All environmental chemicals necessary for life enter the body by the nose and mouth. The senses of smell (olfaction) and taste (gustation) monitor such chemicals, determine the flavor and palatability of foods and beverages, and warn of dangerous environmental conditions, including fire, air pollution, leaking natural gas, and bacteria-laden foodstuffs. These senses contribute significantly to quality of life and, when dysfunctional, can have untoward physical and psychological consequences. A basic understanding of these senses in health and disease is critical for the physician, because thousands of patients present to doctors’ offices each year with complaints of chemosensory dysfunction. Among the more important recent developments in neurology is the discovery that decreased smell function is among the first signs, if not the first sign, of such neurodegenerative diseases as Parkinson’s disease (PD) and Alzheimer’s disease (AD), signifying their “presymptomatic” phase.
Odorous chemicals enter the front of nose during inhalation and active sniffing, as well as the back of the nose (nasopharynx) during deglutition. After reaching the highest recesses of the nasal cavity, they dissolve in the olfactory mucus and diffuse or are actively transported by specialized proteins to receptors located on the cilia of olfactory receptor cells. The cilia, dendrites, cell bodies, and proximal axonal segments of these bipolar cells are located within a unique neuroepithelium covering the cribriform plate, the superior nasal septum, superior turbinate, and sectors of the middle turbinate (Fig. 28-1). Each of the ~6 million bipolar receptor cells expresses only one of ~450 receptor protein types, most of which respond to more than a single chemical. When damaged, the receptor cells can be replaced by stem cells near the basement membrane. Unfortunately, such replacement is often incomplete.
After coalescing into bundles surrounded by glia-like ensheathing cells (termed fila), the receptor cell axons pass through the cribriform plate to the olfactory bulbs, where they synapse with dendrites of other cell types within the glomeruli (Fig. 28-2). These spherical structures, which make up a distinct layer of the olfactory bulb, are a site of convergence of information, because many more fibers enter than leave them. Receptor cells that express the same type of receptor project to the same glomeruli, effectively making each glomerulus a functional unit. The major projection neurons of the olfactory system—the mitral and tufted cells—send primary dendrites into the glomeruli, connecting not only with the incoming receptor cell axons, but with dendrites of periglomerular cells. The activity of the mitral/tufted cells is modulated by the periglomerular cells, secondary dendrites from other mitral/tufted cells, and granule cells, the most numerous cells of the bulb. The latter cells, which are largely GABAergic, receive inputs from central brain structures and modulate the output of the mitral/tufted cells. Interestingly, like the olfactory receptor cells, some cells within the bulb undergo replacement. Thus, neuroblasts formed within the anterior subventricular zone of the brain migrate along the rostral migratory stream, ultimately becoming granule and periglomerular cells.
FIGURE 28-2
Schematic of the layers and wiring of the olfactory bulb. Each receptor type (red, green, blue) projects to a common glomerulus. The neural activity within each glomerulus is modulated by periglomerular cells. The activity of the primary projection cells, the mitral and tufted cells, is modulated by granule cells, periglomerular cells, and secondary dendrites from adjacent mitral and tufted cells. (From www.medicine.yale.edu/neurosurgery.)

The axons of the mitral and tufted cells synapse within the primary olfactory cortex (POC) (Fig. 28-3). The POC is defined as those cortical structures that receive direct projections from the olfactory bulb, most notably the piriform and entorhinal cortices. Although olfaction is unique in that its initial afferent projections bypass the thalamus, persons with damage to the thalamus can exhibit olfactory deficits, particularly ones of odor identification. Such deficits likely reflect the involvement of thalamic connections between the primary olfactory cortex and the orbitofrontal cortex (OFC), where odor identification occurs. The close anatomic ties between the olfactory system and the amygdala, hippocampus, and hypothalamus help to explain the intimate associations between odor perception and cognitive functions such as memory, motivation, arousal, autonomic activity, digestion, and sex.
Tastants are sensed by specialized receptor cells present within taste buds—small grapefruit-like segmented structures located on the lateral margins and dorsum of the tongue, roof of the mouth, pharynx, larynx, and superior esophagus (Fig. 28-4). Lingual taste buds are imbedded in well-defined protuberances, termed fungiform, foliate, and circumvallate papillae. After dissolving in a liquid, tastants enter the opening of the taste bud—the taste pore—and bind to receptors on microvilli, small extensions of receptor cells within each taste bud. Such binding changes the electrical potential across the taste cell, resulting in neurotransmitter release onto the first-order taste neurons. Although humans have ~7500 taste buds, not all harbor taste-sensitive cells; some contain only one class of receptor (e.g., cells responsive only to sugars), whereas others contain cells sensitive to more than one class. The number of taste receptor cells per taste bud ranges from zero to well over 100. A small family of three G-protein-coupled receptors (GPCRs), namely T1R1, T1R2, and T1R3, mediate sweet and umami taste sensations. Bitter sensations, on the other hand, depend on T2R receptors, a family of ~30 GPCRs expressed on cells different from those that express the sweet and umami receptors. T2Rs sense a wide range of bitter substances but do not distinguish among them. Sour tastants are sensed by the PKD2L1 receptor, a member of the transient receptor potential protein (TRP) family. Perception of salty sensations, such as induced by sodium chloride, arises from the entry of Na+ ions into the cells via specialized membrane channels, such as the amiloride-sensitive Na+ channel.
Recent studies have found that both bitter and sweet taste-related receptors are also present elsewhere in the body, most notably in the alimentary and respiratory tracts. This important discovery generalizes the concept of taste-related chemoreception to areas of the body beyond the mouth and throat, with α-gustducin, the taste-specific G-protein α-subunit, expressed in so-called brush cells found specifically within the human trachea, lung, pancreas, and gallbladder. These brush cells are rich in nitric oxide (NO) synthase, known to defend against xenobiotic organisms, protect the mucosa from acid-induced lesions, and, in the case of the gastrointestinal tract, stimulate vagal and splanchnic afferent neurons. NO further acts on nearby cells, including enteroendocrine cells, absorptive or secretory epithelial cells, mucosal blood vessels, and cells of the immune system. Members of the T2R family of bitter receptors and the sweet receptors of the T1R family have been identified within the gastrointestinal tract and in enteroendocrine cell lines. In some cases, these receptors are important for metabolism, with the T1R3 receptors and gustducin playing decisive roles in the sensing and transport of dietary sugars from the intestinal lumen into absorptive enterocytes via a sodium-dependent glucose transporter and in regulation of hormone release from gut enteroendocrine cells. In other cases, these receptors may be important for airway protection, with a number of T2R bitter receptors in the motile cilia of the human airway that responded to bitter compounds by increasing their beat frequency. One specific T2R38 taste receptor is expressed in human upper respiratory epithelia and responds to acyl-monoserine lactone quorum-sensing molecules secreted by Pseudomonas aeruginosa and other gram-negative bacteria. Differences in T2R38 functionality, as related to TAS2R38 genotype, correlate with susceptibility to upper respiratory infections in humans.
Taste information is sent to the brain via three cranial nerves (CNs): CN VII (the facial nerve, which involves the intermediate nerve with its branches, the greater petrosal and chorda tympani nerves), CN IX (the glossopharyngeal nerve), and CN X (the vagus nerve) (Fig. 28-5). CN VII innervates the anterior tongue and all of the soft palate, CN IX innervates the posterior tongue, and CN X innervates the laryngeal surface of the epiglottis, larynx, and proximal portion of the esophagus. The mandibular branch of CN V (V3) conveys somatosensory information (e.g., touch, burning, cooling, irritation) to the brain. Although not technically a gustatory nerve, CN V shares primary nerve routes with many of the gustatory nerve fibers and adds temperature, texture, pungency, and spiciness to the taste experience. The chorda tympani nerve is famous for taking a recurrent course through the facial canal in the petrosal portion of the temporal bone, passing through the middle ear, and then exiting the skull via the petrotympanic fissure, where it joins the lingual nerve (a division of CN V) near the tongue. This nerve also carries parasympathetic fibers to the submandibular and sublingual glands, whereas the greater petrosal nerve supplies the palatine glands, thereby influencing saliva production.
FIGURE 28-5
Schematic of the cranial nerves (CNs) that mediate taste function, including the chorda tympani nerve (CN VII), the glossopharyngeal nerve (CN IX), and the vagus nerve (CN X). (Copyright David Klemm, Faculty and Curriculum Support [FACS], Georgetown University Medical Center; used with permission.)

The axons of the projection cells, which synapse with taste buds, enter the rostral portion of the nucleus of the solitary tract (NTS) within the medulla of the brainstem (Fig. 28-5). From the NTS, neurons then project to a division of the ventroposteromedial thalamic nucleus (VPM) via the medial lemniscus. From here, projections are made to the rostral part of the frontal operculum and adjoining insula, a brain region considered the primary taste cortex (PTC). Projections from the PTC then go to the secondary taste cortex, namely the caudolateral OFC. This brain region is involved in the conscious recognition of taste qualities. Moreover, because it contains cells that are activated by several sensory modalities, it is likely a center for establishing “flavor.”
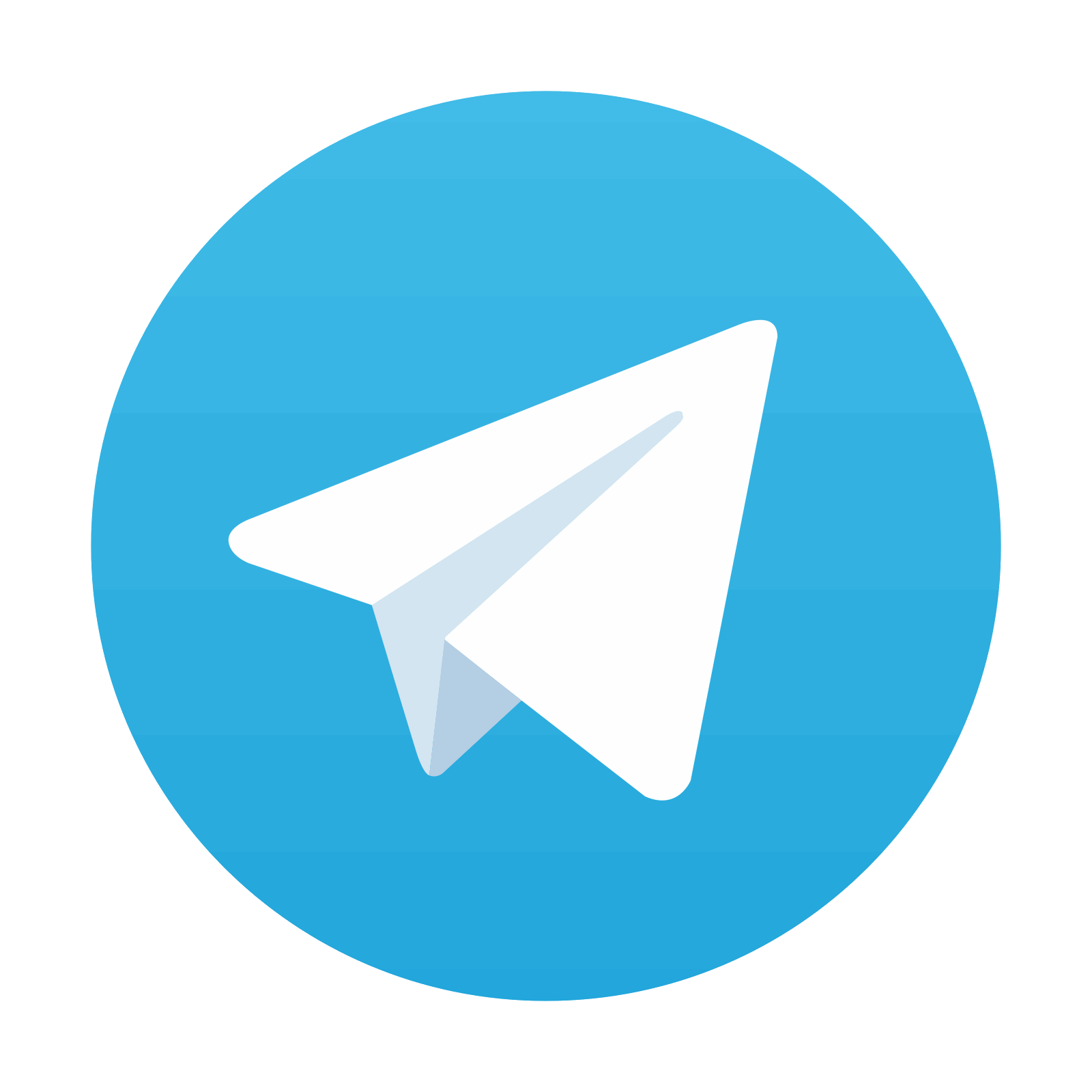
Stay updated, free articles. Join our Telegram channel

Full access? Get Clinical Tree
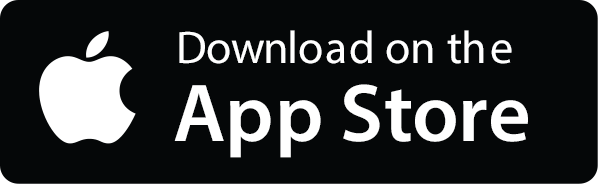
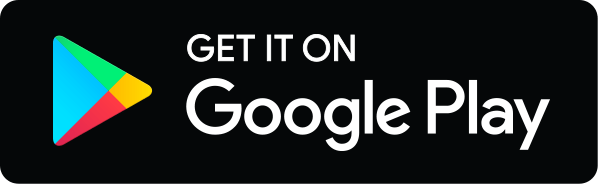