The visual system provides a supremely efficient means for the rapid assimilation of information from the environment to aid in the guidance of behavior. The act of seeing begins with the capture of images focused by the cornea and lens on a light-sensitive membrane in the back of the eye called the retina. The retina is actually part of the brain, banished to the periphery to serve as a transducer for the conversion of patterns of light energy into neuronal signals. Light is absorbed by pigment in two types of photoreceptors: rods and cones. In the human retina there are 100 million rods and 5 million cones. The rods operate in dim (scotopic) illumination. The cones function under daylight (photopic) conditions. The cone system is specialized for color perception and high spatial resolution. The majority of cones are within the macula, the portion of the retina that serves the central 10° of vision. In the middle of the macula a small pit termed the fovea, packed exclusively with cones, provides the best visual acuity.
Photoreceptors hyperpolarize in response to light, activating bipolar, amacrine, and horizontal cells in the inner nuclear layer. After processing of photoreceptor responses by this complex retinal circuit, the flow of sensory information ultimately converges on a final common pathway: the ganglion cells. These cells translate the visual image impinging on the retina into a continuously varying barrage of action potentials that propagates along the primary optic pathway to visual centers within the brain. There are a million ganglion cells in each retina and hence a million fibers in each optic nerve.
Ganglion cell axons sweep along the inner surface of the retina in the nerve fiber layer, exit the eye at the optic disc, and travel through the optic nerve, optic chiasm, and optic tract to reach targets in the brain. The majority of fibers synapse on cells in the lateral geniculate body, a thalamic relay station. Cells in the lateral geniculate body project in turn to the primary visual cortex. This afferent retinogeniculocortical sensory pathway provides the neural substrate for visual perception. Although the lateral geniculate body is the main target of the retina, separate classes of ganglion cells project to other subcortical visual nuclei involved in different functions. Ganglion cells that mediate pupillary constriction and circadian rhythms are light sensitive owing to a novel visual pigment, melanopsin. Pupil responses are mediated by input to the pretectal olivary nuclei in the midbrain. The pretectal nuclei send their output to the Edinger-Westphal nuclei, which in turn provide parasympathetic innervation to the iris sphincter via an interneuron in the ciliary ganglion. Circadian rhythms are timed by a retinal projection to the suprachiasmatic nucleus. Visual orientation and eye movements are served by retinal input to the superior colliculus. Gaze stabilization and optokinetic reflexes are governed by a group of small retinal targets known collectively as the brainstem accessory optic system.
The eyes must be rotated constantly within their orbits to place and maintain targets of visual interest on the fovea. This activity, called foveation, or looking, is governed by an elaborate efferent motor system. Each eye is moved by six extraocular muscles that are supplied by cranial nerves from the oculomotor (III), trochlear (IV), and abducens (VI) nuclei. Activity in these ocular motor nuclei is coordinated by pontine and midbrain mechanisms for smooth pursuit, saccades, and gaze stabilization during head and body movements. Large regions of the frontal and parietooccipital cortex control these brainstem eye movement centers by providing descending supranuclear input.
In approaching a patient with reduced vision, the first step is to decide whether refractive error is responsible. In emmetropia, parallel rays from infinity are focused perfectly on the retina. Sadly, this condition is enjoyed by only a minority of the population. In myopia, the globe is too long, and light rays come to a focal point in front of the retina. Near objects can be seen clearly, but distant objects require a diverging lens in front of the eye. In hyperopia, the globe is too short, and hence a converging lens is used to supplement the refractive power of the eye. In astigmatism, the corneal surface is not perfectly spherical, necessitating a cylindrical corrective lens. As an alternative to eyeglasses or contact lenses, refractive error can be corrected by performing laser in situ keratomileusis (LASIK) or photorefractive keratectomy (PRK) to alter the curvature of the cornea.
With the onset of middle age, presbyopia develops as the lens within the eye becomes unable to increase its refractive power to accommodate on near objects. To compensate for presbyopia an emmetropic patient must use reading glasses. A patient already wearing glasses for distance correction usually switches to bifocals. The only exception is a myopic patient, who may achieve clear vision at near simply by removing glasses containing the distance prescription.
Refractive errors usually develop slowly and remain stable after adolescence, except in unusual circumstances. For example, the acute onset of diabetes mellitus can produce sudden myopia because of lens edema induced by hyperglycemia. Testing vision through a pinhole aperture is a useful way to screen quickly for refractive error. If visual acuity is better through a pinhole than it is with the unaided eye, the patient needs refraction to obtain best corrected visual acuity.
The Snellen chart is used to test acuity at a distance of 6 m (20 ft). For convenience, a scale version of the Snellen chart called the Rosenbaum card is held at 36 cm (14 in.) from the patient (Fig. 25-1). All subjects should be able to read the 6/6 m (20/20 ft) line with each eye using their refractive correction, if any. Patients who need reading glasses because of presbyopia must wear them for accurate testing with the Rosenbaum card. If 6/6 (20/20) acuity is not present in each eye, the deficiency in vision must be explained. If it is worse than 6/240 (20/800), acuity should be recorded in terms of counting fingers, hand motions, light perception, or no light perception. Legal blindness is defined by the Internal Revenue Service as a best corrected acuity of 6/60 (20/200) or less in the better eye or a binocular visual field subtending 20° or less. For driving the laws vary by state, but most states require a corrected acuity of 6/12 (20/40) in at least one eye for unrestricted privileges. Patients with a homonymous hemianopia should not drive.
FIGURE 25-1
The Rosenbaum card is a miniature, scale version of the Snellen chart for testing visual acuity at near. When the visual acuity is recorded, the Snellen distance equivalent should bear a notation indicating that vision was tested at near, not at 6 m (20 ft), or else the Jaeger number system should be used to report the acuity.

The pupils should be tested individually in dim light with the patient fixating on a distant target. There is no need to check the near response if the pupils respond briskly to light, because isolated loss of constriction (miosis) to accommodation does not occur. For this reason, the ubiquitous abbreviation PERRLA (pupils equal, round, and reactive to light and accommodation) implies a wasted effort with the last step. However, it is important to test the near response if the light response is poor or absent. Light-near dissociation occurs with neurosyphilis (Argyll Robertson pupil), with lesions of the dorsal midbrain (Parinaud’s syndrome), and after aberrant regeneration (oculomotor nerve palsy, Adie’s tonic pupil).
An eye with no light perception has no pupillary response to direct light stimulation. If the retina or optic nerve is only partially injured, the direct pupillary response will be weaker than the consensual pupillary response evoked by shining a light into the healthy fellow eye. A relative afferent pupillary defect (Marcus Gunn pupil) can be elicited with the swinging flashlight test (Fig. 25-2). It is an extremely useful sign in retrobulbar optic neuritis and other optic nerve diseases, in which it may be the sole objective evidence for disease. In bilateral optic neuropathy, no afferent pupil defect is present if the optic nerves are affected equally.
FIGURE 25-2
Demonstration of a relative afferent pupil defect (Marcus Gunn pupil) in the left eye, done with the patient fixating on a distant target. A. With dim background lighting, the pupils are equal and relatively large. B. Shining a flashlight into the right eye evokes equal, strong constriction of both pupils. C. Swinging the flashlight over to the damaged left eye causes dilation of both pupils, although they remain smaller than in A. Swinging the flashlight back over to the healthy right eye would result in symmetric constriction back to the appearance shown in B. Note that the pupils always remain equal; the damage to the left retina/optic nerve is revealed by weaker bilateral pupil constriction to a flashlight in the left eye compared with the right eye. (From P Levatin: Arch Ophthalmol 62:768, 1959. Copyright © 1959 American Medical Association. All rights reserved.)

Subtle inequality in pupil size, up to 0.5 mm, is a fairly common finding in normal persons. The diagnosis of essential or physiologic anisocoria is secure as long as the relative pupil asymmetry remains constant as ambient lighting varies. Anisocoria that increases in dim light indicates a sympathetic paresis of the iris dilator muscle. The triad of miosis with ipsilateral ptosis and anhidrosis constitutes Horner’s syndrome, although anhidrosis is an inconstant feature. Brainstem stroke, carotid dissection, and neoplasm impinging on the sympathetic chain occasionally are identified as the cause of Horner’s syndrome, but most cases are idiopathic.
Anisocoria that increases in bright light suggests a parasympathetic palsy. The first concern is an oculomotor nerve paresis. This possibility is excluded if the eye movements are full and the patient has no ptosis or diplopia. Acute pupillary dilation (mydriasis) can result from damage to the ciliary ganglion in the orbit. Common mechanisms are infection (herpes zoster, influenza), trauma (blunt, penetrating, surgical), and ischemia (diabetes, temporal arteritis). After denervation of the iris sphincter the pupil does not respond well to light, but the response to near is often relatively intact. When the near stimulus is removed, the pupil redilates very slowly compared with the normal pupil, hence the term tonic pupil. In Adie’s syndrome a tonic pupil is present, sometimes in conjunction with weak or absent tendon reflexes in the lower extremities. This benign disorder, which occurs predominantly in healthy young women, is assumed to represent a mild dysautonomia. Tonic pupils are also associated with Shy-Drager syndrome, segmental hypohidrosis, diabetes, and amyloidosis. Occasionally, a tonic pupil is discovered incidentally in an otherwise completely normal, asymptomatic individual. The diagnosis is confirmed by placing a drop of dilute (0.125%) pilocarpine into each eye. Denervation hypersensitivity produces pupillary constriction in a tonic pupil, whereas the normal pupil shows no response. Pharmacologic dilatation from accidental or deliberate instillation of anticholinergic agents (atropine, scopolamine drops) into the eye also can produce pupillary mydriasis. In this situation, normal strength (1%) pilocarpine causes no constriction.
Both pupils are affected equally by systemic medications. They are small with narcotic use (morphine, heroin) and large with anticholinergics (scopolamine). Parasympathetic agents (pilocarpine, demecarium bromide) used to treat glaucoma produce miosis. In any patient with an unexplained pupillary abnormality, a slit-lamp examination is helpful to exclude surgical trauma to the iris, an occult foreign body, perforating injury, intraocular inflammation, adhesions (synechia), angle-closure glaucoma, and iris sphincter rupture from blunt trauma.
Eye movements are tested by asking the patient, with both eyes open, to pursue a small target such as a penlight into the cardinal fields of gaze. Normal ocular versions are smooth, symmetric, full, and maintained in all directions without nystagmus. Saccades, or quick refixation eye movements, are assessed by having the patient look back and forth between two stationary targets. The eyes should move rapidly and accurately in a single jump to their target. Ocular alignment can be judged by holding a penlight directly in front of the patient at about 1 m. If the eyes are straight, the corneal light reflex will be centered in the middle of each pupil. To test eye alignment more precisely, the cover test is useful. The patient is instructed to look at a small fixation target in the distance. One eye is covered suddenly while the second eye is observed. If the second eye shifts to fixate on the target, it was misaligned. If it does not move, the first eye is uncovered and the test is repeated on the second eye. If neither eye moves the eyes are aligned orthotropically. If the eyes are orthotropic in primary gaze but the patient complains of diplopia, the cover test should be performed with the head tilted or turned in whatever direction elicits diplopia. With practice, the examiner can detect an ocular deviation (heterotropia) as small as 1–2° with the cover test. In a patient with vertical diplopia, a small deviation can be difficult to detect and easy to dismiss. The magnitude of the deviation can be measured by placing a prism in front of the misaligned eye to determine the power required to neutralize the fixation shift evoked by covering the other eye. Temporary press-on plastic Fresnel prisms, prism eyeglasses, or eye muscle surgery can be used to restore binocular alignment.
Stereoacuity is determined by presenting targets with retinal disparity separately to each eye by using polarized images. The most popular office tests measure a range of thresholds from 800–40 seconds of arc. Normal stereoacuity is 40 seconds of arc. If a patient achieves this level of stereoacuity, one is assured that the eyes are aligned orthotropically and that vision is intact in each eye. Random dot stereograms have no monocular depth cues and provide an excellent screening test for strabismus and amblyopia in children.
The retina contains three classes of cones, with visual pigments of differing peak spectral sensitivity: red (560 nm), green (530 nm), and blue (430 nm). The red and green cone pigments are encoded on the X chromosome, and the blue cone pigment on chromosome 7. Mutations of the blue cone pigment are exceedingly rare. Mutations of the red and green pigments cause congenital X-linked color blindness in 8% of males. Affected individuals are not truly color blind; rather, they differ from normal subjects in the way they perceive color and how they combine primary monochromatic lights to match a particular color. Anomalous trichromats have three cone types, but a mutation in one cone pigment (usually red or green) causes a shift in peak spectral sensitivity, altering the proportion of primary colors required to achieve a color match. Dichromats have only two cone types and therefore will accept a color match based on only two primary colors. Anomalous trichromats and dichromats have 6/6 (20/20) visual acuity, but their hue discrimination is impaired. Ishihara color plates can be used to detect red-green color blindness. The test plates contain a hidden number that is visible only to subjects with color confusion from red-green color blindness. Because color blindness is almost exclusively X-linked, it is worth screening only male children.
The Ishihara plates often are used to detect acquired defects in color vision, although they are intended as a screening test for congenital color blindness. Acquired defects in color vision frequently result from disease of the macula or optic nerve. For example, patients with a history of optic neuritis often complain of color desaturation long after their visual acuity has returned to normal. Color blindness also can result from bilateral strokes involving the ventral portion of the occipital lobe (cerebral achromatopsia). Such patients can perceive only shades of gray and also may have difficulty recognizing faces (prosopagnosia). Infarcts of the dominant occipital lobe sometimes give rise to color anomia. Affected patients can discriminate colors but cannot name them.
Vision can be impaired by damage to the visual system anywhere from the eyes to the occipital lobes. One can localize the site of the lesion with considerable accuracy by mapping the visual field deficit by finger confrontation and then correlating it with the topographic anatomy of the visual pathway (Fig. 25-3). Quantitative visual field mapping is performed by computer-driven perimeters that present a target of variable intensity at fixed positions in the visual field (Fig. 25-3A). By generating an automated printout of light thresholds, these static perimeters provide a sensitive means of detecting scotomas in the visual field. They are exceedingly useful for serial assessment of visual function in chronic diseases such as glaucoma and pseudotumor cerebri.
FIGURE 25-3
Ventral view of the brain, correlating patterns of visual field loss with the sites of lesions in the visual pathway. The visual fields overlap partially, creating 120° of central binocular field flanked by a 40° monocular crescent on either side. The visual field maps in this figure were done with a computer-driven perimeter (Humphrey Instruments, Carl Zeiss, Inc.). It plots the retinal sensitivity to light in the central 30° by using a gray scale format. Areas of visual field loss are shown in black. The examples of common monocular, prechiasmal field defects are all shown for the right eye. By convention, the visual fields are always recorded with the left eye’s field on the left and the right eye’s field on the right, just as the patient sees the world.

The crux of visual field analysis is to decide whether a lesion is before, at, or behind the optic chiasm. If a scotoma is confined to one eye, it must be due to a lesion anterior to the chiasm, involving either the optic nerve or the retina. Retinal lesions produce scotomas that correspond optically to their location in the fundus. For example, a superior-nasal retinal detachment results in an inferior-temporal field cut. Damage to the macula causes a central scotoma (Fig. 25-3B).
Optic nerve disease produces characteristic patterns of visual field loss. Glaucoma selectively destroys axons that enter the superotemporal or inferotemporal poles of the optic disc, resulting in arcuate scotomas shaped like a Turkish scimitar, which emanate from the blind spot and curve around fixation to end flat against the horizontal meridian (Fig. 25-3C). This type of field defect mirrors the arrangement of the nerve fiber layer in the temporal retina. Arcuate or nerve fiber layer scotomas also result from optic neuritis, ischemic optic neuropathy, optic disc drusen, and branch retinal artery or vein occlusion.
Damage to the entire upper or lower pole of the optic disc causes an altitudinal field cut that follows the horizontal meridian (Fig. 25-3D). This pattern of visual field loss is typical of ischemic optic neuropathy but also results from retinal vascular occlusion, advanced glaucoma, and optic neuritis.
About half the fibers in the optic nerve originate from ganglion cells serving the macula. Damage to papillomacular fibers causes a cecocentral scotoma that encompasses the blind spot and macula (Fig. 25-3E). If the damage is irreversible, pallor eventually appears in the temporal portion of the optic disc. Temporal pallor from a cecocentral scotoma may develop in optic neuritis, nutritional optic neuropathy, toxic optic neuropathy, Leber’s hereditary optic neuropathy, Kjer’s dominant optic atrophy, and compressive optic neuropathy. It is worth mentioning that the temporal side of the optic disc is slightly paler than the nasal side in most normal individuals. Therefore, it sometimes can be difficult to decide whether the temporal pallor visible on fundus examination represents a pathologic change. Pallor of the nasal rim of the optic disc is a less equivocal sign of optic atrophy.
At the optic chiasm, fibers from nasal ganglion cells decussate into the contralateral optic tract. Crossed fibers are damaged more by compression than are uncrossed fibers. As a result, mass lesions of the sellar region cause a temporal hemianopia in each eye. Tumors anterior to the optic chiasm, such as meningiomas of the tuberculum sella, produce a junctional scotoma characterized by an optic neuropathy in one eye and a superior-temporal field cut in the other eye (Fig. 25-3G). More symmetric compression of the optic chiasm by a pituitary adenoma (see Fig. 51-1), meningioma, craniopharyngioma, glioma, or aneurysm results in a bitemporal hemianopia (Fig. 25-3H). The insidious development of a bitemporal hemianopia often goes unnoticed by the patient and will escape detection by the physician unless each eye is tested separately.
It is difficult to localize a postchiasmal lesion accurately, because injury anywhere in the optic tract, lateral geniculate body, optic radiations, or visual cortex can produce a homonymous hemianopia (i.e., a temporal hemifield defect in the contralateral eye and a matching nasal hemifield defect in the ipsilateral eye) (Fig. 25-3I). A unilateral postchiasmal lesion leaves the visual acuity in each eye unaffected, although the patient may read the letters on only the left or right half of the eye chart. Lesions of the optic radiations tend to cause poorly matched or incongruous field defects in each eye. Damage to the optic radiations in the temporal lobe (Meyer’s loop) produces a superior quadrantic homonymous hemianopia (Fig. 25-3J), whereas injury to the optic radiations in the parietal lobe results in an inferior quadrantic homonymous hemianopia (Fig. 25-3K). Lesions of the primary visual cortex give rise to dense, congruous hemianopic field defects. Occlusion of the posterior cerebral artery supplying the occipital lobe is a common cause of total homonymous hemianopia. Some patients with hemianopia after occipital stroke have macular sparing, because the macular representation at the tip of the occipital lobe is supplied by collaterals from the middle cerebral artery (Fig. 25-3L). Destruction of both occipital lobes produces cortical blindness. This condition can be distinguished from bilateral prechiasmal visual loss by noting that the pupil responses and optic fundi remain normal.
Corneal abrasions are seen best by placing a drop of fluorescein in the eye and looking with the slit lamp, using a cobalt-blue light. A penlight with a blue filter will suffice if a slit lamp is not available. Damage to the corneal epithelium is revealed by yellow fluorescence of the exposed basement membrane underlying the epithelium. It is important to check for foreign bodies. To search the conjunctival fornices, the lower lid should be pulled down and the upper lid everted. A foreign body can be removed with a moistened cotton-tipped applicator after a drop of a topical anesthetic such as proparacaine has been placed in the eye. Alternatively, it may be possible to flush the foreign body from the eye by irrigating copiously with saline or artificial tears. If the corneal epithelium has been abraded, antibiotic ointment and a patch should be applied to the eye. A drop of an intermediate-acting cycloplegic such as cyclopentolate hydrochloride 1% helps reduce pain by relaxing the ciliary body. The eye should be reexamined the next day. Minor abrasions may not require patching, antibiotics, or cycloplegia.
This results from rupture of small vessels bridging the potential space between the episclera and the conjunctiva. Blood dissecting into this space can produce a spectacular red eye, but vision is not affected and the hemorrhage resolves without treatment. Subconjunctival hemorrhage is usually spontaneous but can result from blunt trauma, eye rubbing, or vigorous coughing. Occasionally it is a clue to an underlying bleeding disorder.
Pinguecula is a small, raised conjunctival nodule at the temporal or nasal limbus. In adults such lesions are extremely common and have little significance unless they become inflamed (pingueculitis). They are more apt to occur in workers with frequent outdoor exposure. A pterygium resembles a pinguecula but has crossed the limbus to encroach on the corneal surface. Removal is justified when symptoms of irritation or blurring develop, but recurrence is a common problem.
This refers to inflammation of the eyelids. The most common form occurs in association with acne rosacea or seborrheic dermatitis. The eyelid margins usually are colonized heavily by staphylococci. Upon close inspection, they appear greasy, ulcerated, and crusted with scaling debris that clings to the lashes. Treatment consists of strict eyelid hygiene, using warm compresses and eyelash scrubs with baby shampoo. An external hordeolum (sty) is caused by staphylococcal infection of the superficial accessory glands of Zeis or Moll located in the eyelid margins. An internal hordeolum occurs after suppurative infection of the oil-secreting meibomian glands within the tarsal plate of the eyelid. Topical antibiotics such as bacitracin/polymyxin B ophthalmic ointment can be applied. Systemic antibiotics, usually tetracyclines or azithromycin, sometimes are necessary for treatment of meibomian gland inflammation (meibomitis) or chronic, severe blepharitis. A chalazion is a painless, chronic granulomatous inflammation of a meibomian gland that produces a pealike nodule within the eyelid. It can be incised and drained or injected with glucocorticoids. Basal cell, squamous cell, or meibomian gland carcinoma should be suspected with any nonhealing ulcerative lesion of the eyelids.
An inflammation of the lacrimal drainage system, dacryocystitis can produce epiphora (tearing) and ocular injection. Gentle pressure over the lacrimal sac evokes pain and reflux of mucus or pus from the tear puncta. Dacryocystitis usually occurs after obstruction of the lacrimal system. It is treated with topical and systemic antibiotics, followed by probing, silicone stent intubation, or surgery to reestablish patency. Entropion (inversion of the eyelid) or ectropion (sagging or eversion of the eyelid) can also lead to epiphora and ocular irritation.
Conjunctivitis is the most common cause of a red, irritated eye. Pain is minimal, and visual acuity is reduced only slightly. The most common viral etiology is adenovirus infection. It causes a watery discharge, a mild foreign-body sensation, and photophobia. Bacterial infection tends to produce a more mucopurulent exudate. Mild cases of infectious conjunctivitis usually are treated empirically with broad-spectrum topical ocular antibiotics such as sulfacetamide 10%, polymyxin-bacitracin, or a trimethoprim-polymyxin combination. Smears and cultures usually are reserved for severe, resistant, or recurrent cases of conjunctivitis. To prevent contagion, patients should be admonished to wash their hands frequently, not to touch their eyes, and to avoid direct contact with others.
This condition is extremely common and often is mistaken for infectious conjunctivitis. Itching, redness, and epiphora are typical. The palpebral conjunctiva may become hypertropic with giant excrescences called cobblestone papillae. Irritation from contact lenses or any chronic foreign body also can induce formation of cobblestone papillae. Atopic conjunctivitis occurs in subjects with atopic dermatitis or asthma. Symptoms caused by allergic conjunctivitis can be alleviated with cold compresses, topical vasoconstrictors, antihistamines, and mast cell stabilizers such as cromolyn sodium. Topical glucocorticoid solutions provide dramatic relief of immune-mediated forms of conjunctivitis, but their long-term use is ill advised because of the complications of glaucoma, cataract, and secondary infection. Topical nonsteroidal anti-inflammatory drugs (NSAIDs) (e.g., ketorolac tromethamine) are better alternatives.
Also known as dry eye, this produces a burning foreign-body sensation, injection, and photophobia. In mild cases the eye appears surprisingly normal, but tear production measured by wetting of a filter paper (Schirmer strip) is deficient. A variety of systemic drugs, including antihistaminic, anticholinergic, and psychotropic medications, result in dry eye by reducing lacrimal secretion. Disorders that involve the lacrimal gland directly, such as sarcoidosis and Sjögren’s syndrome, also cause dry eye. Patients may develop dry eye after radiation therapy if the treatment field includes the orbits. Problems with ocular drying are also common after lesions affecting cranial nerve V or VII. Corneal anesthesia is particularly dangerous, because the absence of a normal blink reflex exposes the cornea to injury without pain to warn the patient. Dry eye is managed by frequent and liberal application of artificial tears and ocular lubricants. In severe cases the tear puncta can be plugged or cauterized to reduce lacrimal outflow.
Keratitis is a threat to vision because of the risk of corneal clouding, scarring, and perforation. Worldwide, the two leading causes of blindness from keratitis are trachoma from chlamydial infection and vitamin A deficiency related to malnutrition. In the United States, contact lenses play a major role in corneal infection and ulceration. They should not be worn by anyone with an active eye infection. In evaluating the cornea, it is important to differentiate between a superficial infection (keratoconjunctivitis) and a deeper, more serious ulcerative process. The latter is accompanied by greater visual loss, pain, photophobia, redness, and discharge. Slit-lamp examination shows disruption of the corneal epithelium, a cloudy infiltrate or abscess in the stroma, and an inflammatory cellular reaction in the anterior chamber. In severe cases, pus settles at the bottom of the anterior chamber, giving rise to a hypopyon. Immediate empirical antibiotic therapy should be initiated after corneal scrapings are obtained for Gram’s stain, Giemsa stain, and cultures. Fortified topical antibiotics are most effective, supplemented with subconjunctival antibiotics as required. A fungal etiology should always be considered in a patient with keratitis. Fungal infection is common in warm humid climates, especially after penetration of the cornea by plant or vegetable material.
The herpesviruses are a major cause of blindness from keratitis. Most adults in the United States have serum antibodies to herpes simplex, indicating prior viral infection. Primary ocular infection generally is caused by herpes simplex type 1 rather than type 2. It manifests as a unilateral follicular blepharoconjunctivitis that is easily confused with adenoviral conjunctivitis unless telltale vesicles appear on the periocular skin or conjunctiva. A dendritic pattern of corneal epithelial ulceration revealed by fluorescein staining is pathognomonic for herpes infection but is seen in only a minority of primary infections. Recurrent ocular infection arises from reactivation of the latent herpesvirus. Viral eruption in the corneal epithelium may result in the characteristic herpes dendrite. Involvement of the corneal stroma produces edema, vascularization, and iridocyclitis. Herpes keratitis is treated with topical antiviral agents, cycloplegics, and oral acyclovir. Topical glucocorticoids are effective in mitigating corneal scarring but must be used with extreme caution because of the danger of corneal melting and perforation. Topical glucocorticoids also carry the risk of prolonging infection and inducing glaucoma.
Herpes zoster from reactivation of latent varicella (chickenpox) virus causes a dermatomal pattern of painful vesicular dermatitis. Ocular symptoms can occur after zoster eruption in any branch of the trigeminal nerve but are particularly common when vesicles form on the nose, reflecting nasociliary (V1) nerve involvement (Hutchinson’s sign). Herpes zoster ophthalmicus produces corneal dendrites, which can be difficult to distinguish from those seen in herpes simplex. Stromal keratitis, anterior uveitis, raised intraocular pressure, ocular motor nerve palsies, acute retinal necrosis, and postherpetic scarring and neuralgia are other common sequelae. Herpes zoster ophthalmicus is treated with antiviral agents and cycloplegics. In severe cases, glucocorticoids may be added to prevent permanent visual loss from corneal scarring.
This is an inflammation of the episclera, a thin layer of connective tissue between the conjunctiva and the sclera. Episcleritis resembles conjunctivitis, but it is a more localized process and discharge is absent. Most cases of episcleritis are idiopathic, but some occur in the setting of an autoimmune disease. Scleritis refers to a deeper, more severe inflammatory process that frequently is associated with a connective tissue disease such as rheumatoid arthritis, lupus erythematosus, polyarteritis nodosa, granulomatosis with polyangiitis (Wegener’s), or relapsing polychondritis. The inflammation and thickening of the sclera can be diffuse or nodular. In anterior forms of scleritis, the globe assumes a violet hue and the patient complains of severe ocular tenderness and pain. With posterior scleritis, the pain and redness may be less marked, but there is often proptosis, choroidal effusion, reduced motility, and visual loss. Episcleritis and scleritis should be treated with NSAIDs. If these agents fail, topical or even systemic glucocorticoid therapy may be necessary, especially if an underlying autoimmune process is active.
Involving the anterior structures of the eye, uveitis also is called iritis or iridocyclitis. The diagnosis requires slit-lamp examination to identify inflammatory cells floating in the aqueous humor or deposited on the corneal endothelium (keratic precipitates). Anterior uveitis develops in sarcoidosis, ankylosing spondylitis, juvenile rheumatoid arthritis, inflammatory bowel disease, psoriasis, reactive arthritis, and Behçet’s disease. It also is associated with herpes infections, syphilis, Lyme disease, onchocerciasis, tuberculosis, and leprosy. Although anterior uveitis can occur in conjunction with many diseases, no cause is found to explain the majority of cases. For this reason, laboratory evaluation usually is reserved for patients with recurrent or severe anterior uveitis. Treatment is aimed at reducing inflammation and scarring by judicious use of topical glucocorticoids. Dilatation of the pupil reduces pain and prevents the formation of synechiae.
This is diagnosed by observing inflammation of the vitreous, retina, or choroid on fundus examination. It is more likely than anterior uveitis to be associated with an identifiable systemic disease. Some patients have panuveitis, or inflammation of both the anterior and posterior segments of the eye. Posterior uveitis is a manifestation of autoimmune diseases such as sarcoidosis, Behçet’s disease, Vogt-Koyanagi-Harada syndrome, and inflammatory bowel disease. It also accompanies diseases such as toxoplasmosis, onchocerciasis, cysticercosis, coccidioidomycosis, toxocariasis, and histoplasmosis; infections caused by organisms such as Candida, Pneumocystis carinii, Cryptococcus, Aspergillus, herpes, and cytomegalovirus; and other diseases, such as syphilis, Lyme disease, tuberculosis, cat-scratch disease, Whipple’s disease, and brucellosis. In multiple sclerosis, chronic inflammatory changes can develop in the extreme periphery of the retina (pars planitis or intermediate uveitis).
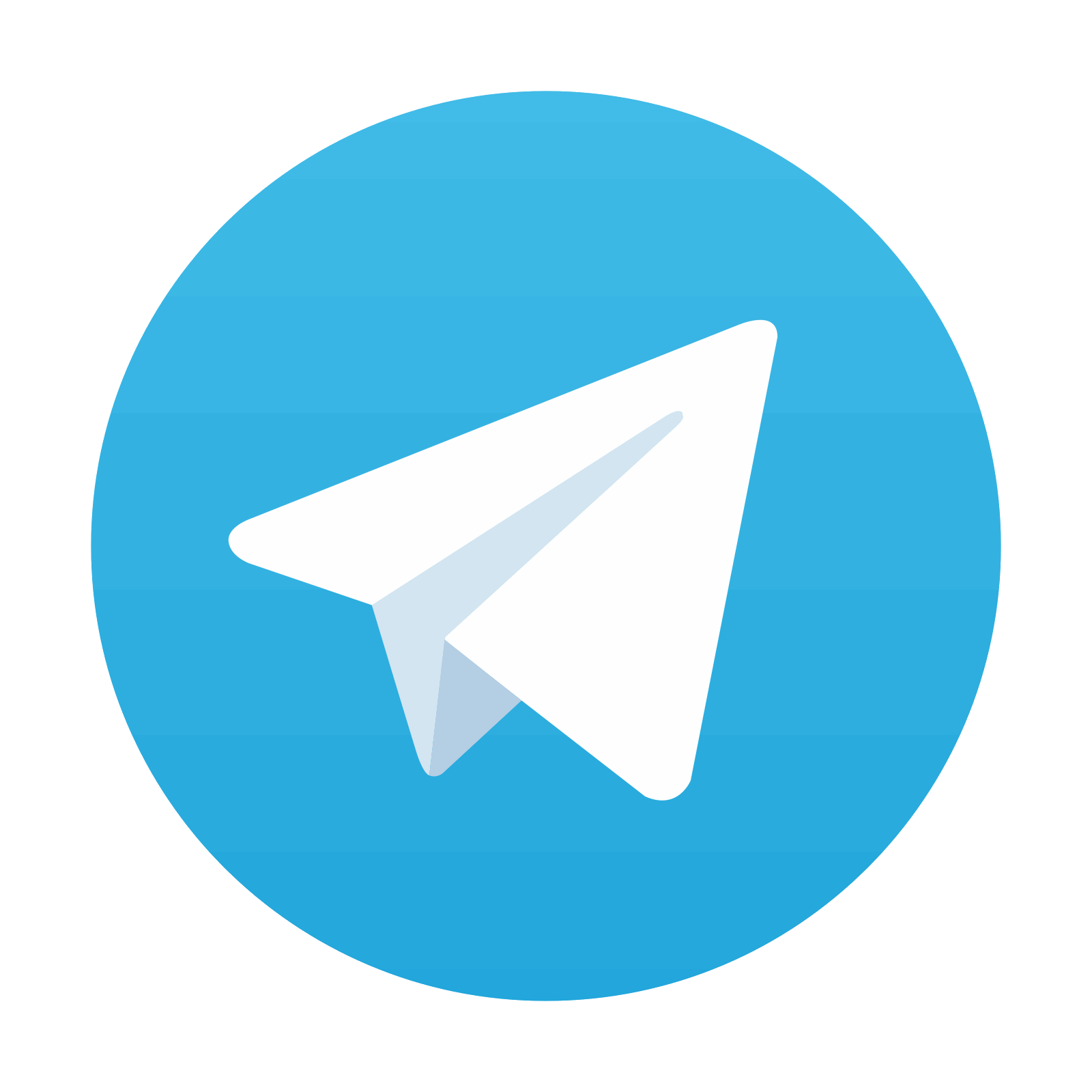
Stay updated, free articles. Join our Telegram channel

Full access? Get Clinical Tree
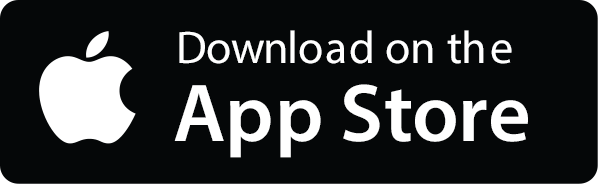
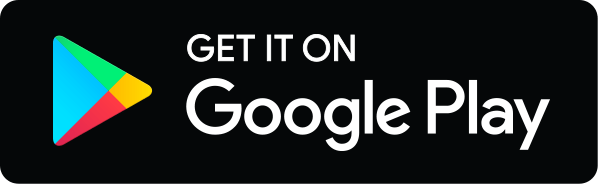