, Marco Cenzato2, Francesco Curto3 and Cristina Motto4
(1)
Department of Neuroscience, Department of Neuroradiology, ASST Grande Ospedale Metropolitano Niguarda, Milan, Italy
(2)
Department of Neuroscience, Department of Neurosurgery, ASST Grande Ospedale Metropolitano Niguarda, Milan, Italy
(3)
Department of Neuroscience, Department of Neuroresuscitation and Intensive Care, ASST Grande Ospedale Metropolitano Niguarda, Milan, Italy
(4)
Department of Neuroscience, Department of Neurology and Stroke Unit, ASST Grande Ospedale Metropolitano Niguarda, Milan, Italy
Hemorrhagic stroke includes spontaneous intracerebral hemorrhage and subarachnoid hemorrhage, and although they represent, respectively, about 15 and 5 % of all strokes, they are an important public health problem throughout the world, due to the elevated rates of mortality and disability, which are higher than in ischemic stroke.
In addition, the global impact of hemorrhagic stroke has significantly increased in recent decades: between 1990 and 2010, there was an increase of 47 % in terms of the absolute number of people affected by the disease. This is mainly due to a significant increase in incidence in low- and middle-income countries (+22 %; 95 % CI 5–30 %), while in high-income countries the incidence has significantly decreased (−19 %; 95 % CI 1–15 %) [1].
According to the same report [1], the prognosis has improved in both high-income and low- and middle-income countries, although in different proportions: reduction in mortality of 38 and 23 %, reduction of Disability-Adjusted Life Years (DALYs) lost 39 and 25 %, and reduction in mortality-to-incidence ratios 27 and 36 %, respectively, probably due to improved disease management.
Hemorrhagic stroke is a medical emergency and must be diagnosed and managed in a timely manner because of the high risk of clinical deterioration in the first hours after the onset of symptoms. Since intracerebral hemorrhage (ICH) and subarachnoid hemorrhage (SAH) recognize different aetiologies and treatments, they will be treated separately.
1.1 Intraparenchymal Brain Hemorrhage
ICH is a focal parenchymal cerebral bleed caused by the rupture of a vessel, resulting in compression and disruption of the brain tissue. It can spread to other cerebral compartments, such as ventricles, rarely the subdural or subarachnoid space.
1.1.1 Epidemiology
The annual incidence of ICH is 24.6 cases per 100,000 inhabitants (95 % CI 19.7–30.7) [2], gradually increasing with age [2–4]. It accounts for 10–15 % of all brain strokes [5], with a variability relating to geographic areas and age. ICH is prevalent in the Asian population where it accounts for 30 % of all strokes [6] and in the age group under 45 years, in which ICH and SAH are 25–55 % of the total number of strokes [7].
1.1.2 Aetiology
ICH can have primary and secondary causes.
Among the primary causes are hypertensive angiopathy and cerebral amyloid angiopathy (CAA).
Hypertensive Angiopathy
High blood pressure is the most important cause of ICH [10] and 50 % of all ICH is caused by hypertension. Hypertensive angiopathy is the predominant cause of ICH also in subjects aged between 40 and 50 years [11]. High blood pressure increases the risk of ICH particularly in patients who do not comply to hypertension therapy and in patients younger than 55 years old who are habitual smokers [12].
By contrast, appropriate control of arterial hypertension reduces the risk of ICH. The majority of ICH related to hypertensive angiopathy occurs because of the rupturing of small perforating arteries such as lenticulostriate, thalamus perforating arteries and the arteries that originate from the basilar artery.
1.1.2.1 Cerebral Amyloid Angiopathy (CAA)
CAA accounts for 5–20 % of the causes of ICH [13, 14] and is typical of the elderly. Amyloid angiopathy is characterized by the progressive deposit of amyloid-beta peptides in the small and medium-diameter capillaries, arterioles and arteries of the cerebral cortex, leptomeninges and cerebellum, causing degenerative alterations that reduce the compliance of the vessel and cause micro-hemorrhages or symptomatic ICH [13, 15].
The location of ICH related to this disease is typically lobar and less frequently cerebellar, rarely deep, and reflects the distribution of pathological changes of microangiopathy. It is a disease that affects the elderly population and is commonly associated with changes in the gene coding for apolipoprotein E [5]. The juvenile familial form is typically associated with mutations in the gene coding for the amyloid precursor protein [16].
Leukoaraiosis and micro-cortical infarcts are associated with CAA and are probably caused by a chronic hypoperfusion of the arteries affected by amyloid angiopathy [13, 17].
Secondary causes of ICH are as follows:
Vascular malformations: arteriovenous malformations (AVMs), dural arteriovenous fistulas DAVFs, aneurysms, cavernous angiomas, venous angiomas, Moyamoya syndrome and telangiectasias
Coagulopathies: secondary to taking anticoagulants, antiplatelets and thrombolytics and congenital and acquired hemorrhagic diathesis (deficiency of coagulation factors, quantitative or qualitative platelet abnormalities)
Exogenous substances (cocaine, amphetamines, alcohol)
Brain tumours and metastases (melanoma, lung cancer, renal cell carcinoma, testicular carcinoma, choriocarcinoma)
Cerebral venous thrombosis
Infectious and inflammatory diseases (septic arteritis, mycotic aneurysms, hemorrhagic encephalitis of Weston-Hurst, vasculitis)
In the elderly, the most frequent causes of ICH are hypertensive angiopathy and amyloid angiopathy, ICH associated with anticoagulant drugs and cancer, while in young people the most frequent causes are vascular malformations, substance abuse and coagulopathy.
1.1.2.2 Location
ICH frequently occurs in the cerebral lobes, basal ganglia, thalamus, brainstem – mostly pons – and cerebellum. Therefore, the locations are defined as lobar, deep, and infratentorial. The deep location is the most frequent and represents about 45 % of all ICH; the lobar location accounts for 30–40 % of all ICH, the cerebellar location 10 % and the brainstem location about 5 %. There may however be differences depending on the characteristics of the study population.
1.1.3 Prognosis
ICH is the type of stroke which leads to higher mortality and disability. 30-day mortality is estimated at between 32 and 50 % [2, 4, 20] and the 1-year survival is 46 % [19]; only 28–35 % of the patients who survive are independent 3 months after the acute event. In ICH patients, the “do not resuscitate” order is quite frequent and reaches 35 % in a recent study [21]. Factors associated with the “do not resuscitate” order are advanced age, ICH severity and early clinical deterioration.
Neurological deterioration commonly occurs in the first hours after onset of symptoms: 20 % of patients present it in the pre-hospital phase; a further 15–23 % of patients show signs of clinical deterioration after arriving at the hospital [22]. Neurological deterioration often signals constant bleeding resulting in expansion of the hematoma.
1.1.3.1 Prognostic Factors
Some of the factors that negatively affect the prognosis have been identified: age, state of consciousness, blood pressure, diabetes, elevated blood glucose on admission, hematoma volume, endoventricular extension of the hemorrhage, perihemorragic oedema, hydrocephalus, concomitant anticoagulant therapy, increased troponin and hyperpyrexia.
Hematoma expansion, intraventricular extension of the hemorrhage with hydrocephalus, hyperglycaemia and perihemorragic oedema are the major predictors of poor outcome in the acute phase of ICH [23].
Hematoma Volume
Among the prognostic factors, the volume of the hematoma is the most significant as it is associated with an increased risk of mortality [24, 25]. A haematoma volume exceeding 50 ml is associated with an unfavourable prognosis. In the first hours after the onset of symptoms, the haematoma can expand as a result of continuous bleeding.
Haematomas generally expand during the first 3 h after onset of symptoms, although a volumetric expansion is possible until 12 h [5]. Increases of various degrees in the volume of hematomas have been observed in up to 73 % of patients evaluated within 3 h of onset of symptoms [26–28]. In more than 35 % of patients struck by ICH, hematomas expanded by more than a third of the initial volume [26].
An algorithm called BRAIN has been proposed and validated in order to predict the risk of ICH growth. This algorithm is scored on 24 points deriving from the INTERACT2 study and based on the volume of the hematoma on the basal patient’s brain CT scan (score per ml of volume <10 = 0, 10–20 = 5>7 = 20), recurrent ICH (yes = 4), anticoagulant therapy with warfarin on onset of symptoms (yes = 6), endoventricular extension (yes = 2) and number of hours from onset of symptoms to brain CT scan (<1 = 5, 1–2 = 4, 2–3 = 3, 3–4 = 2, 4–5 = 1, >5 = 0).
The probability of the hematoma growing varies from 3.4 % for 0 points to 85.8 % for 24 points [29]. This scale is intended to stratify the risk of hematoma growth for clinical and research purposes.
Intraventricular Bleeding in ICH
ICH in the ventricles expands in up to 30–50 % of cases and can be observed in both the early and late phases of the disease [23]. The presence of intraventricular hemorrhage (IVH) bleeding is associated with an unfavourable prognosis [24] and the amount of blood in the ventricles directly correlates with the extent of damage and the probability of survival.
In the INTERACT 2 study, a strong association was observed between the amount of endoventricular bleeding and an unfavourable prognosis, with a cut-off volume of 5–10 ml, above which mortality and disability 90 days after the major event increase significantly [30].
Observational studies have shown that endoventricular administration of thrombolytic facilitates the resolution of intraventricular hemorrhage with or without ICH, reduces intracranial pressure (ICP) and duration of the external ventricular shunt (EVS), and could improve the prognosis for patients.
A clinical randomized and controlled double-blind CLEAR III study (Clot Lysis Evaluation of Accelerated Resolution of Intraventricular Hemorrhage) is currently being carried out in order to assess the effectiveness of the therapy. This study includes patients with IVH, with or without supratentorial ICH with a volume of <30 ml and no underlying vascular abnormalities or coagulopathies, and who require the positioning of EVS. They are randomized to rtPA or placebo [31].
1.1.3.2 Statins
The association between statins and ICH is controversial. A recent meta-analysis suggests that the use of statins before a spontaneous ICH increases neither short-term mortality nor disability [34].
1.1.4 Diagnosis
ICH is a medical emergency and rapid diagnosis of the aetiology of the bleeding is also essential for appropriate management of the patient.
1.1.4.1 Clinical Presentation
ICH presents with acute onset of focal neurologic deficits often accompanied by headache, vomiting and/or alteration of conscious, depending on the location and size of the ICH. Altered consciousness occurs frequently and in a large-scale study only 28 % of patients with ICH had a normal level of consciousness; 30 % of patients were in a coma [35]. The progression of symptoms is observed in 51–63 % of patients, in higher proportions than in ischemic stroke (5–20 %), and it signals an enlargement of the ICH caused by persistent bleeding.
The most frequent clinical features of ICH, such as the onset of headache, impaired consciousness and vomiting, have been used to build diagnostic scales that help distinguish clinically hemorrhagic stroke from ischemic stroke. However, validation studies have not demonstrated a high reliability of ICH diagnosis and clinical data alone is not sufficient to clearly differentiate ischemic from hemorrhagic stroke. The use of neuroimaging is mandatory, given the imperative need to differentiate between the two types of stroke in order to establish the most appropriate treatment.
The clinical severity of the ICH can be assessed using the National Institute Health Stroke Scale (NIHSS), a standardized and reproducible rating scale that retraces the neurological examination and which is commonly used in ischemic stroke. However, the altered consciousness that commonly occurs in ICH means that this scale is not always applicable; in these cases the Glasgow Coma Scale (GCS) is used.
The initial GCS score and the volume of ICH are the best predictors of mortality rate at 1 month from the event [20].
Several prognostic scales have been proposed over time; the most used is the ICH score that takes into account factors that have proven to have a prognostic role: GCS, hematoma volume, presence of endoventricular blood, hematoma location and age (see Appendix) [36]. It is a 5-item scale with a range from 0 (excellent prognosis) to 6 (high probability of death) and with a good degree of correlation with mortality after 30 days and functional prognosis after 1 year [37].
1.1.4.2 Instrumental Diagnosis
Although the sudden onset of symptoms with headache, vomiting, impaired consciousness and rapid deterioration of vigilance may suggest the diagnosis of ICH, the definitive diagnosis is given by computed tomography (CT). The ICH appears on CT as a hyperdense lesion from the outset. The CT scan allows adequate assessment of the location and size of the hematoma and the presence of peri-hematoma oedema and intraventricular bleeding. The examination is rapidly performed and communicated to the emergency departments, allowing an immediate distinction between ischemic stroke and hemorrhagic stroke, thus leading to the most appropriate treatment being delivered in the hyperacute phase.
Magnetic resonance imaging (MRI) with gradient echo sequences can detect hyperacute intracranial hemorrhage with a degree of sensitivity and accuracy that is comparable to CT [38, 39], but this examination is difficult to use in emergency due to the time required, the need for the patient’s cooperation, its poor distribution in emergency areas, as well as its cost.
MRI with susceptibility weighted imaging (SWI), particularly sensitive in highlighting haemoglobin degradation products, is more sensitive than CT scans for detecting microbleeding and previous hemorrhages, as well as detecting structural alterations such as vascular malformations and tumours; it is the preferred choice for diagnosing cavernomas [40].
If an MRI shows findings such as lobar microbleeds, superficial siderosis and white matter hyperintensity, this leads towards the diagnosis of amyloid angiopathy. Therefore, it is a valuable tool for the aetiologic diagnosis of ICH, especially if performed after re-absorption of the hemorrhage.
The multilayer CT angiography (CTA) and CT venography can identify vascular anomalies at the base of ICH, e.g. MAV, aneurysms and venous thromboses. A meta-analysis showed that the diagnostic accuracy of CTA compared to digital angiography (DSA) in ICH is 98.2 %, with a positive predictive value of 97.8 % and negative predictive value of 98.5 %, sensitivity 97.0 % and specificity 98.9 %; the false negative rate was 1 % [41]. The CTA might therefore replace DSA in the initial vascular aetiological diagnosis of ICH in the acute phase.
The contrast-enhanced CT and CTA can highlight the possible presence of a “spot sign”, which was described in 1999 and is defined as a small extravasation of contrast in one or more points of the hematoma. The “spot sign” is visible in about a third of patients with ICH and implies continuous bleeding from the broken vessel. Many single-centre studies have identified the spot sign as a predictor of haematoma expansion in patients undergoing CT within the first few hours of onset of symptoms.
The results were confirmed by the multi-centre observational study PREDICT [42] in which the “spot sign” was observed in 27 % of the 228 patients included in the study and was associated with an increased volume of hematoma and with a deterioration of the patients’ neurological condition. Although the “spot sign” may be a biomarker of enlargement of the hematoma, it should be used with caution.
Cerebral angiography remains the “gold standard” examination for detecting vascular malformations and vasculitis in patients with ICH and should be contemplated in all cases without an obvious cause of ICH and in which previous clinical investigations did not offer a solution. Cerebral angiography is also indicated in people under 55 years with deep ICH [43]. If the results are negative, the vascular diagnostics should be repeated 3–6 months after onset of symptoms.
1.1.4.3 Blood Tests
Blood tests rarely identify the cause of the ICH but can provide indirect information that may contribute to the diagnosis (e.g. inflammatory markers in vasculitis, coagulation abnormalities, abnormal liver enzymes in alcoholics). Blood tests should be complete; on young patients a toxicological examination should be performed to evaluate the possible use of illicit drugs (cocaine, amphetamines, ecstasy).
1.1.5 Treatment of the Acute Phase
Treatment of the acute phase includes general interventions for critically ill patients, such as airway management, and specific treatments for ICH designed to prevent expansion of the hematoma, reduce intracranial hypertension and treat and prevent complications. Treatment of high blood pressure and intracranial hypertension as well as restoration of coagulation should be carried out beforehand in the emergency departments where patients with ICH are evaluated.
The risk of neurological deterioration and cardiac instability is higher in the first 24 h after onset of symptoms; clinical and haemodynamic monitoring should be carried out in intensive/semi-intensive care units. Admission to a stroke unit results in a significant improvement in the prognosis of patients. Furthermore, the mortality rate is significantly lower in patients treated in a stroke unit compared to those treated on a medical ward (risk ratio (RR) 0.73; 95% CI 0.54–0.97, p=0.02) [44].
1.1.5.1 Medical Treatment
Blood Pressure Treatment
Elevated blood pressure (BP) values are reported in approximately 90 % of patients with ICH in the acute phase [45]. A large observational study showed that 75 % of ICH patients had systolic blood pressure >140 mmHg and 20 % of patients had >180 mmHg at onset of symptoms [46]. High blood values may be secondary to pre-existing, poorly controlled hypertension, altered autonomic regulation due to ICH and reaction to the increase in intracranial pressure, pain and neuroendocrine stress response.
Elevated blood pressure values are strongly associated with hematoma expansion, neurological deterioration and poor prognosis, as demonstrated in observational studies [47]. The effect of a rapid lowering of blood pressure in acute ICH patients was evaluated in randomized, controlled clinical trials (ATACH and INTERACT) [48, 49], which showed that aggressive reduction of blood pressure values below 140 mmHg results in a reduced risk of hematoma expansion, in the absence of adverse events. However, the same clinical trials did not show significant differences between the two treatment groups in terms of the 3-month prognosis.
INTERACT-2, a randomized, controlled, open-label study with blind assessment of primary endpoint, included 2839 ICH patients with elevated blood pressure values (systolic BP between 150 and 220 mmHg) who were treated within 6 h of onset of symptoms. This study showed that the intensive reduction of systolic BP with a target of <140 mmHg within 1 h, with a lower limit of 130 mmHg, was effective in improving the 90-day functional prognosis of the patients who had significantly lower modified Rankin scores [50]. Therefore, in acute ICH patients elevated blood pressure values should be intensively reduced until systolic values are <140 mmHg [51].
Acute Haemostatic Treatment
In order to reduce the risk of hematoma expansion and the resulting neurological deterioration, some haemostatic drugs were tested. The recombinant activated factor VII (FVIIa) promotes haemostasis at the site of the bleed and limits the extension of the hematoma. The efficacy of treating patients with acute ICH was tested within 3 h of onset of symptoms in a pilot study, with promising results [52].
Although the phase III study (FAST trial) showed a significant reduction in the risk of hematoma expansion in the group treated with FVIIa at different doses, it did not show any benefits to the prognosis. Mortality and disability at 3 months were higher in the treatment groups (FVIIa 20 ug/kg and 80 ug/kg) compared with the placebo groups, 26 % and 29 % compared to 24 %, respectively [53]. The rate of arterial thrombosis was higher in the group treated with FVIIa at a higher dose compared to the placebo group and the lowest FVII dose.
The lack of effectiveness in the presence of stabilized hematoma expansion would suggest the need for further treatments, including surgery, to be carried out after haemostatic treatment. The analysis of FAST study subgroups suggests potential benefits in patients under 70 years, with a hematoma volume of <60 ml, intraventricular hemorrhage volume of <5 ml and time of treatment <2.5 h from onset of symptoms [53].
Tranexamic acid is currently being tested in primary ICH in the hyperacute phase. The TICH2 study is a multicentre, randomized, controlled, double-blind trial, in which tranexamic acid is administered intravenously (loading dose of 1 g in 10 min + 1 g in 8 h) within 8 h of symptom onset and compared to placebo. The efficacy of treatment is evaluated in terms of mortality and dependency at 3 months.
Coagulation Treatment in Secondary ICH After Anticoagulation Therapy
ICH associated with oral anticoagulant therapies accounts for 12–20 % of the total number [54] and is rising in relation to the increased use of these drugs in the elderly population, who already carry an increased risk of ICH. Most of those on oral anticoagulation therapy take vitamin K antagonist medications such as warfarin, but there is a steady increase in the proportion of patients treated with the new oral anticoagulants dabigatran, rivaroxaban, and apixaban. These drugs are associated with a lower risk of ICH compared to warfarin.
ICH occurring in the course of anticoagulant therapy should be rapidly treated with substances which antagonize the drug taken: protamine sulphate for unfractionated heparin and vitamin K, fresh frozen plasma (FFP) and prothrombin complex concentrate (PCC) if the patient is on an oral anticoagulant therapy with vitamin K antagonists.
The coagulation process should be restored rapidly and preferably within 2 h of onset of symptoms [5, 47]. Vitamin K begins to exert its effect 2 h after administration and reaches its peak after 24 h [55]. Therefore, despite being part of the recoagulation treatment, it is not sufficient for speedy correction of the international normalized ratio (INR).
PCC normalizes INR within a few minutes of administration, but it carries an increased risk (low) of thrombotic events [56]. A randomized and controlled study showed that 4-factor PCC is not less effective than FFP in speedy correction of the INR: INR <1.3 was obtained within 30 min in 62.2 % with PCC and 9.6 % with FFP, the occurrence of thrombotic events was similar (7.8 vs 6.4 %) while fluid overload was greater for FFP (12.8 vs 4.9 %) [57].
The optimal value of INR to be achieved in patients suffering an ICH associated with vitamin K antagonist therapy is not clear, and the targets considered in the various studies are between 1.3 and 1.5 [47]. The recombinant factor VII can quickly normalize INR but it does not restore all vitamin K-dependent factors, so it is not recommended.
As regards the new oral anticoagulants, no substances exist at this time that are able to antagonize the effect of the drug, but specific antidotes are under study. The half-life of these drugs is short and varies from 5 to 15 h. Some studies suggest the use of PCC for inhibitors of activated factor X, rivaroxaban and apixaban [58].
1.1.5.2 Surgical Treatment
A spontaneous cerebral hemorrhage presents two problems that require the involvement of the neurosurgeon:
- 1.
Preventing or treating any secondary damage caused by the bleed itself, due to intracranial hypertension
- 2.
Identifying a possible vascular origin of the bleed and treating it to prevent subsequent bleeding
The presence of blood on the CT scan in an unusual location for hypertensive hematoma (thalamus basal ganglia in elderly patients with hypertension) requires neurosurgical evaluation.
Possible surgical treatment of cerebral hematoma cannot ignore identification of the underlying disease; therefore the most important aim is to understand what has bled.
The lesions that most frequently respond well to neurosurgical treatment are the following:
Vascular Disorders in Need of Neurosurgical Expertise
Aneurysms
Arteriovenous malformations
Cavernous angioma
Dural fistulas
In addition, bleeding can be caused by both intrinsic tumours (gliomas) and extrinsic tumours (metastases more frequently starting from the kidney or prostate).
Finally, there are bleeds that are not due to macroscopic causes but to microscopic vascular lesions and which are typically treated by the neurologist (hypertensive vascular disease, lobar hematoma from amyloid angiopathy); the neurosurgeon must become involved when the size of the bleed may lead to intracranial hypertension.
The subarachnoid location of a bleed typically indicates the presence of an aneurysm, while an intraparenchymal bleed generally suggests the presence of arteriovenous malformations, cavernous angiomas or dural fistulas. However, there are many cases that do not follow this schematism: for example, there may be aneurysms whose dome is in the parenchyma, thus the bleed is substantially intraparenchymal.
The availability of CT angiography in the emergency room greatly facilitates the path towards detection of a hemorrhage: performing a CT scan and CT angiography in the same diagnostic session allows a source of bleeding to be quickly identified or excluded and speeds up the diagnostic and therapeutic pathway.
Spontaneous Intraparenchymal Hematoma
The typical spontaneous intraparenchymal hematoma that is not caused by a macroscopic vascular disease is still a therapeutic problem.
In most cases, intraparenchymal hematomas caused by hypertension expand in the hours after their clinical manifestation and there is a correlation between their extension and blood pressure. There is therefore a fine line between keeping blood pressure high enough to sustain adequate perfusion in the presence of possible intracranial hypertension, and avoiding that blood pressure becomes so high that it encourages the growth of the hematoma.
The general criteria that lead the neurosurgeon to evacuate an intracranial space-occupying process are:
Deterioration of consciousness
Shift of the median line exceeding 5 mm.
Unilateral disappearance of basal cisterns
These general criteria are less reliable in the case of spontaneous intraparenchymal hematomas because extensive literature has demonstrated the usefulness of surgical treatment when the clinical condition of a patient with spontaneous deep hematoma is compromised. It is believed that the very fact of reaching deep regions in the basal nuclei via surgery causes considerable damage to the brain tissue, which is likely to exceed any advantage given by the actual evacuation. Furthermore, patients are often elderly and with multiple comorbid conditions.
A multicentre trial (STITCH I) which was concluded in 2005 did not show any difference between the advantages derived from surgery and from conservative treatment of hematomas deeper than one centimetre from the cortical surface. A second trial (STICH II) [59], which ended in 2013, seemed to suggest a modest advantage derived from the surgical evacuation of lobar hematomas, but not for deep ones. STICH trials refer to traditional surgical techniques, which are certainly invasive. Some publications have highlighted the importance of emptying the hematoma with a minimally invasive endoscopic technique or using catheters and washing with thrombolytic drugs to dissolve the clot.
A multicentre trial (MISTIE III) [60, 61] is currently being carried out: the data seems promising, although it is not at the moment conclusive.
The assessment of a neurosurgeon is therefore necessary if the patient’s clinical condition deteriorates or if the CT scan shows a shift of the midline. However, the criteria are not standardized and coded clinical deterioration is still a parameter that requires clinical assessment regarding the possibility of surgical evacuation.
The situation is completely different if the CT scan shows one of the vascular diseases mentioned above, which require separate, specific treatment.
Cerebral Aneurysm
It should be remembered that an aneurysm can also present with intraparenchymal bleeding and little subarachnoid blood. For treatment, see next chapter.
Arteriovenous Malformations (AVMs)
If radiological diagnostics detect an arteriovenous malformation, bleeding can have a wide range of different causes. AVM is an extremely heterogeneous disease (AVM diameters can vary from a few millimetres to more than ten centimetres; AVMs can be superficial or deep; they can have sharp margins or be diffuse) and it is one of the most complex diseases of neurosurgical interest.
AVMs must be treated by neurosurgical and neuroradiological teams experienced in managing this disease. AVM treatment should be centralized in hospitals with large series because of the wide range of variables that guide the treatment.
After several years in which endovascular treatment was prevalent for AVMs, today surgery is the treatment of choice. Endovascular treatment is reserved for specific cases.
In general the hemorrhage caused by an AVM bleed is not as clinically severe as that caused by the rupture of an aneurysm. Furthermore, the risk of an AVM re-bleeding is lower than that of an aneurysm. For these two reasons, the treatment of an AVM that has bled is rarely considered as an emergency, except in cases of life-threatening bleeding, which rarely have a good outcome.
Given the technical complexity involved in treating an AVM, and if the patient’s clinical condition allows it, surgery is preferable after a few days, when the acute phase is over and when the brain tissue is less oedematous and less tense, making surgical treatment of the malformation technically more manageable. It is rare that patients cannot be transported to a centre where they can be given the best possible treatment.
The diagnosis must be accurate and it is essential to carry out selective angiography to detect the point of possible rupture of the malformation. When the malformation is of such a size that it might reach the ventricle, not infrequently the point of rupture may be due to an aneurysm in a small vessel afferent to the malformation of the same ventricle.
Super-selective angiography, which can only be performed in a highly specialized centre, is needed to detect the point of rupture. In the acute phase, it may be appropriate to treat the site of the rupture intravascularly (if it is detectable on selective angiography) and then secure the malformation.
Today, it is no longer considered suitable to treat AVMs intravascularly with the aim of closing the entire malformation. Since endovascular treatment of AVM can involve a high number of complications, it is no longer considered a first-line treatment.
Nowadays endovascular treatment is limited to:
Closing a possible rupture site (aneurysm on a feeder)
Preparing for surgical treatment
In the second case, the treatment is guided by the neurosurgeon’s request to selectively close some afferences to the AVM, usually the deepest afferences which are furthest from surgical access, so that bleeding can be better controlled during surgery.
In order to ensure the safety of the patient and the success of the treatment, it is essential that the neurosurgeon and the neuroradiologist understand each other and cooperate closely when choosing the endovascular and surgical strategy, which must be defined and coordinated before starting any treatment.
Indications for surgical treatment of an arteriovenous malformation are mainly related to its size and location in relation to the eloquence of the brain area affected by AVM.
The Spetzler and Martin scale score (which is based on three parameters: size, involvement of eloquent area and characteristics of the venous drainage) expresses the surgical risk. There are other more detailed and more precise scales, but the Spetzler and Martin has established itself worldwide for its simplicity and its ability to categorize an extremely heterogeneous disease [62].
Malformations with grade scores four to five (therefore large and in eloquent areas) have an extremely high risk factor during surgery and therefore no surgical solution is considered unless the conditions are life-threatening for the patient. Therapeutic solutions for these malformations have not yet been cleared and defined.
There is general consensus that reducing the volume of the malformation through partial endovascular treatments does not represent a viable therapeutic strategy; rather it increases the risk that the malformation may bleed.
There has recently been investigation into the possibility of treating large malformations with multiple radiosurgery sessions spread over several years, splitting the malformation into various target areas. The results are still preliminary but seem to be encouraging.
In 2013, a randomized multicentre study (ARUBA) [63] investigated the treatment of “unruptured” arteriovenous malformations, as a preventive treatment for bleeding.
This study has been and still is a source of numerous controversies mainly due to the fact that it grouped the possible treatments (surgical, endovascular and radiotherapy) under the single heading of “intervention”, uniting incomplete, partial endovascular treatments or gamma knife treatments for which obliteration time is over 2 years with surgery.
Although this study was published in the prestigious magazine Lancet, its findings are extremely questionable [64–67].
Surgical treatment of AVM must be performed in a specialized centre. The technical difficulty in surgical treatment of arteriovenous malformation is mainly linked to haemostasis of the small blood vessels coming from the white matter, which nourish the malformation.
Malformations normally have some big feeders and some large venous drains which are easily identifiable, but a large number of small vessels exist all around the nidus of the malformation and they are recruited and dilated by the large blood flow from the white matter.
The disproportion between the large flow inside these vessels and their very thin walls makes closure and haemostasis of these vessels very difficult. A crucial component of any neurological damage resulting from surgical treatment of arteriovenous malformations is the need to follow these vessels into the deep white matter to be able to control haemostasis.
The development of new surgical tools like non-stitch bipolar or laser has allowed much more effective control of these vessels and has significantly reduced post-operative morbidity. The surgical strategy consists in firstly identifying, isolating and closing afferent arteries and secondly isolating the AVM nidus from all afferents coming from the white matter and finally closing, blocking off the veins and removing the malformation.
The main risk with surgery is that a residue of the AVM may remain and start bleeding again in the immediate post-operative period. For this reason it is indispensable to carry out angiographic control at the end of the operation so as to exclude the presence of any residue, and any that is found must be removed.
These are long surgical procedures demanding several hours and a lot of patience.
The endovascular option is not contemplated if the arteriovenous malformation bleeds, unless the source of the bleed is clearly identifiable (aneurysm of an afferent vessel or venous ectasia).
The outcome of treating the rupture of an arteriovenous malformation is mainly linked to the patient’s neurological conditions before surgery, which were caused by the bleed.
Because of the peculiar nature of malformations in which the eloquent areas often reorganize around the lesion, most of the neurological deficits caused by bleeding and/or surgery are recovered after some time; therefore evaluation of the outcome cannot be made until 6 months after the event.
Neurological recovery is closely related to the neurological condition on presentation (GCS) and the Spetzler and Martin value of AVM. Overall, only 63 % of patients who have suffered a bleed due to an arteriovenous malformation have a good clinical outcome after 6 months; approximately 10 % of the patients die. Conversely, with malformations which scored I and II on the Spetzler and Martin table, surgical treatment has a good outcome in 96.5 % of the patients.
Bleeding due to an AVM therefore carries significant morbidity and mortality; there are therefore many good reasons for preventive treatment of “unruptured” AVMs.
Endovascular Treatment of Arteriovenous Malformations (AVMs) with Cerebral Hemorrhage
Bleeding caused by an AVM rupture is usually an intraparenchymal hematoma or an intraventricular spillage or a combination of the two. More rarely there is a subarachnoid hemorrhage, usually caused by the rupture of an associated aneurysm.
Unlike treating the rupture of an aneurysm, treating an AVM rupture is not traditionally considered an emergency, as it is believed that the probability of a second episode is more rare (it is estimated at 7–10 % in the first year).
However, we believe that there are some exceptions when the point of rupture is identified (usually represented by a pseudoaneurysm in a small branch relating to the AVM), especially in patients with intraventricular hemorrhage. Therefore, we deem it necessary to carry out an early angiographic study in all cases, especially when the patient’s clinical conditions are severe (small bleeds which are clinically not very symptomatic are more often due to momentary fissuring of a draining vein, at a lower pressure than an arterial afferent).
If a pseudoaneurysm is detected, the lesion is excluded by endovascular treatment.
Procedure: preparation of the coaxial systems is the same as when treating an aneurysm, and it is the same as that used in almost all neurointervention treatments. However, the microcatheter is extremely thin and soft, so it can be transported by the blood stream which tends to drag it up into the branches related to the AVM.
If necessary, a coaxial microguide can be used, which in this case is also extremely soft and thin (about one-sixth of a millimetre). Glue is injected just off the pseudoaneurysm (a few millimetres at most). This liquid immediately polymerizes in contact with blood, and becomes solid.
The adhesive is a cyanoacrylate (n-butyl-cyanoacrylate), quite similar to the instant glue (crazy glue or super glue) commonly used in all homes. If the procedure is successful and eliminates the pseudoaneurysm, we believe the AVM can be considered an unruptured AVM. Therefore, it is possible to proceed with quieter timing, even with stereotactic radiosurgery treatment (gamma knife and cyber knife) when needed or indicated.
Cavernous Angioma
Cavernous angioma is considered a congenital disease, but there are elements that suggest it is an acquired disorder. There is a familial form, in which specific genes have been identified, and a sporadic form where there is no established characteristic chromosomal framework. Furthermore, the familial form often presents multiple cavernous angiomas.
Cavernous angiomas are also called hidden malformations because they are not visible on angiography nor on CT scan when they do not have any calcifications. Therefore, the presence of a cavernous angioma must be suspected when the location of the bleed is not a typical one and the patient is young. MRI is the diagnostic procedure of first choice; T2 sequences detect the characteristic halo of haemosiderin.
These are low-pressure malformations, whose potential bleeding is less detrimental than the bleeding of an aneurysm or an AVM. Bleeding of a cavernous angioma is rarely fatal and rarely leaves serious permanent neurologic consequences because, rather than destroying the nerve fibres in the white matter, low-pressure bleeding dissociates them, and once the hematoma is reabsorbed, most of the symptoms also resolve.
Since cavernous haemangiomas may develop at any site of the central nervous system, the clinical manifestation and the risk of persistent neurological deficits are of course higher when the haemangioma is located (and bleeding) in an eloquent area. Typical examples are cavernous angiomas of the brain stem. The incidence of bleeding of this type of angiomas is higher and their bleeding also has very serious neurological manifestations in the acute phase, although in many cases the outcome may, after some time, not be as serious as one might expect because it is not destructive, but dissociative bleeding.
It is believed that once the angioma has bled, the chances of re-bleeding increase from 0.5 % per year for an “unrupted” cavernoma to 6% per year. Cavernous angiomas on the trunk seem to have a higher incidence of bleeding than in other locations.
Treatment is generally not urgent, since this kind of hematoma is hardly ever life-threatening. The main purpose of surgery is to prevent re-bleeding. It is preferable to wait for neurological conditions to be stabilized and for the oedema associated with the bleed to reduce in size and then intervene after approximately 1 week or 10 days.
The general criteria for removing a cavernous angioma are:
- 1.
It has bled.
- 2.
It is surgically accessible.
Evaluating whether a cavernous angioma is surgically accessible in deep locations like the basal ganglia or brain stem depends on the experience of the surgeon [68]. Such operations are extremely complex and must be performed in highly specialized centres; it is not uncommon that what is judged as not surgically treatable in one hospital might be considered treatable in another one.
Surgery is feasible even on deep haemangiomas, but it requires experience and accurate intraoperative neurophysiological monitoring. This type of surgery is not performed in all neurosurgery centres. Surgery is to be recommended in experienced centres in order to avoid new episodes with potentially more severe outcomes.
It is advisable to avoid waiting too long (months) after the bleed because it is easier to operate when the hematoma is still fresh, when the cleavage plane is still preserved and before scar tissue forms, making removal of the cavernoma from the surrounding parenchyma more difficult. Given the location in the brain stem, it is of vital importance not to damage the surrounding tissue.
On the contrary, for supratentorial cavernous angiomas, which are in non-critical locations, the removal of a margin of surrounding tissue, corresponding to a haemosiderin flange visible on the MRI, helps reduce the risk of epilepsy in the post-operative period.
Dural Arteriovenous Fistulas (DAVFs)
The dural fistula is an acquired disorder which is believed to develop as a result of a focal inflammatory process, which generates a shunt between a meningeal artery and a pial vein. The process occurs in relation to the dural venous sinuses.
The presence of a direct shunt between artery and vein creates venous hypertension which may, depending on the structural characteristics of the venous drainage, carry a more or less severe risk of bleeding.
Symptoms can range from the subjective perception of a blow to cognitive impairment related to intracranial hypertension. Symptoms can typically evolve over years. Different rating scales have quantified the risk of bleeding from this pathology.
The Cognard scale [69] is the simplest one. It distinguishes 4 grades depending on the venous discharge, which might be:
- 1.
In a dural sinus with physiological flow
- 2.
In a dural sinus, partially thrombosed with retrograde flow into the pial veins
- 3.
In a vein without dural discharge in dural sinus
- 4.
In a dural vein that presents ectasia
The risk of bleeding increases with the score on the scale and reaches 40 % a year for grade 4. Venous engorgement or venous ectasia is the cause of the bleeding.
Extended dural fistulas may cause venous engorgement so as to generate intracranial hypertension, even without bleeding, causing progressive cognitive impairment.
Increased knowledge of the structure of dural fistulas led to the realisation that treatment of a dural fistula simply required closure of the venous shunt. The simple deafferentiation from the arterial side is inevitably followed by relapse. For many years this problem was treated surgically (technically simple surgery), but since the introduction of Onix, the treatment has essentially been endovascular.
It is essential that Onix goes beyond the site of the fistula and reaches and closes the venous side of the fistula. Treating fistulas types 1 and 2 (sinus) still causes difficulty when they affect a nonexpendable sinus. Endovascular techniques with Onix and balloon allow their evolution to be controlled.
Surgical treatment with simple interruption of vein drainage is limited to cases where endovascular access does not allow the point of the fistula to be reached safely or as a result of incomplete endovascular treatments that preclude a subsequent endovascular approach [70].
Endovascular Treatment of Dural Arteriovenous Fistulas (DAVFs) with Cerebral Hemorrhage
DAVFs may result in intracranial hemorrhage when the fistula drainage vein is a cerebral vein (unlike a dural sinus) and bursts open because of the excessive arterial flow inside it.
Please note that a DAVF is a pathological arteriovenous passageway localized on the surface of a dura, almost always on the wall of a dural sinus. Arterial flow directly into the sinus results in sinus DAVF, which, depending on the severity, could cause simple hearing disorders or determine mental decline up to stupor and coma because of the progressive dysfunction of the whole intracranial venous system.
If the arterial flow enters a cerebral vein (or cerebellar) at its intersection with the sinus, but without being able to continue to the sinus, a countercurrent flow is generated in the cerebral vein. The vein can undergo progressive expansion up to a potential rupture.
As in all cases of intracranial hemorrhage after diagnosis via angio-CT and/or angiography, the lesion can be treated with an endovascular procedure. It is possible to heal a DAVF with drainage in a cerebral vein by occluding the first segment of the vein (the “foot” of the vein).
The procedure involves catheterization of arteries which are afferent to the fistula (usually a meningeal branch of external carotid artery) with microcatheters similar to those already described, up to a few millimetres from the site of the fistula.
A liquid material is then injected. Similar to glue but without its adhesive characteristics, it is a solution in which the solvent is dimethylsulfoxide (DMSO), which immediately and rapidly evaporates on contact with blood. The solute then precipitates and solidifies, occluding the vessel into which it is released.
The probability of success offered by this method is very high, exceeding 90 %. There may be some possible complications related mostly to thrombosis of the venous components even at some distance from the fistula.
1.1.6 Complications
1.1.6.1 Seizures
Seizures were observed in 4.2–20 % of ICH patients [23], with a risk of 8.1 % within 30 days after the onset of ICH. The ICH lobar location is the one most frequently associated with epilepsy [71].
Seizures should be treated immediately if they occur as they can worsen the prognosis, but there is no sure evidence to support the preventive use of antiepileptic drugs in ICH patients. Some studies indicate that antiepileptic therapy may be suspended 1 month after seizure, subject to an EEG examination.
1.1.6.2 Fever
Fever is an independent negative prognostic factor in ICH patients who survive the first 72 h [72], but there is no evidence that drug treatment improves the prognosis [73].
The external ventricular derivation catheters should be removed within 7 days because of the risk of infection.
1.1.6.3 Treatment of Hypoglycaemia
Elevated blood glucose values on admittance may be due to diabetes which is known or not properly understood or to stress-related hyperglycaemia, and they are associated with a significant increase in mortality. A study carried out on 992 ICH patients showed that hyperglycaemia on admittance (>9.2 mmol/L) in patients without diabetes resulted in a mortality rate four times higher than the mortality rate in patients with normal blood glucose levels (<5.7 mmol/L) [74]. The optimum glucose target is unclear.
1.1.6.4 Deep Vein Thrombosis (DVT)
1.1.6.5 Indications for Monitoring Intracranial Pressure (ICP) in Hemorrhagic Stroke
The indication for monitoring intracranial pressure by means of intraparenchymal catheter or ventricular shunt is linked to the patient’s clinical condition (GCS <8), mass effect of the bleed and evidence of cerebral oedema on brain CT scan [76].
Since there are no randomized studies on the monitoring and treatment of intracranial pressure in these patients, the indication for invasive monitoring, thresholds of intracranial pressure (<20 mmHg) and cerebral perfusion pressure (50–70 mmHg) are taken from studies carried out on traumatic brain injury (TBI) patients.
A limited number of cases show a possible role of elevated ICP in determining outcomes [77–79]. The catheter or ventricular shunt can be used in cases of acute obstructive hydrocephalus and IVH to drain blood from the ventricular system. In the latter case it can be used to administer fibrinolytic drugs directly into liquor [80]. Both acute hydrocephalus and the presence of intraventricular blood are in fact associated with a worse outcome [81, 82].
1.2 Subarachnoid Hemorrhage
Subarachnoid hemorrhage (SAH) is a spontaneous extravasation of blood into the subarachnoid space which occurs in the absence of trauma. It accounts for about 5 % of all strokes. This disease mainly affects young people and it carries high mortality and disability rates [83], so that the social impact and loss of years of productive life are comparable to that resulting from cerebral hemorrhage and ischemic stroke [84].
Therefore, although it represents a small percentage of the total number of strokes, SAH is a major disease and a medical emergency, and it is a diagnostic and therapeutic challenge in emergency departments.
1.2.1 Epidemiology
The incidence of SAH is approximately 10 cases per 100,000 people per year with a range from 2 to 27 cases per 100,000 per year linked to the geographic areas under consideration. SAH is more common in Japan and Finland (22.7 and 19.7 cases per 100,000/year, respectively) and least frequent in China (2 cases per 100,000/year) and in Central and South America (4.2 cases per 100,000). In the United States and in Italy, the incidence of the disease is 9.7 and 10.8 cases per 100,000 per year, respectively [85, 86]. It is higher in black and Hispanic people than in white Americans [86].
In recent decades there has been a reduction of 0.6 % in the incidence of total SAHs, smaller than that observed for stroke in general [85].
The average age of SAH patients is lower than the age of patients suffering other types of stroke and, although the incidence increases with age, approximately 50 % of SAH patients are younger than 55 years [87]. In recent decades an increase has been observed in the average age of patients, from 52 to 62 years [88].
1.2.2 Aetiology
In 85 % of cases, SAH is secondary to spontaneous rupture of a cerebral aneurysm; in 10 % of cases it is an idiopathic SAH, frequently located in the perimesencephalic area; in 5 % of cases it is due to rarer causes such as arterial dissection, arteriovenous malformations, dural arteriovenous fistulas, mycotic aneurysms, fusiform aneurysms, cerebral amyloid angiopathy, reversible vasoconstriction syndrome and vascular lesions in the spinal cord.
1.2.3 Risk Factors
The modifiable risk factors include active and passive cigarette smoking [89], high blood pressure and high alcohol consumption. Each of them doubles the risk of aneurysmal SAH (aSAH) [90]. Another risk factor is represented by the abuse of sympathomimetic substances (cocaine).
The non-modifiable aSAH risk factors are family history, understood as a first-degree relative having suffered an SAH, genetic diseases such as polycystic kidney disease with dominant autosomal inheritance, Ehlers-Danlos syndrome, deficiency of alpha-1-antitrypsin and female gender.
The risk of aSAH in women varies with age of menarche, pregnancies and menopause. Women who have had an early menarche, women who are going through menopause and first-time mothers have a higher risk of aSAH than other women. There is no evidence of increased risk in pregnancy, childbirth and the postpartum period [91, 92].
People with a family history of SAH tend to be younger than those with sporadic SAH and have larger and multiple aneurysms [93, 94].
The risk of SAH increases in the presence of large unruptured aneurysms of the posterior circulation, particularly if symptomatic, and in the presence of previous SAH with or without untreated residual aneurysm, as demonstrated by the long-term follow-up of ISAT study [95].
The International Study of Unruptured Intracranial Aneurysms (ISUIA) study has provided information on the risk of aneurysm rupture in relation to the location and size of the aneurysm. It is higher for aneurysms of the anterior communicating artery and of the basilar artery apex as compared to the middle cerebral artery.
For aneurysms with a diameter of less than 7 mm, the risk of rupture is low (approximately 0.1 % per year) and progressively increases as the size increases. In the study, 75 % of unruptured aneurysms had a diameter of less than 1 cm. Larger aneurysms (>8 mm) longitudinally followed by MRI tend to grow more over time and therefore they are at greater risk of rupture [96].
Also, the morphological characteristics of the aneurysm, such as a bottle-shaped neck and the relationship between the size of the aneurysm and the afferent vessel, are associated with the risk of rupture [97–99] but it is still unclear how to decline such information for individual patients to predict the risk of SAH.
A model has recently been developed derived from the analysis of six cohort studies, which has led to the creation of a map of the risk of rupture of an intracranial aneurysm [100]. The identified predictors of aneurysm rupture are age, high blood pressure, history of SAH, size of aneurysm, location of aneurysm and geographic area. They form the PHASES score which corresponds to a precise risk of rupture at 5 years [100]. According to this score, the risk of rupture of an aneurysm at 5 years varies from 0.25 % in patients below the age of 70 without vascular risk factors and with small aneurysms of the internal carotid artery (<7 mm) to over 15 % in individuals older than 70 with hypertension, a history of SAH and giant aneurysms (<20 mm) of the posterior circulation. In the Finnish and Japanese population, the risk increases by 6.3 and 8.2 times, respectively.
Some studies have demonstrated a connection between diet and SAH. In particular, high consumption of vegetables is associated with a reduced risk of aSAH [101].
The factors that precipitate the rupture of an aneurysm have not yet been clarified, but it is assumed that a sudden increase in transmural pressure can play a role. Some studies have found that activities such as physical exercise, sexual activity and stress precede the occurrence of aSAH in over 20 % of the cases; no triggers have been identified for the remaining 80 % of the cases [89, 102, 103].
1.2.4 Prognosis
The prognosis is severe and the death rate, although it has decreased by 17 % in recent decades thanks to improvements in the management of SAH in hospitalized patients [88, 104], still remains high, reaching 45 % at 30 days in some studies [105].
A meta-analysis of population studies showed a geographical variation in median mortality rate, significantly lower in Japan (27 %) as compared to Europe (44 %), the USA (32 %), Asia excluding Japan (38 %), Australia and New Zealand [88].
In population studies, 12–15 % of SAH patients die before reaching the hospital (at home or during transportation) and 25 % of the remaining patients die within the first two weeks after the event [88, 106].
More than a third of the survivors remain disabled and typically some cognitive deficits are observed, such as impaired memory, executive functioning and attention disorders that impact on daily life [83]. Cognitive deficits tend to improve during the first year; however, they persist in approximately 20 % of patients [107], leading to a low quality of life.
Nineteen percent of patients show residual disability in conditions of dependence 3–12 months after the event [88].
Deterioration of the clinical condition is due to the initial severity of the bleed and/or to the onset of complications such as re-bleeding and late ischemia.
Re-bleeding can also occur very early. In 15 % of cases early clinical deterioration is caused just by re-bleeding that occurred before the first CT scan or before the patient entered the hospital, and it accounts for pre-hospital mortality.
The risk of re-bleeding is higher in the first 24 h after the aSAH, with a peak in the first 6 h; [108] after the first day, the risk of untreated aSAH remains high in the following 4 weeks, reaching 30 % in a month and decreasing gradually from 1 to 2 % per day to around 3 % per year [109]. The prognosis after re-bleeding is severe, with 60 % mortality rate and residual disability in 30 % of cases [110].
Delayed cerebral ischemia occurs in about one third of cases, mainly in the first and second week after the aSAH (peak 4–12 days). About 25 % of patients die and 10 % are disabled.
Negative prognostic factors include advanced age, clinical severity at admission and the extent of bleeding visible on the CT scan.
Other negative prognostic factors are: ruptured aneurysms of the posterior circulation, large diameter aneurysm and more recently the Apo E lipoprotein allele ɛ4 [111, 112]. Clinical severity and in particular the level of consciousness are the most important prognostic factors.
1.2.4.1 Perimesencephalic Nonaneurysmal Hemorrhage
This type of SAH is confined to perimesencephalic cisterns, generally in the anterior portion of the midbrain and the pons, although sometimes it may be limited to the quadrigeminal cistern [113, 114]. The condition is defined by the characteristic distribution of subarachnoid bleeding and by a normal angiography, necessary for excluding the presence of an aneurysm.
The prognosis of perimesencephalic SAH is good, there is no risk of re-bleeding in the short and long term and the only complication that may occur is hydrocephalus [113].
Perimesencephalic SAH also presents with severe headache but the onset of symptoms is more gradual than in aneurysmal SAH (peaking in minutes rather than seconds). The clinical condition of patients are milder, they sometimes appear slightly disoriented but there are no changes in vigilance [115, 116].
The cause of bleeding is not known, but it is assumed to be of venous origin. Milder symptoms, less rapid onset of headache, limited bleeding and absence of aneurysms support this hypothesis [113].
1.2.5 Clinical Presentation
Spontaneous SAH typically manifests with an unusually severe headache that is generally widespread and has a sudden onset. The so-called thunderclap headache is often described as “an explosion” because of the intensity and speed of onset of pain, with peak time from a fraction of a second to a few seconds in 75 % of cases [116]. However, what should raise the suspicion of SAH is not only the intensity of the pain but its mode of onset. The headache might be associated with an altered state of consciousness, focal neurological deficits and vomiting, but in a third of all cases, the headache is the only symptom. In up to 77 % of cases, the headache is accompanied by photophobia, nausea and vomiting [117]. These are not SAH-distinctive characteristics because they are present in about half of patients suffering from thunderclap headache which is not associated with SAH [116].
Impaired state of consciousness is frequent. It was observed in 53 % of 109 patients in a retrospective study [118] and in about two-thirds of 346 patients on arrival at the hospital in a prospective study; approximately half of them were in a coma [119]. The normal state of consciousness may be recovered or it may remain altered.
Rarely, the patient with SAH may present with an acute confusional state (1–2 % of cases) [120].
Focal neurologic deficits are detectable in 10 % of cases and are due either to the extension of the bleeding in the brain parenchyma or to focal cerebral ischemia which is secondary to acute vasoconstriction occurring immediately after rupture of the aneurysm.
The deficits vary in relation to the bleeding site. The complete or partial deficit of the third cranial nerve has a localizing value because it is the sign of a ruptured aneurysm typically located at the level of the internal carotid artery, at the origin of the posterior communicating artery. The deficit of the sixth cranial nerve, when present, has no localizing value.
A stiff neck is a common sign and is due to an inflammatory response to the blood present in the subarachnoid space. It does not appear immediately after the bleed but needs 3–12 h to occur and cannot be present in patients in deep coma and with negligible SAH [121]. Therefore, the non-accrual of neck stiffness does not exclude SAH.
Seizures on onset have been reported in 7 % of SAH patients [116, 122, 123] and are strongly indicative of SAH caused by aneurysm rupture, since they have not been described in patients with perimesencephalic SAH or in patients with thunderclap headache without bleeding [116].
It should be remembered that although the typical presentation has been described, SAH patients may show isolated headaches and a normal neurological examination. Presentation and severity of the headaches can vary, which may make diagnosis difficult [124].
Some studies have shown that 10–43 % of patients diagnosed with SAH presented a so-called “sentinel” headache from 2 to 8 weeks before the major bleed [125, 126]. Such headache is often milder than that which occurs with the major rupture and may persist for days; it may be associated with nausea and vomiting, but meningeal signs are rarely present. In the absence of a proper diagnosis, a major re-bleed is very frequent in the following days or weeks.
Therefore, it is necessary to maintain a high degree of clinical suspicion for the disease, which should be excluded by appropriate diagnostics, since a missed diagnosis is associated with mortality and disability rates at 1 year that are four times higher in patients with minimal or no neurological signs at the initial examination [124]. The necessary examinations must be carried out on all patients struck by an unusual headache with acute onset and/or unusual pain characteristics.
It should not be forgotten that SAH accounts for only about 1–3 % of all visits to the emergency room for acute headache [127].
The headache lasts 1–2 weeks, though sometimes it can last longer; the minimum duration of the headache is unknown [113].
SAH patients may present intraocular hemorrhages of all types: retinal, subhyaloid or vitreous. In a systematic review vitreous hemorrhage was observed in 13 % of aSAH patients and was more frequent in subjects with an impaired state of consciousness [128]. Intraocular hemorrhage is due to increased intracranial pressure that causes obstruction of the venous drainage of the central retinal vein when it crosses the optic nerve sheath. Linear or flame-shaped subhyaloid bleeds are visible in the vicinity of the optical disc and may extend into the vitreous to configure Terson’s syndrome. Patients may complain of a brown stain that obscures the view. The examination of the fundus oculi is essential in these patients.
SAH clinical severity is expressed by means of rating scales.
1.2.6 Acute-Phase Management
Pre-hospital phase management should include vital sign detection, assessment of the level of consciousness, including determination of the Glasgow Coma Scale (GCS) score, and a brief neurological assessment to detect focal neurological deficits by means of a formal scale in order to assess the severity of the symptoms. The patient must be stabilized, and, depending on the patient’s clinical condition, airway management must be performed.
Cardiac arrest occurs in 3 % of cases and it is important to perform cardiopulmonary resuscitation (CPR) because half of the survivors recover their independence [129].
1.2.7 Assessment in Emergency
A typical candidate for SAH diagnostic protocol is a patient presenting with severe headache of sudden onset. The headache is typically described by the patients as the worst of their lives, with a peak time of seconds and associated with nausea, vomiting and focal deficits. Most severe headaches have a benign cause; approximately 10–16 % are caused by a serious medical condition, including SAH. It is more difficult to decide when to be alarmed in subjects with a vague modification of the primary headache they suffer from and with a normal neurological examination.
Patients presenting with acute headache of sudden onset should undergo brain CT scan if any of these conditions are present: age >= 40, neck pain or stiffness, witnessed loss of consciousness, onset during coitus, thunderclap headache and neck stiffness at neurological examination. This decisional scheme has a 100 % sensitivity for diagnosis of SAH [130].
1.2.8 Instrumental Diagnostics
In case of suspected SAH, a brain CT scan without contrast is the first examination that must be performed, but CT scans have some limitations, related mainly to the time when the examination is carried out and the amount of bleeding. A CT scan guarantees a high level of sensitivity (95–99 %) in detecting blood in the subarachnoid space within the first few hours of onset of symptoms, i.e. in the first 24 h; such sensitivity progressively reduces as time goes by (over the following days) because of the degradation of the blood and its dilution secondary to the continuous circulation of cerebrospinal fluid [131–134].
Within the first 6 h from onset of headache in patients with suspected SAH, the sensitivity of a CT scan is 98.5 % (95 % CI 92.1–100 %) [135]. The predictive negative value of CT results is almost 100 % (95 % CI 99.5–100 %) if the exam is carried out with third-generation CT scans and interpreted by neuroradiologists or radiologists experienced in reading Brain CT scan images [134]. This result was confirmed by a recent retrospective study in non-academic centres (negative predictive value 99.9 %; 95 % CI 99.3–100.0 %) [136]. Over the 6 h following onset of headache, sensitivity is reduced to 90.0 % (95 % CI 76.3–97.2) [135].
MRI might have the same sensitivity as CT in detecting SAHs in the first two days after the event [38] but may be more sensitive in the following days when the hyperdensity on a CT scan is reduced. The use of sequences with fluid-attenuated inversion recovery (FLAIR) and SWI is particularly sensitive to paramagnetic substances such as haemoglobin degradation products [137].
If CT scan findings are negative and clinical suspicion is high, a lumbar puncture should be performed, taking into account that in only 3 % of patients with negative CT findings within 12 h of onset of symptoms, a lumbar puncture shows haemoglobin metabolites and an angiography confirms the presence of a ruptured aneurysm [132]. Account must also be taken in the negative predictive value of a third-generation CT carried out within 6 h of the onset of symptoms.
A lumbar puncture should be performed at least 6 h after the onset of symptoms and preferably 12 h afterwards. In fact, if the cerebrospinal fluid (CSF) is obtained early and contains blood, it is almost impossible to discriminate between blood actually present in the subarachnoid space and blood generated by a traumatic puncture.
If correct timing is observed, blood degradation products will be present only in the case of a hemorrhage; in particular there will be bilirubin resulting from fragmentation of erythrocytes in CSF [113, 138]. The three-tube test carried out in order to differentiate the presence of blood resulting from a traumatic puncture from the presence of blood in subarachnoid space is not reliable [139].
There are specific technical recommendations for CSF analysis when SAH is suspected, and the recommended method is spectrophotometry with quantitative analysis of bilirubin.
An increase of bilirubin in the CSF indicates the presence of blood in the subarachnoid space and excludes that it is due to a traumatic puncture. The increase in bilirubin is generally accompanied by the presence of oxyhaemoglobin; the isolated presence of oxyhaemoglobin is almost always an artefact but may occasionally occur in SAH [140].
The absence of oxyhaemoglobin and bilirubin does not support diagnosis of SAH. It is not advisable to proceed directly to a vascular imaging exam (CT angiography or MRA) before demonstrating the presence of SAH, as unruptured aneurysms with diameters of <5 mm could be detected, which are to be considered incidental findings [113].
A recent study has shown that CT scans and CT angiograms detect aneurysmal SAH in 99 % of cases [141] and lumbar puncture remains the correct approach.
Bleeding resulting in a ruptured aneurysm cannot be confined to the subarachnoid space and may extend to the brain parenchyma, the ventricular system and the subdural space.
An intraparenchymal hematoma has a localizing value for the ruptured aneurysm: frontal or fronto-basal hematomas in anterior communicating artery aneurysms, temporal area hematomas for posterior communicating aneurysms and hematomas in situ for middle cerebral artery aneurysm. The possibility that an intraparenchymal hematoma is secondary to the rupture of a cerebral aneurysm varies from 4 to 35 % of cases.
1.2.8.1 Identifying Location of the Bleed
Digital angiography has always been considered the gold standard for identifying the aneurysmal site of a bleed. Currently CT angiography has become the rule even if it might not identify small aneurysms. The sensitivity and specificity of CT angiography depend on the execution method and on the experience of the professional who interprets it, being, respectively, 90–97 % and 93–100 % [142–144].
The advantages of CT angiography are linked to the fact that it can be performed quickly and immediately after a CT scan which shows the presence of SAH. In many patients, the decision regarding the type of treatment of the aneurysm – endovascular or surgical – is made on the basis of CT findings. Most unidentified aneurysms on CT angiography have a diameter of less than 4 mm [145].
CT angiography and MRA angiography have the same sensitivity for aneurysms. MRA is used to study unruptured aneurysms and for screening patients at high risk of aneurysm, since it does not involve using radiation and contrast medium; vice versa, it is rarely used in the acute phase when the patient may not be very cooperative. MRA has two disadvantages: it requires more time than CT angiography and it is rarely found in emergency departments.
In patients with SAH, cerebral angiography is indicated even if no aneurysm has been revealed in a noninvasive examination. It is used also to plan the type of aneurysm treatment (embolization or microsurgery).
If cerebral angiography findings are negative, the exam should be repeated a week after symptom onset, as it could detect aneurysms which went undetected in the acute phase because of thrombosis of the aneurysm or immediate destruction of the aneurysm at the moment of rupture (when they are small) or local or widespread vasospasm. In 1–2 % of cases, aneurysms are detected in the second angiography exam.
If the second angiography findings are negative, the exam should be repeated after 1–3 months. Cerebral angiography is an invasive examination and it is not risk-free; the risk of temporary or permanent neurological complications in SAH patients is 1.8 % and the risk of re-bleeding of the aneurysm during the exam is quite low [146].
It should be remembered that cerebral angiography, in the case of SAH which is compatible with the rupture of an aneurysm of the posterior circulation, must include the study of both vertebral arteries since aneurysms which are localized on the posterior-inferior cerebellar artery or other proximal branches of the vertebral artery might not be highlighted if only one vertebral artery is studied.
In 10–15 % of cases, the causes of bleeding are not identified. Such SAHs are defined as SAH sine materia or more properly as SAH with negative angiography. Most of these cases fall within the category of nonaneurysmal perimesencephalic SAH of venous origin.
If the angiography is negative in the acute phase, an MRI should be performed to exclude a cervical disease that might have originated the SAH [147].
1.2.9 Rating Scales
As previously mentioned, neurological severity on admission, age, amount of bleeding and endoventricular extension of bleeding are prognostic factors for outcome after SAH.
Among these factors, the neurological status of the patient on admission is the most important and can vary over time. In order to have standardized assessment, it is necessary to have a valid and reproducible assessment tool, having good correlation with prognosis and good interobserver agreement.
Three rating scales are basically used to assess SAH: the Hunt and Hess scale, the World Federation of Neurological Surgeons (WFNS) scale and the modified Fisher scale (see Appendix). These scales have been created for different reasons, none of which are strongly related to the prognosis.
The Hunt and Hess (HH) scale was created in 1968 to stratify patients in relation to the surgical risk [148] and is still widely used. It takes into account the level of consciousness, intensity of headache, neck stiffness and severity of focal deficits. The categories are not clearly defined, however, and the scale has low levels of reproducibility and validity [149, 150].
The World Federation of Neurological Surgeons scale was proposed in 1988 by a commission of the WFNS in an attempt to identify a valid and reproducible tool [151]. It is based on the Glasgow Coma Scale (GCS), which has the advantage of having good interobserver agreement [152–154], and a grade has been added to levels 13 and 14 to insert the presence or absence of focal neurological deficits. The scale is easy to use but the correlation between each grade and the prognosis is controversial, and the cut-offs within the scale are based on consensus and not on a formal assessment.
Another scale was later proposed, based solely on GCS: the Prognosis on Admission of Aneurysmal Subarachnoid Hemorrhage (PAASH) Scale [155]. This scale is divided into five categories and the cut-offs between them are different from the WFNS cut-offs. In fact, the PAASH cut-offs have been selected by calculating the point at which two consecutive categories correspond to a statistically different prognosis at 6 months. This scale has good internal and external validity [156].
In the HH, WFNS and PAASH scales, the higher the score, the worse the patient’s prognosis. A study which evaluated the prognostic accuracy of the three scales showed that the WFNS and PAASH scales have a good prognostic value. The PAASH scale seems to have a more linear and gradual correlation with the risk of poor prognosis in the higher categories than the WFNS scale, and every increase in grade relates to a poorer prognosis [156] (See Appendix).
The interobserver agreement is similar in the WFNS and PAASH scales (weighted kappa values: 0.60, 95 % CI 0.48–0.73, and 0.64, 95 % CI 0.49–0.79, respectively), while it is lower for the HH scale (weighed kappa values: 0.48, 95 % CI 0.36–0.59) [157].
The modified Fisher scale is based on CT findings and derives from the original Fisher scale. It was developed in order to better predict the risk of angiographic vasospasm. The correlation is linear: the higher the score, the higher the risk of angiographic vasospasm [158, 159] (See Appendix).
Another scale has recently been proposed to stratify the risk of cerebral ischemia: the VASOGRADE scale. It combines the WFNS scale and the Modified Fisher Scale (VASOGRADE green, grade 1 or 2 on the modified Fisher scale and WFNS 1 or 2; VASOGRADE yellow, grade 3 or 4 on Modified Fisher Scale and WFNS 1, 2, or 3; VASOGRADE red, WFNS 4 or 5) [160].
1.2.10 Treatment of the Acute Phase and Complications
Emergency treatment includes airway management, close haemodynamic monitoring, support treatment, prevention and treatment of complications. This may require rapid transfer of the patient to a tertiary centre to secure the aneurysm.
The number of clinical interventions is limited in SAH patients, but it has been shown that by treating patients in specialized centres that have a large volume of patients and multidisciplinary teams, their prognosis improves [161, 162]. “Large volume” is intended as at least 35 cases per year, with best benefits found in centres that treat more than 60 cases/year; in general, the higher the number of cases treated, the better the prognosis [163, 164].
Patients who survive the early hours are struck by three major complications: re-bleeding, delayed ischemia and hydrocephalus. In addition, there may be systemic complications that have a negative impact on prognosis.
1.2.10.1 Re-bleeding
Re-bleeding is a serious complication that significantly worsens the patient’s prognosis [165] and is probably related to the dissolution of the clot by natural fibrinolysis at the site of aneurysm rupture. The risk of re-bleeding occurs in 4–15 % of cases during the first post-bleed days and decreases gradually over the next 2 weeks [166, 167]. There are no more incidences of it once the aneurysm is secured [168].
Aneurysm Treatment
The most effective treatment to reduce the risk of re-bleeding is early exclusion of the aneurysm from the circulation causing the bleed. Treatment of the aneurysm can be either endovascular or surgical, by positioning a clip on its neck.
Endovascular treatment has the advantage of avoiding craniotomy and allows more rapid recovery after the procedure, but it needs angiographic follow-up because there is a risk of the aneurysm reopening due to coil compaction.
The choice of treatment is based on the location and morphology of the aneurysm neck. Middle cerebral aneurysms or aneurysms localized on tortuous vessels are treated surgically because of the difficulty in reaching them intravascularly, while endovascular treatment is easier with deep posterior circulation aneurysms. Patients with comorbidities are more often delivered endovascular treatment.
Endovascular Treatment of Aneurysms in Emergency
Endovascular treatment of aneurysms in emergency was introduced into clinical practice in the early 1990s and has gradually consolidated to become the treatment of choice in most cases. Some exceptions still remain.
Once satisfied that the bleeding episode was caused by the rupture of an aneurysm, and once the bleeding aneurysm has been identified by means of a CT angiography or an angiographic study, the usual approach is to intervene as soon as possible and usually in the first 24 h to prevent a further rupture, which is highly probable (about 30–40 % in the first weeks with peaks in the first few days).
Endovascular treatment is performed through catheterization of cerebral vessels, starting from the femoral artery (usually the right one for the logistics of the equipment). In extremely rare cases, access is achieved through direct puncture of the carotid artery, usually when femoral access fails.
The procedure is carried out by inserting coaxial catheters (one inside the other). A first tube, called the introducer (usually 2.5 mm calibre and 15 cm in length), is inserted into the femoral artery. The tube contains a guide catheter approximately 90 cm long and calibre 6 French (6 French = 2 mm), which is sent into the internal carotid or vertebral artery, up to a height corresponding more or less to the second-third cervical vertebra.
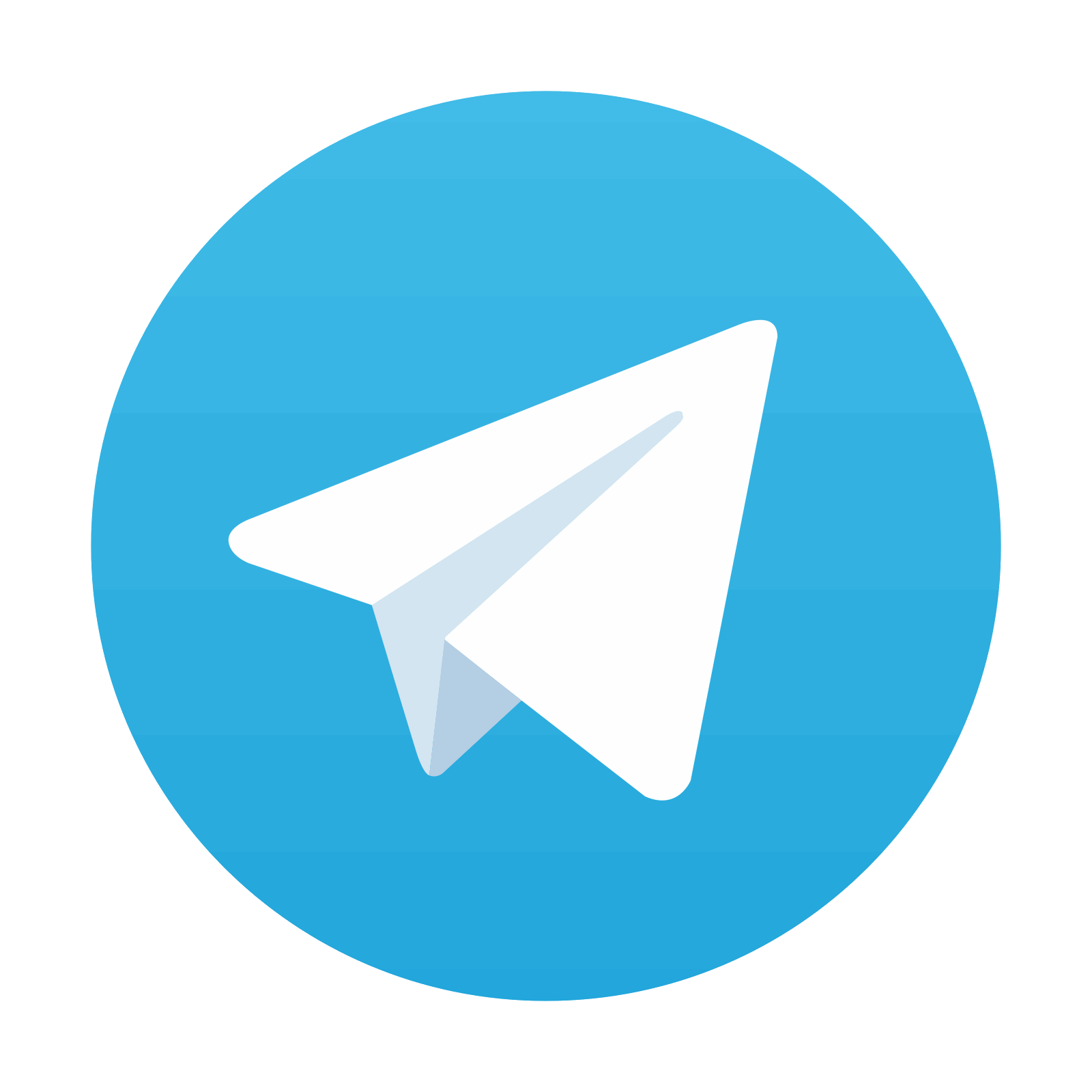
Stay updated, free articles. Join our Telegram channel

Full access? Get Clinical Tree
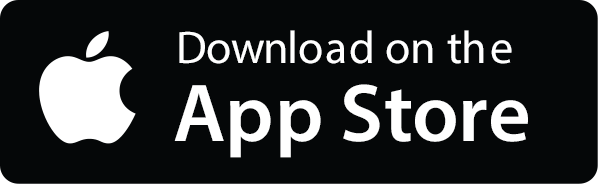
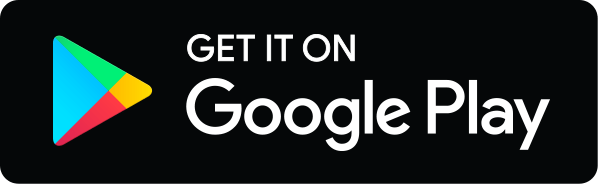
