to the frequent lack of documentation regarding seizure duration [41]. Most existing studies have been performed on convulsive SE because the assessment of nonconvulsive SE (NCSE) is difficult in these regions. The incidence of NCSE in developing countries is similar to that in developed countries [45], but the distribution of underlying etiologies is different: febrile SE, infectious etiologies, and traumatic brain injuries are more common in developing countries. Among patients admitted with seizure-related illnesses in those countries, a greater proportion has SE [46].
The epilepsy treatment gap between developing and developed regions remains substantial [47]. Causes of the epilepsy treatment gap include a scarcity of skilled healthcare practitioners, inability to pay for treatment, unavailability of drugs, local cultural beliefs, use of traditional medicines, and distance from healthcare facilities [41, 48]. A concerted effort to address this gap has been made through the World Health Organization and the International League Against Epilepsy (ILAE), specifically to reduce the physical and social burden of epilepsy, to train and educate healthcare professionals, to dispel stigma, to identify and assess interventions that may lead to epilepsy prevention, and to develop a worldwide model for integration into local health systems [49]. Whether these initiatives have actually reduced treatment gaps in SE remains to be demonstrated.
The treatment gap in SE shares many of these challenges, but it differs in other respects. Several common challenges include the unavailability of medications and the distances from health care facilities. Also, because SE may be more readily recognized as a neurologic emergency, it may not share the same social and cultural barriers (such as stigma based on cognitive or psychiatric dysfunction), though it likely shares others (such as fear that seizures are contagious [41]). One significant gap is the inability to pay for comprehensive diagnosis and treatment. The nature of seizures in patients with acute non-traumatic coma mirrors that in developed regions in that most seizures in these patients are nonconvulsive, detectable only through EEG, and are independently associated with poor outcome [50]. Yet, the cost of EEG equipment and, particularly, the trained personnel required to perform and interpret the EEG remain prohibitive in many resource-poor countries.
Although continued advances in the basic healthcare infrastructure of resource-poor countries will help to reduce the treatment gap for SE gradually, there are opportunities to narrow this gap more quickly by harnessing recent advances in technology. Producing ultra-affordable hardware has been demonstrated through projects such as the Raspberry Pi, where a full-functioning, reasonably powered computer could be produced for $5–25 [51]. A similar approach could be taken to design and manufacture an inexpensive basic EEG acquisition system that could be operated with minimal training, to reduce the cost of EEG substantially. Multiple studies have demonstrated the relatively good performance of reduced channel montages in detecting seizures and SE [52–54]. Furthermore, the growth of the telecommunications industry in the developing world, particularly in mobile broadband, has been remarkably rapid, with internet penetration now surpassing 35% [55]. Access to mobile broadband could be harnessed for education and training and creates the possibility for remote EEG review, increasing access to neurophysiologist expertise.
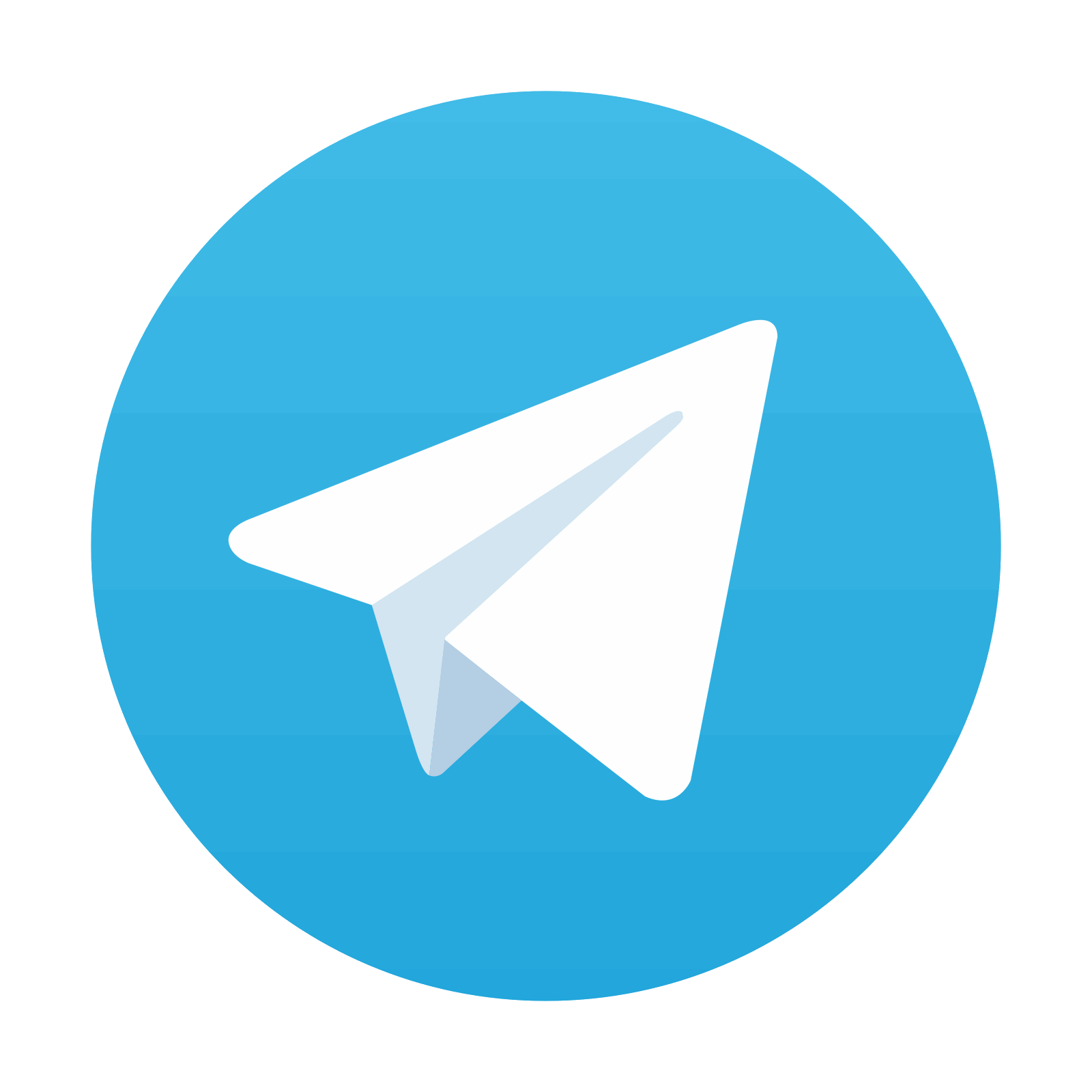
Stay updated, free articles. Join our Telegram channel

Full access? Get Clinical Tree
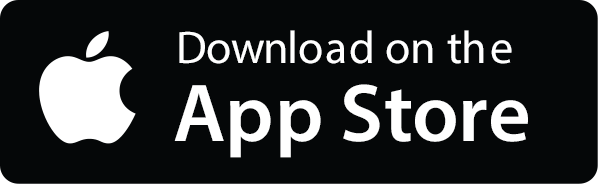
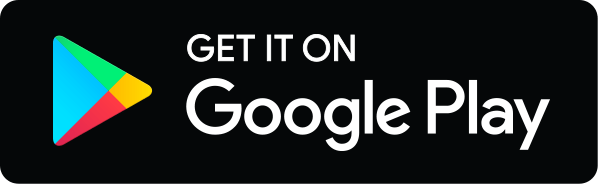