Cellular Aspects of Neural Development
Early in the development of the nervous system, a hollow tube of ectodermal neural tissue forms at the embryo’s dorsal midline. The cellular elements of the tube appear undifferentiated at first, but they later develop into various types of neurons and supporting glial cells.
The embryonic neural tube has three layers (Fig 2–1): the ventricular zone, later called the ependyma, around the lumen (central canal) of the tube; the intermediate zone, which is formed by the dividing cells of the ventricular zone (including the earliest radial glial cell type) and stretches between the ventricular surface and the outer (pial) layer; and the external marginal zone, which is formed later by processes of the nerve cells in the intermediate zone (Fig 2–1B).
The intermediate zone, or mantle layer, increases in cellularity and becomes gray matter. The nerve cell processes in the marginal zone, as well as other cell processes, become white matter when myelinated.
The largest neurons, which are mostly motor neurons, differentiate first. Sensory and small neurons, and most of the glial cells, appear later, up to the time of birth. Newly formed neurons may migrate extensively through regions of previously formed neurons. When glial cells appear, they can act as a framework that guides growing neurons to the correct target areas. Because the axonal process of a neuron may begin growing toward its target during migration, nerve processes in the adult brain are often curved rather than straight. The newer cells of the future cerebral cortex migrate from the deepest to the more superficial layers. The small neurons of the incipient cerebellum migrate first to the surface and later to deeper layers, and this process continues for several months after birth.
Neurons
Neurons vary in size and complexity. For example, the nuclei of one type of small cerebellar cortical cell (granule cell) are only slightly larger than the nucleoli of an adjacent large Purkinje cell. Motor neurons are usually larger than sensory neurons. Nerve cells with long processes (eg, dorsal root ganglion cells) are larger than those with short processes (Figs 2–2 and 2–3).
Figure 2–2
Schematic illustration of nerve cell types. A: Central nervous system cells: (1) motor neuron projecting to striated muscle, (2) special sensory neuron, and (3) general sensory neuron from skin. B: Autonomic cells to smooth muscle. Notice how the position of the cell body with respect to the axon varies.
Figure 2–3
Schematic drawing of a Nissl-stained motor neuron. The myelin sheath is produced by oligodendrocytes in the central nervous system and by Schwann cells in the peripheral nervous system. Note the three motor end-plates, which transmit the nerve impulses to striated skeletal muscle fibers. Arrows show the direction of the nerve impulse. (Reproduced, with permission, from Junqueira LC, Carneiro J, Kelley RO: Basic Histology: Text & Atlas, 11th ed. McGraw-Hill, 2005.)
Some neurons project from the cerebral cortex to the lower spinal cord, a distance of less than 2 ft in infants or 4 ft or more in adults; others have very short processes, reaching, for example, only from cell to cell in the cerebral cortex. These small neurons, with short axons that terminate locally, are called interneurons.
Extending from the nerve cell body are usually a number of processes called the axon and dendrites. Most neurons give rise to a single axon (which branches along its course) and to many dendrites (which also divide and subdivide, like the branches of a tree). The receptive part of the neuron is the dendrite, or dendritic zone (see Dendrites section). The conducting (propagating or transmitting) part is the axon, which may have one or more collateral branches. The downstream end of the axon is called the synaptic terminal, or arborization. The neuron’s cell body is called the soma, or perikaryon.
The cell body is the metabolic and genetic center of a neuron (see Fig 2–3). Although its size varies greatly in different neuron types, the cell body makes up only a small part of the neuron’s total volume.
The cell body and dendrites constitute the receptive pole of the neuron. Synapses from other cells or glial processes tend to cover the surface of a cell body (Fig 2–4).
Figure 2–4
Electron micrograph of a nerve cell body (CB) surrounded by nerve processes. The neuronal surface is completely covered by either synaptic endings of other neurons (S) or processes of glial cells. Many other processes around this cell are myelinated axons (M). CB, neuronal cell body; N, nucleus, ×5000. (Courtesy of Dr. DM McDonald.)
Dendrites are branches of neurons that extend from the cell body; they receive incoming synaptic information and thus, together with the cell body, provide the receptive pole of the neuron. Most neurons have many dendrites (see Figs 2–2, 2–3, and 2–5). The receptive surface area of the dendrites is usually far larger than that of the cell body. Because most dendrites are long and thin, they act as resistors, isolating electrical events, such as postsynaptic potentials, from one another (see Chapter 3). The branching pattern of the dendrites can be very complex and determines how the neuron integrates synaptic inputs from various sources. Some dendrites give rise to dendritic spines, which are small mushroom-shaped projections that act as fine dendritic branches and receive synaptic inputs (Fig 2–5). Dendritic spines are currently of great interest to researchers. The shape of a spine regulates the strength of the synaptic signal that it receives. A synapse onto the tip of a spine with a thin “neck” will have a smaller influence than a synapse onto a spine with a thick neck. Dendritic spines are dynamic, and their shape can change. Changes in dendritic spine shape can strengthen synaptic connections so as to contribute to learning and memory. Maladaptive changes in spines may contribute to altered function of the nervous system after injury, for example, contributing to chronic pain after nerve injury (Fig 2–6).
Figure 2–6
Micrographs showing dendrites of dorsal horn neurons from a normal rat (A) and from a rat following nerve injury (B). Note the increased number of dendritic spines and their altered shape following nerve injury. Bar = 10 μm. (Modified from Tan AM et al: Rac1-regulated dendritic spine remodeling contributes to neuropathic pain after peripheral nerve injury, Exper Neurol 2011;232:222–233.)
A single axon or nerve fiber arises from most neurons. The axon is a cylindrical tube of cytoplasm covered by a membrane, the axolemma. A cytoskeleton consisting of neurofilaments and microtubules runs through the axon. The microtubules provide a framework for fast axonal transport (see Axonal Transport section). Specialized molecular motors (kinesin molecules) bind to vesicles containing molecules (eg, neurotransmitters) destined for transport and “walk” via a series of adenosine triphosphate (ATP)-consuming steps along the microtubules.
The axon is a specialized structure that conducts electrical signals from the initial segment (the proximal part of the axon, near the cell body) to the synaptic terminals. The initial segment has distinctive morphological features; it differs from both cell body and axon. The axolemma of the initial segment contains a high density of sodium channels, which permit the initial segment to act as a trigger zone. In this zone, action potentials are generated so that they can travel along the axon, finally invading the terminal axonal branches and triggering synaptic activity, which impinges on other neurons. The initial segment does not contain Nissl substance (see Fig 2–3). In large neurons, the initial segment arises conspicuously from the axon hillock, a cone-shaped portion of the cell body. Axons range in length from a few microns (in interneurons) to well over a meter (ie, in a lumbar motor neuron that projects from the spinal cord to the muscles of the foot) and in diameter from 0.1 μm to more than 20 μm.
Many axons are covered by myelin. The myelin consists of multiple concentric layers of lipid-rich membrane produced by Schwann cells in the peripheral nervous system (PNS) and by oligodendrocytes (a type of glial cell) in the central nervous system (CNS) (Figs 2–8, 2–9, 2–10, and 2–11). The myelin sheath is divided into segments about 1 mm long by small gaps (1 μm long) where myelin is absent; these are the nodes of Ranvier. The smallest axons are unmyelinated. As noted in Chapter 3, myelin functions as an insulator. In general, myelination serves to increase the speed of impulse conduction along the axon.
Figure 2–7
Diagrammatic view, in three dimensions, of a prototypic neuron. Dendrites (1) radiate from the neuronal cell body, which contains the nucleus (3). The axon arises from the cell body at the initial segment (2). Axodendritic (4) and axosomatic (5) synapses are present. Myelin sheaths (6) are present around some axons.
Figure 2–8
A: In the peripheral nervous system (PNS), unmyelinated axons are located within grooves on the surface of Schwann cells. These axons are not, however, insulated by a myelin sheath. B: Myelinated PNS fibers are surrounded by a myelin sheath that is formed by a spiral wrapping of the axon by a Schwann cell. Panels 1–4 show four consecutive phases of myelin formation in peripheral nerve fibers. (Reproduced, with permission, from Junqueira LC, Carneiro J, Kelley RO: Basic Histology, 11th ed. McGraw-Hill, 2005.)
Figure 2–10
Oligodendrocytes form myelin in the central nervous system (CNS). A single oligodendrocyte myelinates an entire family of axons (2–50). There is little oligodendrocyte cytoplasm (Cyt) in the oligodendrocyte processes that spiral around the axon to form myelin, and the myelin sheaths are connected to their parent oligodendrocyte cell body by only thin tongues of cytoplasm. This may account, at least in part, for the paucity of remyelination after damage to the myelin in the CNS. The myelin is periodically interrupted at nodes of Ranvier, where the axon (A) is exposed to the extracellular space (ES). (Redrawn and reproduced with permission from Bunge M, Bunge R, Pappas G: Ultrastructural study of remyelination in an experimental lesion in adult cat spinal cord, J Biophys Biochem Cytol May;10:67–94, 1961.)
Figure 2–11
Electron micrograph showing oligodendrocyte (OL) in the spinal cord, which has myelinated two axons (A1, A2). ×6600. The inset shows axon A1
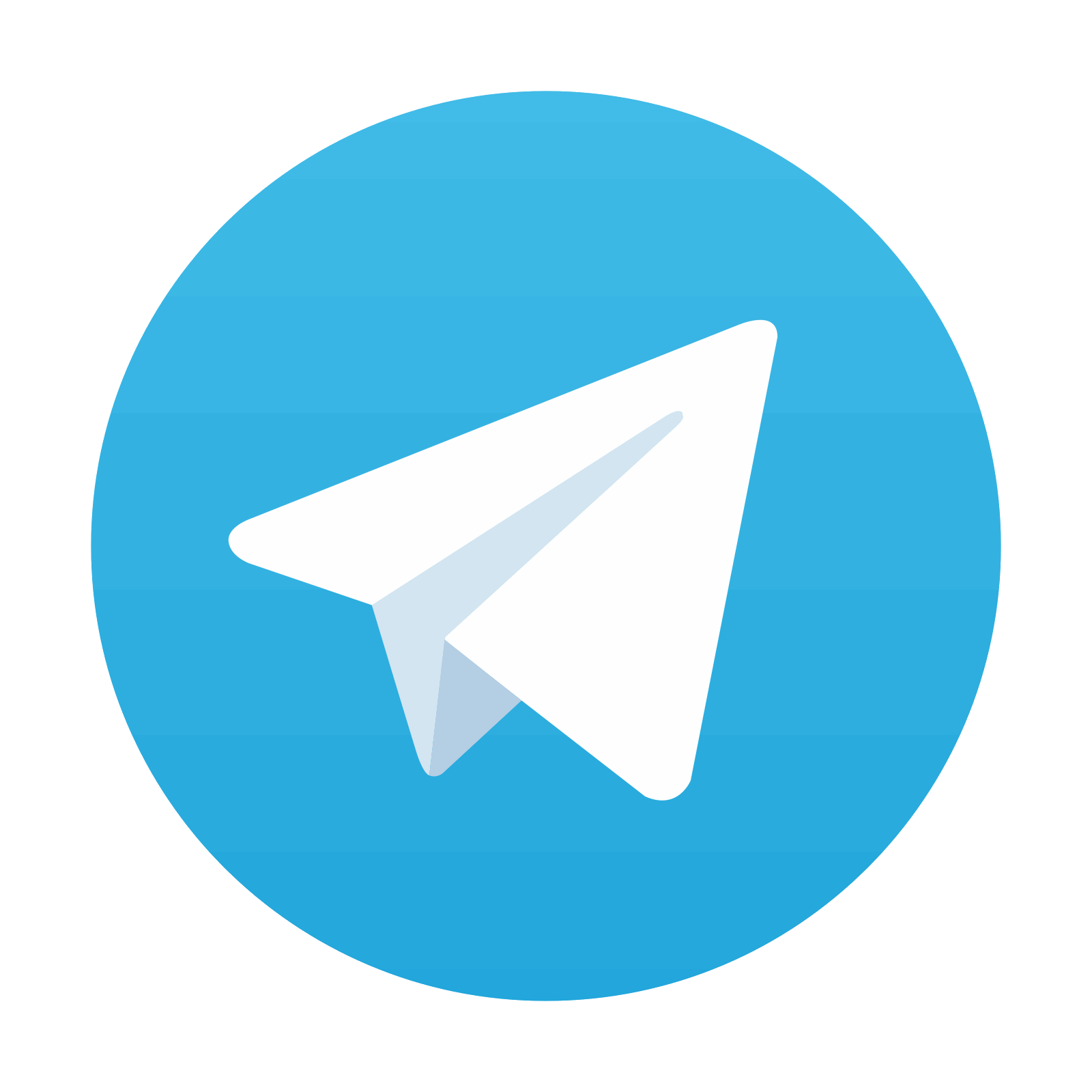
Stay updated, free articles. Join our Telegram channel

Full access? Get Clinical Tree
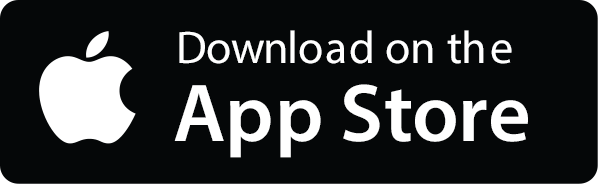
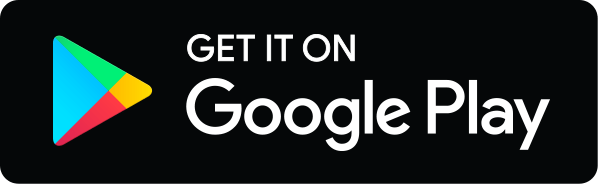
