Diffuse Gliomas
The WHO classification of brain tumors has undergone great change in the 2016 update to the 4th edition with regard to the diffuse, or infiltrating, gliomas (1). The unifying feature of the entities in this group is their pattern of infiltrative growth, which prevents them from being completely excised and contributes to their inexorably progressive nature. Now, in addition to the classic histomorphologic, phenotypic diagnoses along lines of cell type, there is a further refinement and subcategorization into molecular genetic categories, primarily based on the presence of isocitrate dehydrogenase (IDH) mutations and whether chromosome arms 1p and 19q are deleted, creating a “layered” or integrated genetic and morphologic diagnosis. This approach has improved the correlation between pathologic diagnosis and clinical outcome and has provided a more consistently objective set of variables by which these entities are defined. Furthermore, it has brought closer the once separate categories of diffuse astrocytoma and oligodendroglioma based on their shared genetic characteristics. At the same time, the approach has provided a means to reliably and reproducibly sort the once ambiguous and oftentimes problematic category of mixed oligoastrocytomas discretely into either oligodendrogliomas or astrocytomas, drawing a more sharp and unambiguous border between the two.
Ultimately, the most important large-scale categorization of infiltrating gliomas is whether or not they exhibit IDH1 or IDH2 mutations. Broadly speaking, two tumors with identical histologic findings will have significantly different outcomes and responses to treatment if one is IDH mutant and the other is IDH wild type. IDH-mutant infiltrating gliomas do so much better than their wild-type counterparts that IDH-mutant cases of the highest histologic grade often have a better clinical course than the lowest histologic grade of IDH-wild–type cases. Caveat lector: there are rare cases of low-grade diffuse gliomas in children that have their own unique set of genetic findings and are exceptions to this generalization.
If one then separates the infiltrating gliomas into IDH mutant and IDH wild type and then arranges them in order of 1p/19q status and histologic grade, the entities form a rough spectrum of clinical outcomes, with IDH-mutant, 1p/19q codeleted, and histologically low-grade tumors at one end with the best outcomes, and IDH-wild–type, 1p/19q intact, histologically high-grade tumors at the other (Table 3-1). That is the approach taken here in presenting the diffuse gliomas that previously occupied entirely separate
chapters. In some cases, there are histologic patterns that are associated with one IDH status, but not consistently. To avoid redundancy, those patterns are discussed once under the heading that more typically characterizes them.
chapters. In some cases, there are histologic patterns that are associated with one IDH status, but not consistently. To avoid redundancy, those patterns are discussed once under the heading that more typically characterizes them.
TABLE 3-1 Diffuse Gliomas as Categorized by WHO 2016 Update to 4th Ed. Classification of Tumours of the Central nervous System | |||||||||||||||||||||||
---|---|---|---|---|---|---|---|---|---|---|---|---|---|---|---|---|---|---|---|---|---|---|---|
|
IDH-Mutant Infiltrating Gliomas
Isocitrate Dehydrogenase
IDH is a metabolic enzyme associated with the Krebs or citric acid cycle that converts isocitrate to α-ketoglutarate by removing a carboxylic group. There are at least three isoforms in humans: IDH3 being more in the mitochondria and directly involved in the citric acid cycle, whereas IDH1 and IDH2 are cytoplasmic and not involved directly in the citric acid cycle (2).
In 2008, Parsons et al. published findings of DNA sequencing in glioblastomas, including a recurring point mutation, R132H, in the gene IDH1 that was associated with younger patient age and improved survival (3). That discovery led to finding IDH1 and IDH2 mutations in a large number of infiltrating gliomas, including most lower-grade lesions (4). Greater than 80% of IDH mutations are IDH1 R132H, the rest are other substitutions at codon 132 in IDH1 or are in codon 172 of IDH2. We now understand that IDH mutations are an early, inciting event in gliomagenesis and that those tumors constitute a genetically and clinically distinct group.
The mechanism by which IDH mutations lead to gliomas is through a toxic gain of function in which the mutant protein produces D-2-hydroxyglutarate, an “oncometabolite” that promotes proliferation and induces a globally hypermethylated genome (5,6) In the context of IDH mutation, additional upregulation of telomere lengthening allows the tumor cells to divide indefinitely. Oligodendrogliomas do that through activating mutations in the promoter for the gene TERT and astrocytomas through inactivation of ATRX, which is an inhibitor of telomere lengthening through the alternative pathway (7,8).
Oligodendroglioma, IDH Mutant, 1p19q Codeleted (WHO Grade II), Anaplastic Oligodendroglioma, IDH Mutant, and 1p19q Codeleted (WHO Grade III)
Oligodendrogliomas constituted approximately 8% of all gliomas in a recent large tumor registry with an annual incidence of about 0.5 per 100,000 person-years, and a 2:1 ratio of grade II to grade III tumors (9). The vast majority of oligodendrogliomas occur in adults during the fourth through sixth decades of life with median ages of around 41 years for grade II tumors and 49 years for grade III (9). As with infiltrating astrocytomas, males have a somewhat higher incidence by a ratio of about 3:2 (10,11). No familial tumor predispositions are specifically linked to oligodendrogliomas. Oligodendrogliomas are rare in children, and only show IDH mutations and 1p19q codeletions occasionally, and then mostly in older children and adolescents, supporting the idea that the vast majority of pediatric “oligodendrogliomas” are in fact a distinct tumor type (12).
![]() FIGURE 3-1 T2-weighted magnetic resonance imaging (MRI) showing a grade II oligodendroglioma, IDH mutant, 1p/19q codeleted, following the cortical contour, a feature commonly seen in such lesions. |
Nearly all oligodendrogliomas arise within the cerebral hemispheres, and most of the rare cases affecting the brainstem, cerebellum, and spinal cord are of unknown IDH status, therefore unconfirmed. Within the hemispheres, the mass typically infiltrates along the cortex, causing expansion and loss of the gray–white matter junction. This corticotropism may explain the high rate of seizures as presenting complaints in oligodendroglioma. Other nonspecific signs and symptoms of mass lesions, such as headache, nausea/vomiting, and focal neurologic deficits, are also common (13,14).
Radiology
Magnetic resonance imaging (MRI) shows a variably infiltrative intra-axial lesion in the cerebral hemispheres that often is centered in or follows the contours of the cortex. Grade II oligodendrogliomas are generally poorly circumscribed, homogeneously T2/FLAIR hyperintense and T1 hypointense, and without contrast enhancement, whereas grade III cases enhance and tend toward more circumscribed outlines (15). Calcifications are common and are more readily identified on CT imaging. The expanding cortical ribbon seen in MRI of oligodendrogliomas is similar to that of dysembryoplastic neuroepithelial tumor (DNT) (Figure 3-1), even sometimes showing nodularity and satellite lesions.
Treatment and Prognosis
According to CBTRUS registry data, the 5-year survival rate for patients with grade II oligodendrogliomas is about 80%, significantly higher than the 50% for grade II astrocytomas, although
these are not all genetically defined cases (9). Data from other series that include IDH status and codeletion also consistently show better survival for oligodendroglioma, albeit by varying margins (16,17). Anaplastic oligodendrogliomas, WHO grade III, have a 5-year survival rate of 55% to 60% (9). There is considerable individual variability in time to progression and overall survival. In the past, age was a powerful predictor of prognosis, with survival being inversely related to age at diagnosis. However, it’s unclear whether this difference remains after excluding genetically defined astrocytomas.
these are not all genetically defined cases (9). Data from other series that include IDH status and codeletion also consistently show better survival for oligodendroglioma, albeit by varying margins (16,17). Anaplastic oligodendrogliomas, WHO grade III, have a 5-year survival rate of 55% to 60% (9). There is considerable individual variability in time to progression and overall survival. In the past, age was a powerful predictor of prognosis, with survival being inversely related to age at diagnosis. However, it’s unclear whether this difference remains after excluding genetically defined astrocytomas.
Radiation and chemotherapy are effective against oligodendrogliomas, yet the effects are temporary, and even cases with a complete response tend to recur over time and progress to death from disease. Combination chemotherapy is a common and well-established initial treatment for oligodendrogliomas of either grade, consisting of procarbazine, lomustine (CCNU) and vincristine, known by abbreviation as “PCV,” although grade II lesions may not be treated at all until recurrence after resection. Temozolomide, the typical chemotherapeutic agent for glioblastomas, is frequently used as a second-line agent for recurrent cases, and is now increasingly being used as a first-line agent, particularly with anaplastic oligodendrogliomas (18). Radiotherapy is additionally used in many cases when there is subtotal resection, anaplasia, or recurrence (19). The exact role of radiation relative to chemotherapy remains undecided in oligodendrogliomas.
Histopathology
In their classical form, oligodendrogliomas are one of the iconic lesions of neuropathology, such that medical students with modest pathology instruction may recognize them; however, as a morphologic diagnosis, oligodendroglioma has shown great interobserver variability, especially with regard to mixed oligoastrocytic tumors (20). As a result of large collaborative studies of glioma genetics, it is now known that what was previously a “spectrum” diagnosis ranging from pure oligodendroglioma to pure astrocytoma, spanned by a poorly reproducible and ambiguous mixed category, is actually a sharply demarcated, binary distribution with regard to cell type. Oligodendrogliomas are now defined more by their genetic features, specifically IDH mutations and 1p/19q codeletion, than they are by histopathologic ones. Although the vast majority of oligodendrogliomas will have classical features, some will have histologic features that are more astrocytic. Regardless of how astrocytic the morphology appears, an infiltrating glioma with whole-arm 1p/19q codeletion and an IDH mutation is an oligodendroglioma. Below are the typical histologic features of oligodendrogliomas, none of which is present in all cases.
Nuclear features are consistent in oligodendrogliomas and are the most important histologic clue that an infiltrating glioma is oligodendroglial (14,21). Low-magnification views facilitate recognition of the uniformity of tumor nuclei, which is not usually seen in infiltrating astrocytomas. At higher magnification, the nuclei are round with regular contours and
robustly defined nuclear envelopes. Chromatin density is variable, but usually somewhat pale with a small, visible nucleolus (Figure 3-2). Although the nuclei in the preponderance of oligodendrogliomas exemplify this description, some grade III lesions can have marked atypia, especially following treatment (Figure 3-3).
robustly defined nuclear envelopes. Chromatin density is variable, but usually somewhat pale with a small, visible nucleolus (Figure 3-2). Although the nuclei in the preponderance of oligodendrogliomas exemplify this description, some grade III lesions can have marked atypia, especially following treatment (Figure 3-3).
![]() FIGURE 3-2 The typical histologic appearance of oligodendroglioma cells with uniform, round, regular nuclei containing pale chromatin and a single small nucleolus and surrounded by a small “halo.” |
![]() FIGURE 3-3 The uniformity of nuclear features of oligodendrogliomas deteriorates in some anaplastic oligodendrogliomas, but this finding is usually focal. |
![]() FIGURE 3-4 Cytoplasmic clearing results from delayed fixation and gives the impression of plant-like cell walls when prominent. |
The cytoplasm of oligodendroglial cells is classically clear, forming a halo and giving the widely known “fried egg” appearance (Figure 3-4). However, this effect is the result of an autolytic process that is halted when the tissue is either frozen or rapidly fixed, limiting its diagnostic utility. This clearing can also appear in other tumor types, including astrocytomas, ependymomas, neurocytomas, and DNT. Few processes, as the roots oligo and dendro suggest, extend from the cytoplasm, a trait best seen on crush preparations (Figure 3-5). In some cells, eccentric orbs of eosinophilic
cytoplasm create mini- or microgemistocytes that resemble gemistocytic astrocytes, except smaller with more monotonous and regular nuclei and scant cytoplasmic processes (Figures 3-6 and 3-7). Occasional cells may contain refractile, GFAP-positive, eosinophilic granules that should not be confused with the eosinophilic granular bodies (EGBs) of pilocytic astrocytoma and ganglioglioma (Figure 3-8) (22).
cytoplasm create mini- or microgemistocytes that resemble gemistocytic astrocytes, except smaller with more monotonous and regular nuclei and scant cytoplasmic processes (Figures 3-6 and 3-7). Occasional cells may contain refractile, GFAP-positive, eosinophilic granules that should not be confused with the eosinophilic granular bodies (EGBs) of pilocytic astrocytoma and ganglioglioma (Figure 3-8) (22).
![]() FIGURE 3-6 Minigemistocytes are characterized by process-poor smooth globose eosinophilic cytoplasm that indents the nucleus. |
![]() FIGURE 3-7 Some oligodendroglioma cells contain internally coarsely fibrillar cytoplasm but still extend few processes and are sometimes called “gliofibrillary oligodendrocytes.” |
![]() FIGURE 3-8 Scattered cells with eosinophilic granules appear in some anaplastic oligodendrogliomas and should not be confused with the eosinophilic granular bodies of lower-grade lesions. |
![]() FIGURE 3-9 Many oligodendrogliomas also have a rich capillary vasculature and scattered calcifications. |
Several other histologic findings are typical for oligodendrogliomas but are neither necessary nor sufficient for the diagnosis. Calcifications are common and can form among tumor cells (Figure 3-9) or precipitate within tumoral blood vessels. The vasculature comprises anastomosing delicate capillaries that resemble chicken wire or honeycomb (see Figure 3-9). Tumor cells collect along obstructions in their paths of invasion, making secondary structures,
seen as perivascular and subpial aggregates and perineuronal satellitosis (Figure 3-10). An abundant myxoid background is present in some oligodendrogliomas and may pool into microcystic spaces (Figure 3-11).
seen as perivascular and subpial aggregates and perineuronal satellitosis (Figure 3-10). An abundant myxoid background is present in some oligodendrogliomas and may pool into microcystic spaces (Figure 3-11).
![]() FIGURE 3-10 Perineuronal satellitosis is more characteristic of oligodendrogliomas, but it can also be seen in some infiltrating astrocytomas. |
Occasional oligodendrogliomas show morphologic evidence of neurocytic differentiation, including nucleus-free zones of neuropil and fibrillar rosettes (23).
![]() FIGURE 3-11 The mucoid ground substance produced by some oligodendrogliomas pools into microcystic spaces, resembling pilocytic astrocytoma and dysembryoplastic neuroepithelial tumor. |
Grade III anaplastic oligodendrogliomas have features similar to their grade II counterparts but have microvascular proliferation or endothelial hypertrophy, necrosis, and/or high mitotic rates. These features are discussed further in the section Grading.
Immunohistochemistry
The three most important immunostains for determining whether an infiltrating glioma is an oligodendroglioma are IDH1 R132H, ATRX, and p53. Greater than 90% of oligodendrogliomas will demonstrate cytoplasmic reactivity for the antibody to the mutant IDH1 protein, the remaining negative cases having alternate, nonimmunoreactive mutations of IDH1 or IDH2. Retained ATRX expression and a wild-type pattern of p53 staining further support the diagnosis of oligodendroglioma, although the latter can rarely be strong and diffuse (mutant pattern).
GFAP is positive in many oligodendrogliomas (∼70%) but often only in a subset of cells, including the mini/microgemistocytes and “gliofibrillary oligodendrocytes” which contain a narrow perinuclear rim of staining and scant processes (24,25). The tumor cells also show variable amounts of staining for synaptophysin, ranging from none to strong and diffuse, depending on the specific antibody used. This immunohistochemical evidence of neuronal differentiation in oligodendrogliomas is supported by frequent ultrastructural observation of neurosecretory granules and synapses (26). Other markers of more mature neuronal phenotype, neuronal nuclear antigen (NeuN) and phosphorylated neurofilament, are not seen, although a surprising number react with antibodies against nonphosphorylated neurofilament (27). Neurofilament staining is most useful to demonstrate invasion of native neurons by infiltrating tumor cells. MIB-1 indices are variable, commensurate with the grade of the individual tumor. Nuclear reactivity for p53, a surrogate for TP53 mutation, is uncommon in oligodendrogliomas and more typical of IDH-mutant astrocytomas (28).
Genetics
The diagnosis of oligodendroglioma in adults requires the presence of an IDH1 or IDH2 mutation, unless the IDH status is unable to be assessed (see oligodendroglioma, NOS). IDH-wild–type cases diagnosed as oligodendroglioma in pediatric patients are discussed under oligodendroglioma, NOS.
Originally an indicator of prognosis and response to therapy, codeletion of chromosome arms 1p and 19q is now definitional in combination with IDH mutations for the diagnosis of oligodendroglioma (29,30,31). The codeletion almost always results from a translocation of nearly the entire 1p and 19q arms, t(1;19)(q10;p10), leading to loss of the derivative chromosome, thus deletion of those arms (32). For many years, fluorescence in situ hybridization (FISH) directed at 1p36 and 19q13 was the standard method of identifying 1p/19q codeletion. However, this method also detects small deletions of those loci without loss of the remaining arm. Because of this, FISH detects “codeletion” in other gliomas, such as IDH-wild–type glioblastoma, in approximately 5% of cases. Therefore,
such false positives by FISH can result in a small number of IDH-mutant astrocytomas being misdiagnosed as oligodendrogliomas. Although FISH remains an acceptable method for detecting codeletion, other approaches that assess for whole-arm deletion may reduce the incidence of false positives due to focal deletions. Among these are loss of heterozygosity (LOH) by polymerase chain reaction (PCR), comparative genomic hybridization (CGH), or single nucleotide polymorphism (SNP) microarray.
such false positives by FISH can result in a small number of IDH-mutant astrocytomas being misdiagnosed as oligodendrogliomas. Although FISH remains an acceptable method for detecting codeletion, other approaches that assess for whole-arm deletion may reduce the incidence of false positives due to focal deletions. Among these are loss of heterozygosity (LOH) by polymerase chain reaction (PCR), comparative genomic hybridization (CGH), or single nucleotide polymorphism (SNP) microarray.
Another genetic feature that is present in almost all cases of oligodendroglioma is activating mutations of the TERT promoter, although this finding is not specific (33). TERT is the gene for telomere reverse transcriptase, which is a component of the telomerase complex that functions to lengthen the repeat sequences at the ends of chromosomes; the telomeres, thereby increasing the number of times cells can divide. TERT promoter mutations in oligodendrogliomas result in higher expression of TERT. In the setting of an IDH-mutant infiltrating glioma, the presence of a TERT promoter mutation is strongly suggestive of a 1p/19q codeletion. However, TERT promoter mutations are also common in IDH-wild–type infiltrating astrocytomas, so diagnostic conclusions based on TERT promoter status should only follow thorough assessment of IDH1 and IDH2 mutation status. Even then, TERT mutations are also occasionally present in IDH-mutant astrocytomas (16,34).
Other common genetic findings in oligodendrogliomas include inactivating mutations of CIC, FUBP1, and NOTCH1, which may be present singly or in combination, and at least one of which is present in most cases of genetically defined oligodendroglioma. FUBP1 and CIC are within deleted segments of 1p and 19q, respectively, and mutations in them are extremely rare in other gliomas. NOTCH1 mutations may be seen in other infiltrating gliomas and are associated with progression in oligodendrogliomas.
Grading
Because IDH status was not available for incorporation into older series, looking at histologic grading of oligodendrogliomas, it’s unclear to what degree any potential contamination with IDH-wild–type gliomas may have contributed to the conclusions of those studies. Nevertheless, there does appear to be a difference in survival among oligodendroglioma patients based on histologic grade (16,37).
The specific criteria for what constitutes anaplasia in oligodendrogliomas are not rigidly defined in the 2016 WHO update (1). Grade II oligodendrogliomas can show occasional mitotic figures, cytologic atypia, and dense hypercellularity. The minimum 2016 WHO criteria for grade III anaplastic oligodendroglioma are “brisk mitotic activity” and “conspicuous
microvascular proliferation,” without further definition. Mentioned, but not recommended, is the definition of brisk mitotic activity from a large series supporting the importance of that feature: 6 or more mitotic figures in 10 high-magnification (400×) fields (37). Necrosis, palisading or not, is another criterion for anaplasia that is widely accepted (Figure 3-12). As a soft sign, high-grade examples also commonly develop cytologic features that are more epithelioid, with increased cytoplasm, crisp cytoplasmic borders, and prominent nucleoli. A small number of grade III cases, especially those following treatment, also exhibit marked nuclear pleomorphism and/or coarsely granular tumor cells that resemble EGBs (Figure 3-13). Such nuclear pleomorphism may raise concern for glioblastoma, but oligodendroglial lesions will retain their paucity of fibrillar processes. Palisading of tumor cells around necrosis is uncommon but may occasionally be seen and should not be misconstrued as evidence of glioblastoma in an IDH-mutant, 1p/19q-codeleted tumor.
microvascular proliferation,” without further definition. Mentioned, but not recommended, is the definition of brisk mitotic activity from a large series supporting the importance of that feature: 6 or more mitotic figures in 10 high-magnification (400×) fields (37). Necrosis, palisading or not, is another criterion for anaplasia that is widely accepted (Figure 3-12). As a soft sign, high-grade examples also commonly develop cytologic features that are more epithelioid, with increased cytoplasm, crisp cytoplasmic borders, and prominent nucleoli. A small number of grade III cases, especially those following treatment, also exhibit marked nuclear pleomorphism and/or coarsely granular tumor cells that resemble EGBs (Figure 3-13). Such nuclear pleomorphism may raise concern for glioblastoma, but oligodendroglial lesions will retain their paucity of fibrillar processes. Palisading of tumor cells around necrosis is uncommon but may occasionally be seen and should not be misconstrued as evidence of glioblastoma in an IDH-mutant, 1p/19q-codeleted tumor.
Differential Diagnosis
Although rounded nuclei with haloes are typical for oligodendrogliomas, they appear in myriad other CNS neoplasms; therefore, they are by no means specific. The adoption of IDH mutations and 1p/19q codeletion as definitional features in adult oligodendrogliomas has, however, made that diagnosis more objective and simplified the exclusion of morphologic mimics. After one has ruled out IDH mutations and 1p/19q codeletion in an oligodendroglial-appearing tumor, these other entities should be considered.
In children, pilocytic astrocytoma, DNT, and infiltrating astrocytoma are usually in the differential diagnosis with oligodendroglioma; however,
the first two are much more likely in pediatric patients. The haloed round nuclei of DNT can intermingle in an infiltrative pattern with native brain, creating an appearance identical to oligodendroglioma, especially in small samples where more typical areas of DNT are lacking. Even when typical areas are present, myxoid and microcystic backgrounds may be seen in both and can significantly overlap. Nevertheless, several morphologic features can usually distinguish the two: compared with DNT, oligodendrogliomas have more open chromatin and prominent nucleoli, higher proliferation indices, and lack “floating neurons.” Evidence of IDH mutation or whole-arm 1p/19q codeletion effectively rules out a diagnosis of DNT, although sample size and cellularity can occasionally make such testing difficult. Mutations or other alterations of FGFR1 and, to a lesser degree BRAF, have been described in DNTs and can support that diagnosis (38,39).
the first two are much more likely in pediatric patients. The haloed round nuclei of DNT can intermingle in an infiltrative pattern with native brain, creating an appearance identical to oligodendroglioma, especially in small samples where more typical areas of DNT are lacking. Even when typical areas are present, myxoid and microcystic backgrounds may be seen in both and can significantly overlap. Nevertheless, several morphologic features can usually distinguish the two: compared with DNT, oligodendrogliomas have more open chromatin and prominent nucleoli, higher proliferation indices, and lack “floating neurons.” Evidence of IDH mutation or whole-arm 1p/19q codeletion effectively rules out a diagnosis of DNT, although sample size and cellularity can occasionally make such testing difficult. Mutations or other alterations of FGFR1 and, to a lesser degree BRAF, have been described in DNTs and can support that diagnosis (38,39).
Typical cases with biphasic growth, EGBs, and Rosenthal fibers aside, pilocytic astrocytoma can appear almost identical to oligodendroglioma. These oligo-like cases are more common in the posterior fossa/cerebellum and are usually poorly infiltrative, containing few native axons. Any diagnosis of oligodendroglioma in the posterior fossa of a child that is both low grade and contrast enhancing is dubious, even with a diffuse growth pattern. Identification of a KIAA1549-BRAF gene fusion, which is present in most posterior fossa pilocytic astrocytomas, may be helpful in confirming that diagnosis and ruling out oligodendroglioma (40). In both pilocytic astrocytoma and DNT, curable grade I lesions, the distinction from oligodendroglioma is critical.
The distinction of oligodendroglioma from diffuse astrocytoma is now based on genetic characteristics. All infiltrating gliomas, regardless of morphology, should be tested for IDH mutations and 1p/19q codeletion. Morphologically, oligodendrogliomas have monomorphic, round, regular nuclei with open, fine chromatin, and small nucleoli. The nuclei of astrocytomas are more pleomorphic and elongate with irregular contours and denser chromatin. By analogy, the nuclei of oligodendrogliomas are like Florida oranges, and those of diffuse astrocytomas are like Idaho potatoes (Figure 3-14). Small-cell astrocytomas/glioblastomas are an exception and share many histologic features with oligodendroglioma, including monomorphic nuclei, haloes, capillary-rich vasculature, and microcalcifications. Immunostaining can usually strongly suggest oligodendroglioma in the context of the appropriate morphology. IDH1 R132H reactivity with retention of ATRX nuclear staining and lack of strong p53 expression make IDH-mutant astrocytoma highly unlikely.
Patients with long-standing epilepsy may accumulate an excess of nonneoplastic oligodendrocytes, resulting in hypercellular white matter with prominent perivascular accumulations that resemble secondary structures in oligodendroglioma (Figure 3-15). These hyperplastic cells are
indistinguishable from other native oligodendroglia, unlike neoplastic oligodendroglia that usually have slightly larger nuclei. Mitotic figures may even be encountered rarely. MRI showing T2-FLAIR hyperintensity favors a neoplastic lesion. Demonstration of 1p or 19q deletion or mutant IDH1 essentially rules out a reactive process.
indistinguishable from other native oligodendroglia, unlike neoplastic oligodendroglia that usually have slightly larger nuclei. Mitotic figures may even be encountered rarely. MRI showing T2-FLAIR hyperintensity favors a neoplastic lesion. Demonstration of 1p or 19q deletion or mutant IDH1 essentially rules out a reactive process.
![]() FIGURE 3-15 Nonneoplastic oligodendroglia accumulate in the white matter of patients with long-standing epilepsy, even forming false secondary structures around blood vessels. |
Central and extraventricular neurocytomas are similar to oligodendrogliomas in monomorphism and capillary vasculature and are subject to the same perinuclear haloes with delayed fixation. Oligodendrogliomas can occasionally show significant immunoreactivity for synaptophysin, but generally lack anucleate zones of neuropil and fibrillar rosettes, with rare exceptions. Widespread immunoreactivity for NeuN is present in most neurocytomas and only rarely in oligodendrogliomas (41). Neurocytomas with 1p/19q codeletion have been reported, although their IDH status was unknown, so it is unclear whether they could have been oligodendrogliomas with neurocytic differentiation (42).
Diffuse leptomeningeal glioneuronal tumor (DLGT), a rare tumor that is usually low grade and occurs in children, has an oligodendroglial histology, similar immunohistochemical profile, and frequent deletions of 1p and/or 19q by FISH. However, there are no reported cases of this entity with IDH1 mutations and only a minority is 1p/19q codeleted (43,44). Furthermore, most DLGTs show KIAA1549-BRAF fusions similar to pilocytic astrocytomas (45).
Oligodendroglioma, NOS (WHO Grade II) and Anaplastic Oligodendroglioma, NOS (WHO Grade III)
In some cases with classic oligodendroglioma histology, assessment of IDH and/or 1p/19q status may not be feasible for whatever reason. In such cases, a diagnosis of “oligodendroglioma, NOS” may be issued with the understanding that it is “provisional.” Even when immunohistochemistry strongly supports a diagnosis of oligodendroglioma (positive IDH1 R132H and ATRX and wild-type pattern p53), it is not a substitute for 1p/19q status, and the modifier NOS applies (1).
In most pediatric cases diagnosed as oligodendroglioma (WHO grade II), there is neither an IDH mutation nor whole arm 1p/19q codeletion. Although the 2016 WHO tacitly recognizes IDH-wild–type oligodendrogliomas, it is, in my opinion, a diagnosis to be strenuously avoided in children. Available data from sequencing arrays show that IDH-wild–type, grade II oligodendrogliomas in children have a similar set of genetic abnormalities as grade I tumors such as DNT, angiocentric glioma, and rosette-forming glioneuronal tumor (38,46,47). Such grade I lesions may recur over time but are indolent, rarely progress to malignancy and are potentially curable by surgery. A diagnosis of grade II oligodendroglioma, NOS may encourage less aggressive surgery, because such tumors are typically not resectable, increasing the likelihood of eventual recurrence and persistence of seizures, if present. That diagnosis may also result in unnecessary chemotherapy, or even radiation in some cases, both of which carry risk of harm to the patient. Anaplastic IDH-wild–type cases are rare and less controversial as regards treatment, yet there is little evidence beyond subjective morphologic impression that such cases are indeed oligodendrogliomas.
Oligoastrocytoma, NOS (WHO Grade II) and Anaplastic Oligoastrocytoma, NOS (WHO Grade III)
The 2016 WHO discourages use of the term oligoastrocytoma except in rare cases that are morphologically ambiguous and cannot be adequately tested for molecular markers of oligodendroglioma or astrocytoma, such as 1p/19q codeletion, TERT mutation, IDH mutation, ATRX loss, or p53 mutation. As with oligodendroglioma, NOS, it should be understood to be a provisional diagnosis. There are exceptionally rare “dual-genotype” cases in which there are two separate populations that show the genetic findings of oligodendroglioma and astrocytoma (48,49). Such cases could reasonably be called oligoastrocytomas after thorough workup, even though the “dual-genotype” cases explicitly do not constitute an official category in the WHO 2016 update (1).
Diffuse Astrocytoma, IDH Mutant (WHO Grade II) and Anaplastic Astrocytoma, IDH Mutant (WHO Grade III)
Clinical Context
Grade II and III astrocytomas are less common than glioblastomas, making up only about 8% of adult neuroepithelial tumors. Of those, around three-quarters contain a point mutation in the IDH1 or, less commonly, IDH2 gene (50). The incidence of IDH-mutant astrocytomas peaks in the third and fourth decades of life, with no significant differences between grades II and III (51). IDH mutations are rare in the pediatric population and primarily occur in adolescents. There is a modest male predominance for both grades (10,16). The signs and symptoms at presentation vary widely, ranging from acute onset of seizures to subtle and slowly progressive deficits in speech, mentation, movement, etc., sometimes over the course of months. Seizures are the most common presenting sign. IDH-mutant astrocytomas overwhelmingly occur in the cerebral hemispheres, showing a mild predilection for the frontal and temporal lobes, and are rare in the cerebellum, brain stem, and spinal cord. A larger proportion of grade II astrocytomas have IDH mutations than grade III, the latter having a higher frequency of IDH-wild–type cases.
Neuroimaging by magnetic resonance (MR) shows a poorly defined lesion with mild T1 hypointensity and T2 hyperintensity that often advances along white matter tracts (Figure 3-16). Increased T2 signal is thought to mark the presence of edema in areas infiltrated by tumor.
Gadolinium contrast material has no effect in grade II astrocytomas, whereas it causes enhancement in a subset of grade III cases, providing the radiologist a clue to higher grade (52). A lack of contrast enhancement, however, does not rule out the possibility of an anaplastic lesion. The tumor’s epicenter usually lies within the white matter. Areas of brain permeated by neoplastic cells are often expanded, causing subtle mass effects on surrounding structures. Spread from one hemisphere to the other along the corpus callosum or a commissure is typical for infiltrating astrocytomas. Circumscription, cyst formation with or without a mural nodule, and contrast enhancement each argue strongly against a grade II astrocytoma. The differential diagnosis on imaging broadens for grade III lesions, but these cases typically present less of a challenge histologically because of their higher cellularity and nuclear pleomorphism.
Gadolinium contrast material has no effect in grade II astrocytomas, whereas it causes enhancement in a subset of grade III cases, providing the radiologist a clue to higher grade (52). A lack of contrast enhancement, however, does not rule out the possibility of an anaplastic lesion. The tumor’s epicenter usually lies within the white matter. Areas of brain permeated by neoplastic cells are often expanded, causing subtle mass effects on surrounding structures. Spread from one hemisphere to the other along the corpus callosum or a commissure is typical for infiltrating astrocytomas. Circumscription, cyst formation with or without a mural nodule, and contrast enhancement each argue strongly against a grade II astrocytoma. The differential diagnosis on imaging broadens for grade III lesions, but these cases typically present less of a challenge histologically because of their higher cellularity and nuclear pleomorphism.
Therapy protocols for grade II astrocytomas generally include radiation only when symptomatic or when surveillance imaging shows progression. Grade III astrocytomas receive radiotherapy upon diagnosis, sometimes accompanied by temozolomide chemotherapy. The behavior of diffuse astrocytomas is variable, yet virtually all progress in extent and grade over time and are resistant to therapy. Although many patients receive a gross total resection, due to the infiltrative nature of the tumor cells, microscopic residual disease is essentially always present.
In the past, there was a significant difference in survival between grade II and grade III diffuse astrocytomas (28,53,54,55). However, when segregated by IDH mutation status, the difference in survival among IDH-mutant cases based on WHO histologic grade decreases, around 11 years median overall survival for grade II and 9 years for grade III (51,56). Older series had also indicated a survival advantage in grade II and III astrocytomas for younger patients, although this was likely also due to greater numbers of IDH-wild–type tumors with shorter survival and higher age at diagnosis. There is little correlation with age and survival among IDH-mutant astrocytomas, grades II and III (51).
Histopathology
The separate sections below are intended only to represent histologic patterns that may be seen in IDH-mutant astrocytomas, in order to facilitate their recognition. The following morphologic patterns are not suggestions for diagnostic terminology and should be taken to represent the variety of histologic appearances among grades II and III IDH-mutant astrocytomas. Diagnoses should be limited to the either diffuse astrocytoma, IDH mutant (WHO grade II), or anaplastic astrocytoma, IDH mutant (WHO grade III).
Fibrillary
The most common histologic pattern of both IDH-mutant and IDH-wild–type astrocytoma is the “fibrillary,” which is composed of tumor cell nuclei distributed singly throughout brain parenchyma, imparting varying degrees of hypercellularity. The neoplastic cells most often appear as “naked nuclei” that have little or no discernible fibrillar cytoplasm on routine sections and are generally larger and have more folds and irregular
contours of the nuclear membrane than their nonneoplastic counterparts (Figure 3-17). The chromatin pattern ranges from the more common hyperchromatic and coarse to the occasional open and smooth. Nucleoli are usually subtle in infiltrating astrocytomas. Sometimes the nuclei lack hyperchromasia, enlargement and irregularity, making their morphologic recognition difficult without complementary methods. Perinuclear clearing, or “haloes,” is a typical feature of oligodendrogliomas that can also appear in the cells of astrocytomas. In such cases, nuclear pleomorphism and irregularity favor astrocytic differentiation. By definition, necrosis and endothelial proliferation are absent. The WHO discourages diagnostic use of the term “fibrillary,” and it is presented here more for historical context.
contours of the nuclear membrane than their nonneoplastic counterparts (Figure 3-17). The chromatin pattern ranges from the more common hyperchromatic and coarse to the occasional open and smooth. Nucleoli are usually subtle in infiltrating astrocytomas. Sometimes the nuclei lack hyperchromasia, enlargement and irregularity, making their morphologic recognition difficult without complementary methods. Perinuclear clearing, or “haloes,” is a typical feature of oligodendrogliomas that can also appear in the cells of astrocytomas. In such cases, nuclear pleomorphism and irregularity favor astrocytic differentiation. By definition, necrosis and endothelial proliferation are absent. The WHO discourages diagnostic use of the term “fibrillary,” and it is presented here more for historical context.
![]() FIGURE 3-17 Grade II infiltrating astrocytomas are usually modestly cellular and contain large and hyperchromatic nuclei with irregular nuclear contours. |
Although histologic grading appears not to have as much prognostic value in IDH-mutant astrocytomas, current WHO grading guidelines for them remain in effect (31). According to those guidelines, a single mitotic figure is not sufficient to raise the grade of an infiltrating astrocytoma to grade III. A single instance of mitosis must be considered in the context of the total area of the tissue section; a small needle or endoscopic biopsy may only need one to achieve grade III status, whereas one matters less in a large open biopsy. The rate of Ki-67/MIB1 staining is a useful adjunct to mitotic count in these situations. Correlation with radiology and clinical history may also be helpful. Other “soft” features that indicate grade III astrocytoma include greater degrees of hypercellularity and nuclear pleomorphism than grade II lesions (Figure 3-18).
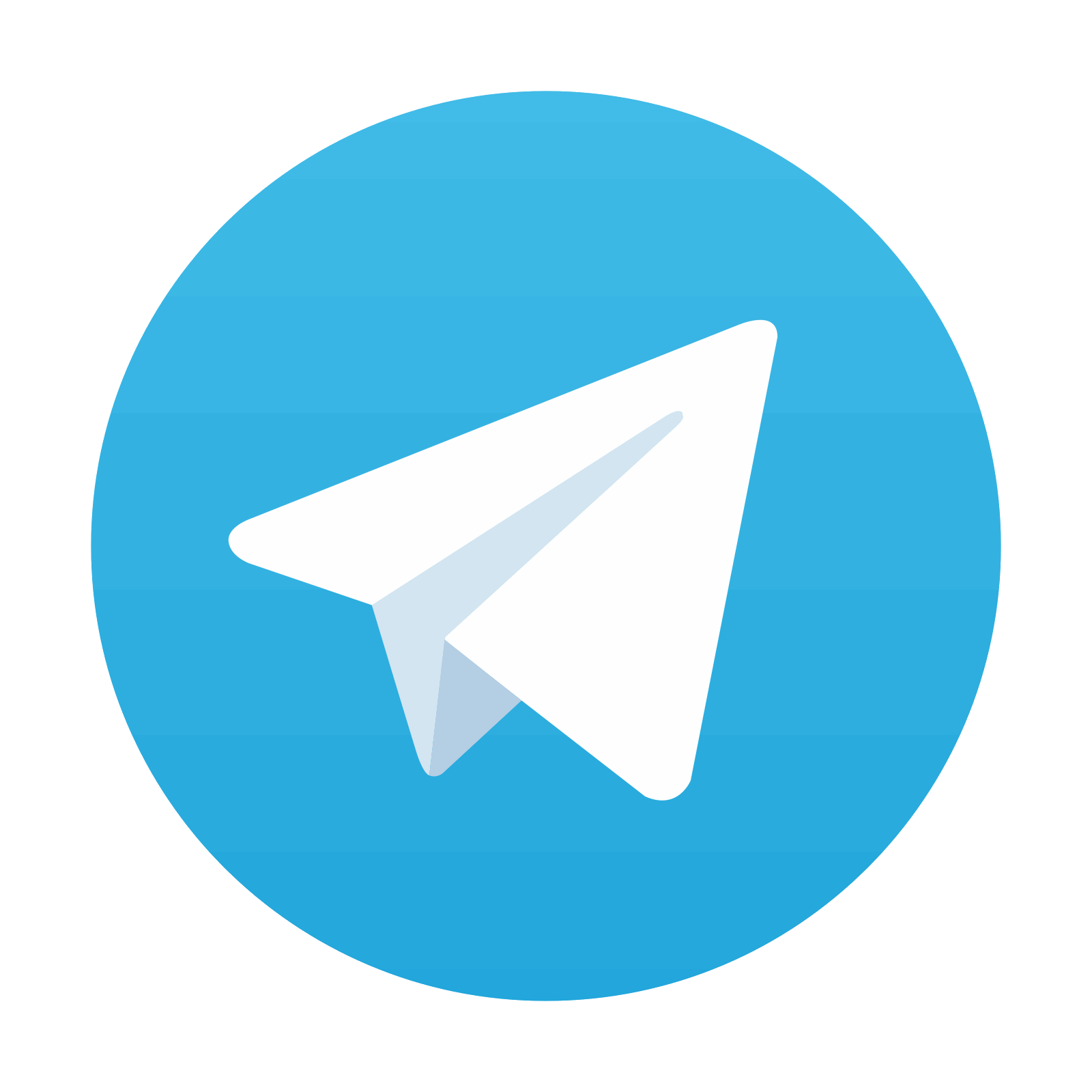
Stay updated, free articles. Join our Telegram channel

Full access? Get Clinical Tree
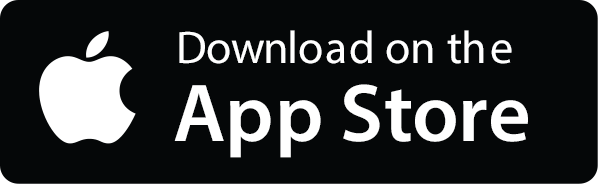
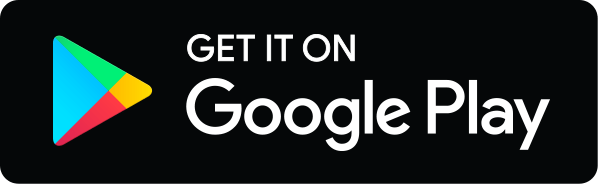
