Immunogen
Principal mode of action
Behavioral and cognitive abnormalities in adult offspring born to immune-challenged mothers
Methodological advantages
Methodological disadvantages
Sensorimotor gating
Selective attention
Social behavior
Exploratory behavior
Working memory
Cognitive flexibility
Sensitivity to psychotomimetic drugs
Influenza
Broad innate and adaptive antiviral immune response, including production of cytokines and antibodies, and activation of B- and T- cells following maternal intranasal application
↓
ND
↓
↓↓
ND
ND
↑
Full spectrum of immune response normally induced by infections, including antibody production
Stringent biosafety precautions necessary
No easy control of immune response intensity and duration
Poly(I:C)
Recognition by TLR3 and induction of cytokine-associated viral-like acute phase response after systemic maternal administration
↓↓↓
↓↓↓
↓↓
↓↓
↓↓↓
↓↓↓
↑↑↑
No stringent biosafety precautions necessary
Strictly limited duration of immune response
Easy control of (cytokine-associated) immune response intensity
Limited immune response spectrum
LPS
Recognition by TLR4 and induction of cytokine-associated bacterial-like acute phase response after systemic maternal administration
↓↓
ND
↓↓
↓
ND
ND
↑
No stringent biosafety precautions necessary
Strictly limited duration of immune response
Easy control of (cytokine-associated) immune response intensity
Limited immune response spectrum
Marked fetal losses due to spontaneous abortion (high doses)
Turpentine
Local tissue damage, recruitment and activation of immune cells, and secretion of pro-inflammatory cytokines after maternal intramuscular injection
↓
ND
ND
ND
ND
ND
↑
No stringent biosafety precautions necessary
Strictly limited duration of inflammatory response
Maternal localization of maternal inflammatory response
Limited immune response spectrum
Muscular injury/trauma
IL-6
Induction of fever and acute phase responses after systemic maternal administration
↓
↓
↓
↓
ND
ND
ND
No stringent biosafety precautions necessary
Strictly limited duration of immune response
Easy control of immune stimulus intensity
Limited immune response spectrum
The mouse prenatal influenza model has recently extended to experimental investigations in rhesus monkeys, demonstrating the emergence of reduced gray and white matter in distinct cortical and parieto-cortical brain regions of neonates born to influenza-infected mothers (Short et al. 2010). The extension of such translational research to rhesus monkeys is especially relevant in the present context, because prenatal corticogenesis is more advanced in primate as compared with rodent species, and therefore, primate models help to verify the relevance of findings in animal models to the human condition. Taken together, the experimental data obtained in mouse and primate prenatal viral infection models can be taken as experimental evidence to support causal effects of prenatal influenza infection in the development of long-term brain abnormalities.
The Present, Part 1: Models of Viral-Like Immune Activation by Polyriboinosinic–Polyribocytidilic Acid
Another class of animal models of prenatal immune challenge makes use of immune-activating agents that evoke cytokine-associated immune responses in the mother without using live viral or bacterial pathogens (Meyer et al. 2009a; Meyer and Feldon 2010). These models were initially developed to test whether altered expression of maternal and/or fetal cytokines might assume a key role in mediating the link between maternal infection during pregnancy and abnormal brain development in the offspring (Gilmore and Jarskog 1997; Meyer et al. 2009b). One of the most popular and widely used methods nowadays is maternal administration of polyriboinosinic–polyribocytidilic acid (poly[I:C]) (Meyer and Feldon 2012). Since its initial application in mouse developmental biology, the prenatal poly(I:C) model has exerted an appreciable impact on researchers concentrating on the neurodevelopmental and neuroimmunological basis of complex human brain disorders such as schizophrenia (Meyer and Feldon 2012).
In the mouse prenatal poly(I:C) model, pregnant dams are exposed to the immunological manipulation at a specific gestational stage, and the brain and behavioral consequences of the prenatal immunological manipulation are then compared in the resulting offspring relative to offspring born to vehicle-treated control mothers (Meyer and Feldon 2012). Poly(I:C) is a commercially available synthetic analog of double-stranded RNA. Double-stranded RNA is generated during viral infection as a replication intermediate for single-stranded RNA or as a by-product of symmetrical transcription in DNA viruses (Akira and Takeda 2004). It is recognized as foreign by the mammalian immune system primarily through the transmembrane protein toll-like receptor 3 (Akira and Takeda 2004). Transmembrane protein toll-like receptors are a class of pathogen recognition receptors that recognize invariant structures present on virulent pathogens. Upon binding to toll-like receptor 3, double-stranded RNA or its synthetic analog poly(I:C) leads to the expression of an extensive collection of innate immune response genes and proteins. The array of these responses involves the production and release of many pro-inflammatory cytokines, including interleukin (IL)-1β, IL-6, and tumor necrosis factor (TNF)-α (Cunningham et al. 2007). In addition, poly(I:C) is a potent inducer of the type I interferons (IFNs): IFN-α and IFN-β (Kimura et al. 1994). Administration of poly(I:C) can therefore efficiently mimic the acute phase response to viral infection (Kimura et al. 1994) and leads to significant inflammatory processes in the fetal brain when given systemically to pregnant dams (Meyer et al. 2006; Abazyan et al. 2010).
As extensively reviewed elsewhere (Meyer et al. 2009a; Meyer and Feldon 2010, 2012), numerous neurochemical and brain morphological abnormalities have been detected in adult mice and rats after maternal gestational exposure to poly(I:C). An increasing amount of rodent studies further provides robust evidence for the emergence of a multitude of behavioral, cognitive, and pharmacological dysfunctions after prenatal poly(I:C)-induced immune activation (Table 2.1). One intriguing feature of the prenatal poly(I:C) model is that the full spectrum of behavioral, cognitive, and pharmacological abnormalities only emerges after the offspring have reached late adolescence or early adulthood (Zuckerman et al. 2003; Ozawa et al. 2006; Piontkewitz et al. 2001; Vuillermot et al. 2010). This maturational delay is indicative of a progression of pathological symptoms from pubescence to adulthood, which is consistent with the post-pubertal onset of full-blown psychotic behavior in schizophrenia and related disorders (Tandon et al. 2009).
Numerous theories have been put forward to explain why most subjects at risk for schizophrenia develop full-blown psychotic disease only after reaching late adolescence or early adulthood. One prevalent hypothesis suggests that this delayed clinical course might be related to the functional maturation of intracortical connectivity, especially within prefrontal-temporolimbic cortical pathways (Weinberger and Lipska 1995). Other theoretical accounts of this maturational delay focus on the influence of (sex-dependent) hormonal refinements occurring during the periadolescent stage of life (Halbreich and Kahn 2003) and/or interactions with exposure to stressful situations and associated changes in the stress-response system (Corcoran et al. 2003). The prenatal poly(I:C) model provides a unique opportunity to identify the developmental character and molecular processes of neuropathological changes across postnatal brain maturation (Meyer and Feldon 2012; Piontkewitz et al. 2012). Such efforts are highly desirable, because therapeutic interventions during the periadolescent life span might represent an effective strategy to reduce the incidence of or even prevent the emergence of multiple brain dysfunctions in high-risk subjects with a (immune-mediated) neurodevelopmental predisposition to adult mental illness. Experimental efforts toward this direction are underway, and the prenatal poly(I:C) model has already provided initial promising data suggesting that periadolescent treatment with reference antipsychotic or antidepressant drugs can successfully block at least some of the behavioral and brain abnormalities in prenatally poly(I:C)-exposed offspring (Meyer et al. 2010; Piontkewitz et al. 2009, 2011; Richtand et al. 2011).
Another important feature of the prenatal poly(I:C) model is that the severity of the long-term brain and behavioral changes is dependent on the intensity of the cytokine-associated immune reaction (Shi et al. 2003; Meyer et al. 2005). The apparent impact of the precise immune stimulus intensity suggests that there is a threshold of (viral-like) immune activation that is required to induce long-term brain and behavioral pathology in the offspring. The dose-dependent nature of the prenatal poly(I:C)-induced effects parallels findings indicating that more severe forms of prenatal immune abnormalities are associated with more intense structural brain abnormalities in offspring affected with schizophrenia (Ellman et al. 2010). Another feasible assumption derived from such dose-response effects is that prenatal (viral-like) infection enhances the risk of schizophrenia only if the infectious process is associated with relatively strong immunological reactions in the maternal/fetal compartments and/or if this early-life immunological insult takes place in conjunction with additional genetic or environmental risk factors (see following).
Finally, the prenatal poly(I:C) model is also very useful to test the hypothesis that the vulnerability to infection-induced neurodevelopmental abnormalities differs between distinct stages of fetal development. This can be achieved by comparing the effects of prenatal poly(I:C) at distinct gestational stages relative to corresponding prenatal control treatment (Meyer et al. 2006, 2008a; Li et al. 2009; Fortier et al. 2007). The poly(I:C)-induced immune reactions in the maternal host are time-limited, ranging from 24 to 48 h, depending on the precise dose used (Cunningham et al. 2007), thus allowing the experimenter to precisely time the maternal immune response according to specific periods of fetal development. Such experimental investigations seem highly relevant to address some of the ongoing debates as to whether the strength of the association between prenatal maternal infection and enhanced schizophrenia risk is dependent on the precise prenatal timing (Meyer et al. 2007).
The Present, Part 2: Models of Bacterial-Like Immune Activation by Lipopolysaccharide
Maternal administration of the bacterial endotoxin lipopolysaccharide (LPS) is a widely used model system to mimic an innate acute phase response to bacterial infection in the absence of live bacteria exposure. LPS is an inherent cell wall component of gram-negative bacteria, which is recognized mainly by the pathogen recognition receptor transmembrane protein toll-like receptor 4 (Akira and Takeda 2004). Upon binding to toll-like receptor 4, LPS stimulates the expression of a wide array of innate immune responses that include the synthesis and release of various pro-inflammatory cytokines (Akira and Takeda 2004). There are some notable similarities between the cytokine-associated inflammatory responses triggered by LPS and poly(I:C) (Akira and Takeda 2004; Kimura et al. 1994). Therefore, it might not seem surprising that prenatal LPS treatment, similarly to poly(I:C), precipitates a number of behavioral and neurochemical changes relevant to schizophrenia and related psychotic illnesses (Table 2.1).
Despite the apparent similarities between the LPS- and poly(I:C)-induced effects, there are also some noticeable differences between the two models with respect to the nature of brain and behavioral changes. For example, early prenatal poly(I:C) treatment in mice has been shown to increase midbrain dopamine cells (Vuillermot et al. 2010), whereas prenatal LPS exposure leads to the opposite effect (Carvey et al. 2003). Prenatal LPS exposure in rhesus monkey has also been found to cause a significant increase in global white matter volume (Willette et al. 2011), whereas an opposite pattern (i.e., decreased white matter volume) has been noted in rhesus monkey offspring born to influenza-infected mothers (Short et al. 2010). Such differences in the long-term outcomes between prenatal exposures to bacterial-like and viral/viral-like immunogens might be taken to support the idea that different pathogens can induce a distinct set of neuroimmune abnormalities across brain development.
It remains to be determined whether such differences can be explained by the differential immune signatures and pathophysiological responses triggered by different immunogens. Unlike LPS, for example, poly(I:C) is a potent inducer of type I IFNs that stimulate antiviral immune responses (Kimura et al. 1994). By contrast, LPS is more effective in stimulating the production and secretion of TNF-α from innate immune cells such as macrophages (Reimer et al. 2008). The differential effects on TNF-α might be a reason why LPS is more potent than poly(I:C) in triggering anorexia, lethargy, and febrile responses (Hopwood et al. 2009). In view of the strong apoptotic activity of TNF-α (ClarkI 2007), maternal LPS exposure might also be more effective in inducing neurotoxic effects in the fetal brain compared with maternal poly(I:C) treatment (Hagberg et al. 2012). Indeed, maternal or intrauterine exposure to LPS has been widely used as an experimental model system for the induction of perinatal white and gray matter damage in relation to cerebral palsy and other developmentally acquired neurological conditions (Hagberg et al. 2012; Burd et al. 2012; Harvey and Boksa 2012).
The Present, Part 3: Models of Local Inflammation and Exposure to Individual Cytokines
There have been recent attempts to explore whether local maternal inflammation during pregnancy is sufficient to induce long-term brain and behavioral changes in the offspring. One promising model is based on maternal intramuscular injection of turpentine oil (Aguilar-Valles and Luheshi 2011; Aguilar-Valles et al. 2010, 2012). After its intramuscular injection, turpentine remains confined at the site of administration and locally causes tissue damage, recruitment and activation of immune cells, and secretion of pro-inflammatory cytokines (Aguilar-Valles and Luheshi 2011; Aguilar-Valles et al. 2010, 2012). This experimental approach offers the opportunity to study the effects of circulating inflammatory mediators that are solely produced by the maternal immune system. Hence, in contrast to poly(I:C)- or LPS-based models of systemic immune activation (Ashdown et al. 2006; Hsiao and Patterson 2011), placental secretion of inflammatory markers is minimal, and this readily facilitates the delineation of the relative contribution of maternally produced versus placenta-derived inflammatory factors in the link between prenatal inflammation and abnormal brain and behavioral development (Aguilar-Valles and Luheshi 2011; Aguilar-Valles et al. 2010, 2012). Intriguingly, maternal turpentine treatment is effective in inducing long-term behavioral and pharmacological changes in the offspring, some of which are highly similar to the pathologies found after prenatal exposure to other immune-activating agents (Table 2.1). These findings provide further support for the hypothesis that induction of maternal inflammatory responses might be a key factor mediating the association between maternal infection during pregnancy and altered behavioral development in the offspring. Moreover, given that intramuscular injection of turpentine causes localized tissue damage, the findings from this model might be taken to encourage human epidemiological studies exploring potential associations between maternal physical trauma and increased risk of neurodevelopmental disorders in the offspring.
Another valuable approach to delineate the role of cytokine-associated mechanisms is to treat pregnant animals with specific cytokines. This approach has been successfully implemented both in rats and mice (Smith et al. 2007; Samuelsson et al. 2006). The existing data indicate that the inflammatory cytokine IL-6 might be a crucial immunological mediator of the link between maternal immune activation and altered brain development. Indeed, administration of exogenous IL-6 to pregnant animals is sufficient to induce long-lasting structural and functional abnormalities in the adult offspring, some of which are highly comparable to those induced by prenatal exposure to other immune-activating agents such as poly(I:C) (Smith et al. 2007; Samuelsson et al. 2006). Moreover, when IL-6 is eliminated from the maternal immune response by genetic interventions or with IL-6 blocking antibodies, maternal immune challenge by poly(I:C) is no longer efficient in inducing behavioral maldevelopment in the resulting offspring (Smith et al. 2007). Prenatal exposure to other pro-inflammatory cytokines alone, including IL-1β, IFN-γ, or TNF-α, seems to be insufficient to precipitate similar behavioral deficits in the adult animals; and co-administration of soluble IL-1β or IFN-γ receptor antagonist to pregnant dams does not prevent the behavioral deficits caused by prenatal poly(I:C) exposure (Smith et al. 2007).
It remains to be further elucidated why IL-6 might be a key cytokine in mediating the link between prenatal immune challenge and altered brain development. One parsimonious explanation might be that IL-6 is readily capable of crossing the placental barrier, whereas other inflammatory mediators such as IL-1β and TNF-α display only minimal transplacental transfer (Zaretsky et al. 2004). Interestingly, IL-6 exerts some noticeable anti-inflammatory effects in addition to its known pro-inflammatory functions (Xing et al. 1998). The disruption of the balance between pro- and anti-inflammatory signaling in prenatal life might thus represent an important mechanism precipitating changes in brain and behavioral development (Meyer et al. 2008b). Taken together, it seems that the nature and/or severity of neuropathological outcomes after prenatal infection and/or inflammation might be, at least in part, influenced by the specificity of cytokine-associated immunological reactions. Additional research toward this direction is highly warranted, because the identification of specific key mechanisms might offer an effective strategy to attenuate or even prevent the neurodevelopmental sequelae associated with prenatal infection.
The Future: Deconstructing the Phenotypes and Broadening the Concepts
It seems no longer a matter of debate that the etiology of multifaceted neuropsychiatric disorders such as schizophrenia is multifactorial. With the establishment of more sophisticated genetic techniques and epidemiological approaches, the list of potential candidate genetic and environmental risk factors for schizophrenia is constantly rising (Brown 2011; Owen 2012a). Furthermore, it is becoming increasingly evident that seemingly remote disorders such as schizophrenia, autism, attention-deficit/hyperactivity disorder, and major depression share considerable amounts of risk factors and brain dysfunctions (Cheung et al. 2010; Meyer et al. 2011; Smoller et al. 2013; Moreno-De-Luca et al. 2013). The presence of shared genetic and environmental risk between those illnesses has led to the proposal that they might lie along a continuum of genetically and environmentally induced neurodevelopmental causality (Moreno-De-Luca et al. 2013; Owen 2012b). This concept has a number of implications for those of us who are attempting to study disease-relevant neurobiological and behavioral correlates in animal models. First and foremost, animal researchers should not aim for an animal model that could possibly recapitulate the full spectrum of behavioral symptoms associated with a complex brain disorder such as schizophrenia. This is especially true when the model is based on one particular genetic or environmental manipulation only. Such single risk-factor models should rather be expected to induce a restricted set of brain and behavioral changes, and those changes should not be canalized or fitted into one specific neuropsychiatric disease entity (O’Donnell 2011).
Some of these considerations have encouraged animal researchers to develop translational models that incorporate multiple etiologically relevant risk factors (Ayhan et al. 2009; Kas et al. 2011). On the basis of the role of prenatal infection in schizophrenia, there have been recent attempts to explore the cumulative impact of immune-related environmental challenges and distinct genetic abnormalities in the disruption of brain and behavioral development. The rationale for these attempts is given by epidemiological findings suggesting that prenatal infections seem to have rather modest effects in large populations in spite of their relatively frequent occurrence (Selten et al. 2010). It has therefore been proposed that early-life immune challenge might unfold its neuropathological impact primarily in genetically predisposed subjects (Clarke et al. 2009). Another feasible scenario is that initial exposure to a prenatal environmental insult, such as infection, can render the offspring more vulnerable to the pathological effects of a second postnatal stimulus, such as exposure to traumatizing experiences or chronic consumption of drugs of abuse (Maynard et al. 2001).
Rodent prenatal immune activation models seem highly suitable for these kinds of gene–environment or environment–environment interaction studies. With the maternal poly(I:C) administration model in mice, it has recently been shown that the brain and behavioral consequences of (mild and physiologically relevant) prenatal immune activation are markedly exacerbated in offspring with genetic predisposition to neurodevelopmental abnormalities induced by mutant expression of disrupted-in-schizophrenia 1 (Abazyan et al. 2010; Lipina et al. 2013) or by mutations in the dopamine-related transcription factor Nurr1 (Vuillermot et al. 2012). Intriguingly, the combination of such genetic manipulations and prenatal immune activation does not only produce additive effects but further leads to behavioral disturbances that are not manifest after exposure to either manipulation alone (Abazyan et al. 2010; Lipina et al. 2013; Vuillermot et al. 2012). Our laboratory has recently developed an environmental “two-hit” model, in which exposure to physiologically relevant dose of maternal poly(I:C) treatment served as the first hit and exposure to sub-chronic stress in pubescence served as the second hit (Giovanoli et al. 2013). In this model, mild prenatal immune activation and peripubertal stress caused synergistic effects in the development of specific behavioral abnormalities such as sensorimotor gating deficiency and enhanced sensitivity to psychotomimetic drugs (Giovanoli et al. 2013). Neither immune activation alone nor stress alone affected these behavioral functions in adulthood, so that abnormalities in these domains became evident only after combined exposure to the two environmental factors (Giovanoli et al. 2013). Hence, prenatal infection can act as a “disease primer” that increases the vulnerability of the offspring to the detrimental neuropathological effects of other environmental insults such as peripubertal stress.
Taken together, animal models might critically help to identify complex interactions between discrete genetic and environmental risk factors in the development of chronic mental illnesses. These novel approaches hold promise especially for psychiatric disorders with neurodevelopmental components, considering how little we understand about the disruption of brain development induced by combined effects of multiple genetic or environmental adversities.
Concluding Remarks
Modeling the epidemiological association between prenatal immune challenge and altered brain and behavioral development in rodent systems has produced an astonishing amount of experimental data supporting a role of immune-mediated neurodevelopmental abnormalities in major psychiatric illnesses. Many of the models can mimic a broad spectrum of behavioral, cognitive, and pharmacological abnormalities relevant to schizophrenia and beyond. Perhaps one of the most important features of prenatal immune action models is that they are “neurodevelopmental disruption models”: they allow a multifaceted, longitudinal monitoring of the disease process as it unfolds during the course of neurodevelopment from prenatal to adult stages of life. Moreover, they do not rely on any presumption of the neuronal substrates of a specific disorder, and therefore they offer an unbiased way to identify etiopathological processes underlying the changes in neurodevelopmental trajectories and behavioral functions after exposure to prenatal adversities such as infection. Quite surprisingly, however, prenatal immune activation models have thus far largely ignored the (pathological) processes occurring during the early postnatal period. Indeed, the investigation of physiological, behavioral, and neuroanatomical functions at neonatal or early juvenile stages of life is relatively rare in the existing models (Baharnoori et al. 2012; Escobar et al. 2011). It would seem highly important to gain knowledge about possible disturbances in early neurobehavioral functions, because subjects who later go on to develop chronic mental illness such as schizophrenia often show (subtle) cognitive, motor, and social disabilities during the premorbid phase of the disease (Fatemi and Folsom 2009; Tandon et al. 2009).
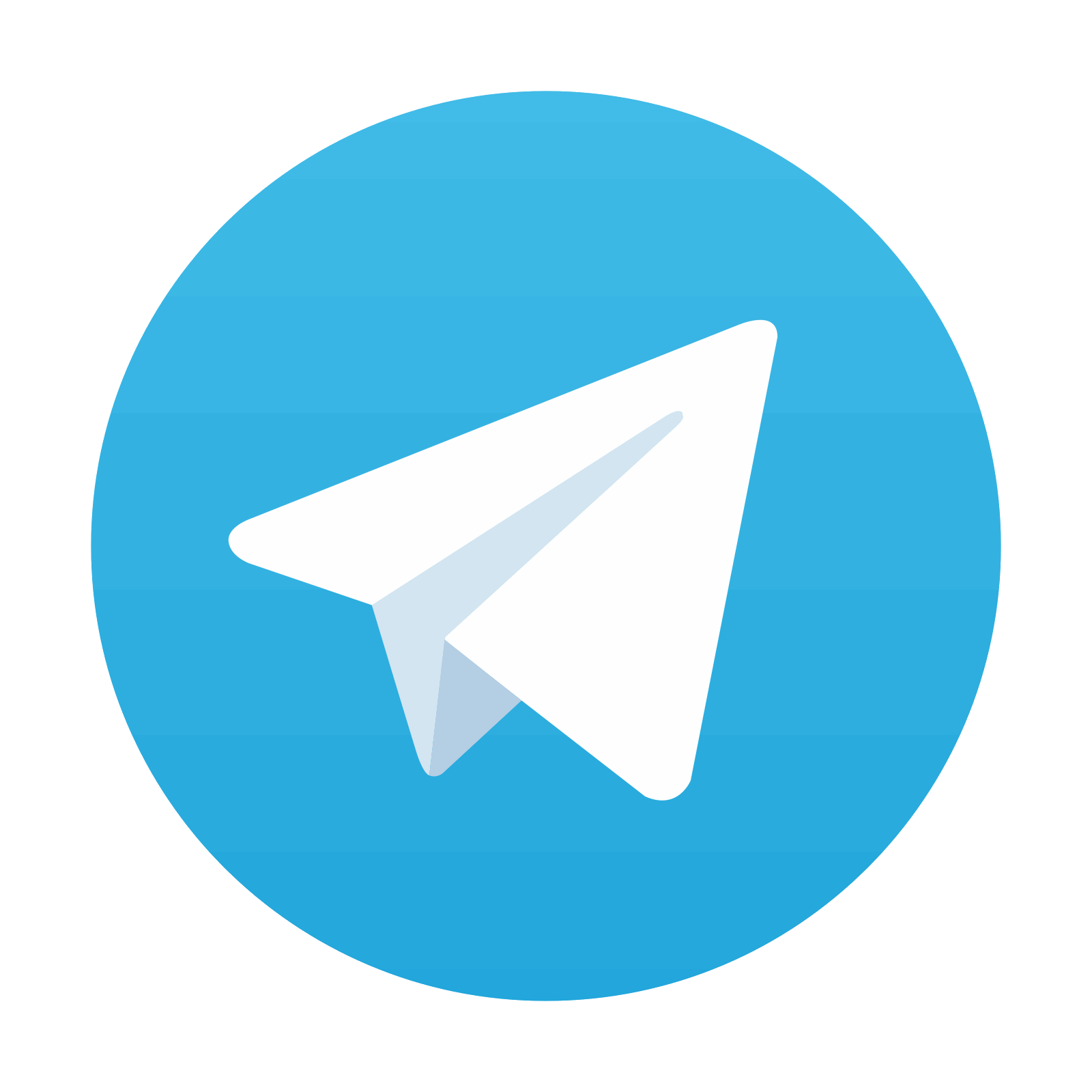
Stay updated, free articles. Join our Telegram channel

Full access? Get Clinical Tree
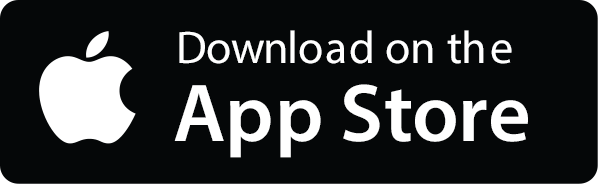
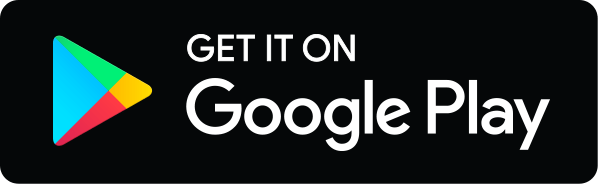