Critically ill patients are prone to a wide variety of fluid, electrolyte, and acid-base disorders. Two of those disorders are of particular relevance to patients with critical neurological illness: hypo- and hypernatremia. These are by far the most common electrolyte abnormalities in patients with central nervous system disease and have the most pressing diagnostic and therapeutic implications. This chapter therefore focuses exclusively on these disorders. Interested readers will find a recent, more comprehensive discussion of fluid, electrolyte, and acid-base abnormalities in critically ill patients elsewhere.1
Hypo- and hypernatremia are manifestations of impaired water homeostasis. They occur frequently in patients with central nervous system disease2 and are associated with increased morbidity and mortality.3,4 Since sodium is the predominant solute in the extracellular fluid, changes in plasma sodium concentration (PNa) usually correlate with changes in extracellular fluid osmolality (measured as plasma osmolality [Posm]). (Some important exceptions to the correlation between hyponatremia and hypo-osmolality are discussed below.) The changes in Posm, in turn, are associated with changes in cell volume, because of the osmotic effect on water movement across the cell membrane: Hypo-osmolality is associated with cell swelling, and hyperosmolality with cell shrinkage. These alterations in cell volume are of particular concern in the brain, because of its enclosure in the rigid calcavarium. To properly diagnose and treat disorders of water homeostasis, it is important for clinicians to understand their pathophysiology.
Plasma sodium concentration (PNa) normally varies very little. This tight regulation of the PNa depends on the following elements: (1) modulation of pituitary secretion of arginine vasopressin (AVP; also known as antidiuretic hormone [ADH]) over a wide range in response to physiologic stimuli; (2) kidneys that are capable of responding to circulating AVP by varying the urine concentration; (3) intact thirst; and (4) access to water.
The normal response to water ingestion (of sufficient magnitude to lower the plasma osmolality even slightly) is the excretion of maximally dilute urine (urine osmolality < 100 mOsm/kg). The underlying physiologic sequence is as follows: The plasma hypo-osmolality is sensed by the cells comprising the hypothalamic osmostat. These hypothalamic nuclei then proportionately reduce their synthesis of AVP, leading to diminished AVP release into the circulation by the posterior pituitary. The lower circulating AVP concentration causes less vasopressin-receptor type 2 (V2 receptor) stimulation of the epithelial cells lining the renal collecting duct. This, in turn, results in the insertion of proportionately fewer water channels into the collecting duct, creating a more water-impermeable conduit, which allows excretion of the dilute urine elaborated by the more proximal segments of the nephron.5
Conversely, plasma hyperosmolality leads to higher circulating AVP concentration and proportionately higher water permeability of the collecting duct, and the excretion of a concentrated urine.5
Figure 49-1 shows the relationship between plasma osmolality, plasma AVP concentration, and urine osmolality. The normal “set point” is a plasma osmolality of about 285 mOsm/kg. Notice that the minimum achievable urine osmolality is about 50 mOsm/kg, and the maximum about 1200 mOsm/kg.6
When plasma osmolality rises beyond 290 to 295 mOsm/kg, the thirst center of the hypothalamus is stimulated. At that point, neurologically intact individuals with access to water will drink until the plasma osmolality returns to normal.6
It is important to recognize that plasma osmolality is not the only determinant of AVP synthesis and release. Low arterial blood pressure and low effective arterial volume powerfully stimulate AVP release.6 This baroreceptor-mediated AVP release is teleological, since water retention is an important component in the defense against hypovolemia. So primal is this circulatory defense that the baroreceptor stimulation predominates over any osmolal effect on AVP release.6 Thus, a volume-contracted or hypotensive individual will have high circulating AVP levels and excrete a concentrated urine, even if the plasma osmolality is low. In addition, circulating AVP levels rise with pain, stress, nausea, hypoxia, hypercapnia, and a variety of medications.6
A 55-year-old man is admitted to the intensive care unit with a subarachnoid hemorrhage (SAH). The PNa is 141 mmol/L. The patient is intubated and mechanically ventilated. The initial increase in intracranial pressure is effectively controlled (see Chapter 1, Subarachnoid Hemorrhage). On the fifth hospital day, he is extubated. The PNa is noted to have fallen to 134 mmol/L. The following day, it is 128 mmol/L.
Hyponatremia (PNa < 135 mmol/L) is seen in approximately 3% of hospitalized patients and as many as 30% of patients in intensive care units.3 Among neurosurgical patients, the incidence of significant hyponatremia (PNa < 130 mmol/L) is about 14%, with SAH patients having the highest incidence (almost 20%).2 The most important clinical manifestations of hypo-osmolal hyponatremia are caused by cerebral edema from osmotically induced water movement into brain cells. As noted above, however, not all hyponatremia is associated with hypo-osmolality. Hyponatremia may coexist with a normal, high or low plasma osmolality.
Isotonic hyponatremia (also known as factitious or pseudohyponatremia) is a laboratory artifact seen in the setting of marked hypertriglyceridemia or paraproteinemia, when the sodium measurement method involves a predilution step.7 The hyponatremia per se is of no clinical importance except as a clue to its pathogenesis.
Hypertonic hyponatremia results from the presence in extracellular fluid of abnormal amounts of osmotically effective solutes other than sodium (eg, glucose, mannitol, or glycerol). The osmotic pressure exerted by the nonsodium solute leads to redistribution of water from the intracellular to the extracellular fluid compartment, resulting in dilution of the extracellular sodium mass and resultant hyponatremia. The hyponatremia is real (not pseudo-), but it is accompanied by hypertonicity and a decrease in cell volume due to cellular dehydration, rather than cerebral edema.
Hypotonic hyponatremia, by far the most common type of hyponatremia, is caused by an inability of the kidney to excrete sufficient electrolyte-free water to match water intake. It is this form of hyponatremia in which the hyponatremia itself is of clinical concern.
Based on this differential diagnosis, the diagnostic algorithm for hyponatremia begins (Figure 49-2) with an assessment of the plasma osmolality (Posm). This may be estimated by the following formula, based on commonly measured plasma solutes:
where Pgluc is the plasma glucose concentration and BUN is blood urea nitrogen concentration, both in mg/dL. If there is a suspicion that an unmeasured osmotically effective solute may be present in the plasma (eg, mannitol or glycerol), the Posm should be measured directly.
The glucose concentration is 101 mg/dL and the BUN 14 mg/dL. The patient had never received mannitol or glycerol. Thus, the estimated Posm is 267 mOsm/kg. To be sure, the patient’s physician repeats the basic chemistry panel and measured the Posm. The PNa is now 125 mmol/L and the Posm is 261 mOsm/kg.
The normal Posm is 280 to 285 mOsm/kg. This patient thus has hypotonic hyponatremia. This may occur either because the normal diluting capacity of the kidney is overwhelmed by excessive water intake or because the diluting capacity of the kidney is impaired. The former condition is characterized by excretion of a maximally dilute urine (Uosm < 100 mOsm/kg H2O), and the latter is not. The classic example of the former condition is primary polydipsia, seen most commonly in patients with schizophrenia.8 There are no reported cases of primary polydipsia in association with stroke, either hemorrhagic or ischemic.
The Uosm is 450 mOsm/kg, and the urine electrolytes (in mmol/L) are as follows: Na 180, K 40, and Cl 220.
This patient clearly has impaired urinary diluting capacity. The concentrated urine usually reflects a high circulating AVP level, which is clearly inappropriate for the Posm (Figure 49-1). However, because AVP release is affected by systemic hemodynamics as well as osmolality (see above), assessment of the patient’s extracellular fluid volume status and hemodynamics is crucial at this juncture (Figure 49-2). Patients with hyponatremia may present with normal, low, or increased extracellular fluid volume.
Patients with hyponatremia as a result of pure (electrolyte-free) water excess have ostensibly normal plasma volume status (euvolemia) because the excess water distributes throughout the total body water space; only one-third of total body water is extracellular, and only one-twelfth is intravascular. Thus, an adult patient whose total body water is expanded by 6 L would have a PNa of 122 mmol/L and will have his intravascular volume expanded by only 500 mL. The only evidence of the mild intravascular volume expansion is low BUN and plasma uric acid concentration.9
The paradigm of euvolemic hyponatremia with a concentrated urine is the syndrome of inappropriate antidiuretic hormone (SIADH). SIADH is by far the most common cause of hyponatremia in patients with neurosurgical disease, accounting for more than 70% of cases.2 It is characterized by elevated circulating AVP (ADH) levels that are inappropriate to vasopressin’s two physiologic stimuli (ie, osmotic or hemodynamic).10 Hypotonic hyponatremia in patients with SIADH develops to the extent that water ingestion exceeds water elimination by insensible (sweat and perspiration; respiratory), gastrointestinal, and renal routes. Because the normal response to extracellular hypotonicity is the elaboration of maximally dilute urine (urine osmolality < 100 mOsm/kg), the urine need only be inappropriately concentrated (ie, > 100 mOsm/kg) to be compatible with a diagnosis of SIADH.
Because hypothyroidism11 and glucocorticoid insufficiency12 may impair urinary dilution even with suppressed AVP, patients in whom a diagnosis of SIADH is entertained should undergo appropriate tests of thyroid and adrenocortical function (see Chapter 57, Nutrition and Metabolic Support.
Once a diagnosis of SIADH is made, its cause must be established: Just because a patient with SAH is found to have SIADH does not mean that the SAH is the cause. There may be another more easily remediable cause. Table 49-1 lists important causes of SIADH. They fall into five major categories: intracranial abnormalities, intrathoracic abnormalities, tumors, drugs, and idiopathic causes. Important causes of SIADH in any hospitalized patient include pain, nausea, psychological stress, and most commonly, drugs.
Intracranial abnormalities
Intrathoracic abnormalities
Drugs
Extracranial tumors
HIV/AIDS Hereditary
Miscellaneous
Ideopathic |
The urinary diluting impairment in hypovolemia is mediated both by decreased delivery of fluid to the diluting segments of the nephron and by baroreceptor stimulation of AVP release. Thus, the volume-contracted patient cannot excrete electrolyte-free water normally, and even in the face of modest water ingestion may develop hyponatremia.
The cause of the volume contraction usually is obvious (eg., hemorrhage, vomiting, diarrhea, diuretics). When it is not, the urine sodium concentration may be helpful in distinguishing between renal and extrarenal solute losses. Renal losses (eg, as a result of diuretic medications) are usually reflected by sodium wasting, and extrarenal (GI, skin, “3rd space,” or hemorrhagic) losses are usually accompanied by sodium conservation (urine sodium concentration < 10 mmol/L). Exceptions occur in the recovery phase after diuretic therapy, when the kidney has regained its capacity to respond to the volume depletion and with vomiting. In the latter situation, urinary sodium excretion is obligated by the bicarbonaturia that accompanies the vomiting-induced metabolic alkalosis. In that situation, the urine chloride concentration tends to be very low and is the best indicator of extracellular volume depletion.13,14
Renal salt wasting—in this setting, more commonly called cerebral salt wasting (CSW)—may be responsible for hypovolemic hyponatremia in some patients with intracranial pathology (eg, tumors, hemorrhage).15,16 The proposed underlying pathogenesis is a failure of the kidneys to adequately reabsorb sodium, leading to volume depletion. The mechanism of hyponatremia, then, is similar to that of other hypovolemic states. The pathogenesis of the renal salt wasting is incompletely understood: Putative mechanisms include impaired sympathetic efferent pathways to the kidneys from the damaged central nervous system and excessive secretion of a circulating natriuretic factor (eg, B-type natriuretic peptide [BNP]).17 Because of a hyponatremic syndrome in patients with central nervous system disease, CSW is difficult to distinguish from SIADH, for several reasons. First, both are characterized by high urinary sodium excretion. Second, and particularly vexing, patients who have been diagnosed with CSW have hypouricemia, in contrast to most volume-depleted patients, who have hyperuricemia. The hypouricemia is thought to reflect a generalized impairment of solute reabsorption in the proximal tubule.17 Thus, the distinction of CSW from SIADH hinges completely on documentation of volume depletion. This seemingly simple determination is in fact quite complex and fraught with error.15,18-20 Indeed, so controversial is the syndrome of CSW that some investigators think it is the most common cause of hyponatremia in patients with central nervous system disease,15,21 whereas others question its existence altogether.18-20
The hyponatremia associated with diuretic treatment is multifactorial. Insofar as diuretics produce overt volume depletion, they can cause hyponatremia by the mechanisms discussed above. Thiazide diuretics in particular have been associated with the development of acute severe, symptomatic hyponatremia, particularly in small, older women, in the absence of overt signs of volume depletion.22 The cause of the often precipitous hyponatremia remains uncertain,23 although subtle volume depletion, hypokalemia, increased thirst,24 and upregulation or renal tubule water channels25 have been implicated.
Hypervolemic hyponatremia generally is seen in patients who cannot excrete sodium normally because they have either severe renal failure26 or one of the pathologic edema-forming states (eg, congestive heart failure, hepatic cirrhosis, nephrotic syndrome).
Hyponatremia is common in the pathologic edema states, especially congestive heart failure and hepatic cirrhosis. The hormonal profile of such patients mirrors that of patients with intravascular volume depletion, even though their absolute intravascular volume typically is increased. Thus, these disorders are said to be characterized by “diminished effective circulating volume.”27 Because of the perceived intravascular volume depletion, urinary diluting capacity is compromised for reasons similar to those in hypovolemic hyponatremia.
The patient is afebrile and breathing at 18 per minute. His fluid volume status is equivocal: The blood pressure is 126/68; the heart rate is 96 at rest; there is no jugular venous distension and no dependent edema. In view of the copious urinary sodium and the possibility of subtle volume depletion, his physician entertains the diagnosis of CSW. He gives him intravenous normal saline solution at a rate of 120 mL/h. The patient is incapable of taking anything by mouth. The next day, the PNa has fallen to 118 mmol/L. The patient’s mental status, which had been improving over the past few days, has now declined.
Why did the PNa fall after infusing 3 L of normal saline solution (sodium concentration, 154 mmol/L)?
The magnitude of the decline in the patient’s PNa implies that there was a gain of electrolyte-free water of about 2 L. By definition, normal saline solution contains no electrolyte-free water. The kidney, however, is capable of generating electrolyte-free water and returning it to the circulation during a saline infusion, a process that has been called desalination.28 This happens when the patient is excreting a large amount of electrolyte in a concentrated urine (under the influence of high circulating AVP)—exactly the case with our patient. In essence, the patient receives an isotonic saline solution, excretes a hypertonic saline solution, and returns the reabsorbed water to the circulation, further diluting his plasma sodium. In our patient, this could have been predicted by the recognition that his urine electrolyte concentration greatly exceeded his plasma electrolyte concentration.29
The clinical manifestations of hyponatremia are largely attributed to intracellular volume expansion (cellular edema), which occurs only when hyponatremia is associated with hypotonicity. (Tonicity or effective osmolality describes the capacity of particles in solution to effect water movement across a semi-permeable membrane like the cell membrane.) Intracellular volume expansion is of greatest consequence in the brain, where it is translated into increased intracranial pressure because of the rigid calvarium.30
The pathophysiology of hypotonic hyponatremia has important implications for its management. Most cells—especially brain cells—have adaptive mechanisms for mitigating tonicity-related volume changes.30 Hypotonic, swollen cells begin discharging electrolyte within minutes in order to mitigate cellular edema. Additional small solutes are then extruded, such that after several days of sustained hypotonicity, cell volume is restored to normal.30
The morbidity and mortality associated with hypotonic hyponatremia are influenced by several factors, the most important of which is the rate at which the Posm falls.30 Hyponatremia that develops in less than 48 hours can overwhelm the brain’s volume-adaptive mechanisms and may precipitate catastrophic cerebral edema.31 Other factors that influence morbidity and mortality with hyponatremia include the magnitude of the hyponatremia, the patient’s age and gender, and the nature and severity of any underlying diseases.30 The very young and the very old, women, and alcoholics appear to be at particular risk.32 Cell-volume adaptation to hypotonicity may be deficient in premenopausal women, who suffer more frequent and more severe neurologic consequences than men with equivalent degrees of hypotonicity.33
In patients with acute hyponatremia, neurologic symptoms usually do not occur until the PNa falls below 125 mmol/L, although death from acute hyponatremia has been reported at 128 mmol/L.34 The earliest symptoms include anorexia, nausea, and malaise. Between 120 and 110 mmol/L, headache, lethargy, confusion, agitation, and obtundation may be seen. More severe symptoms (seizures, coma) may occur with levels below 110 mmol/L.35 In that setting, hypoxemia is common and often is associated with noncardiogenic pulmonary edema.36 Focal neurologic findings are unusual but do occur, and brain herniation has been described in acute, severe cases, especially in young women following surgery.33 Hypoxia appears to exacerbate the cerebral damage in hyponatremia.37
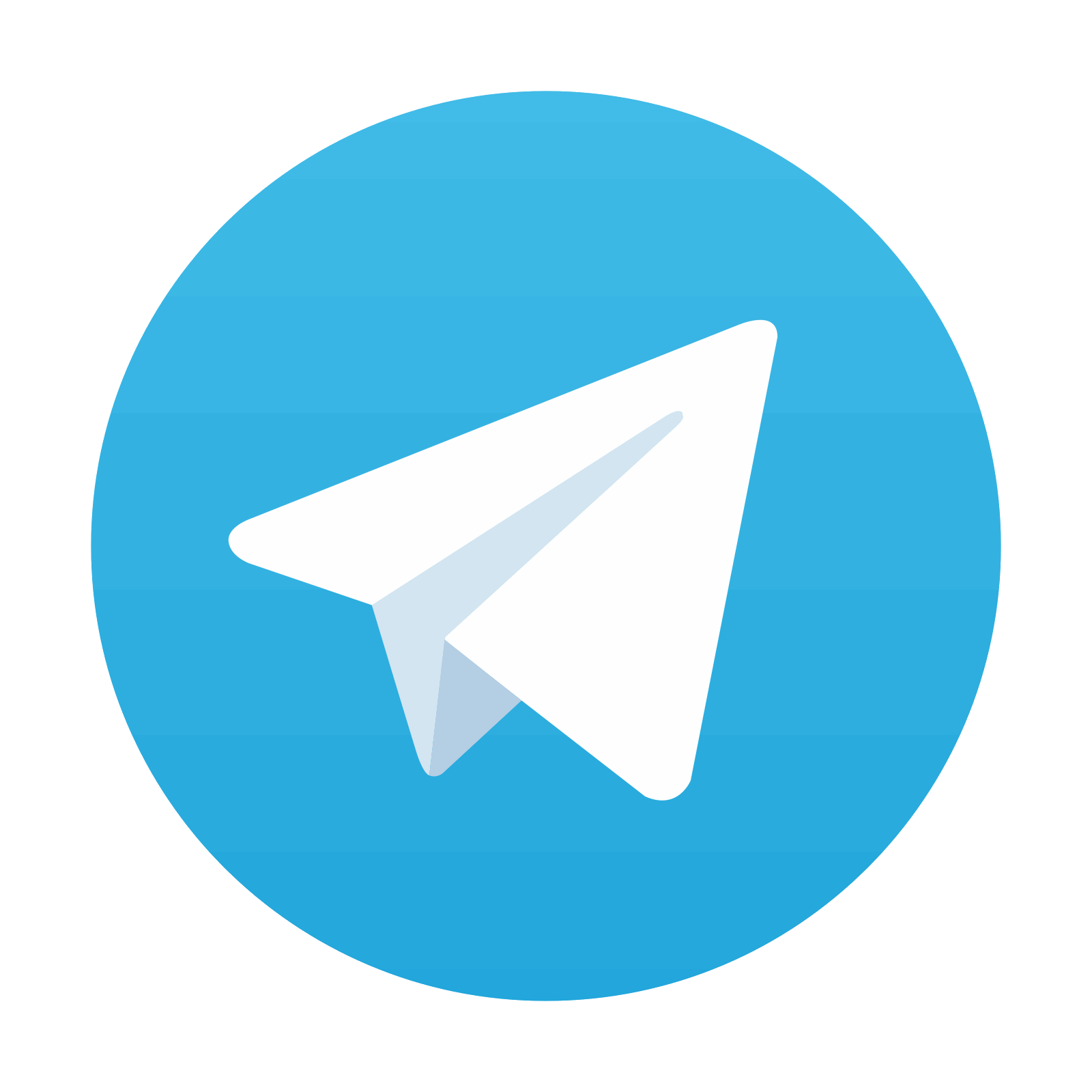
Stay updated, free articles. Join our Telegram channel

Full access? Get Clinical Tree
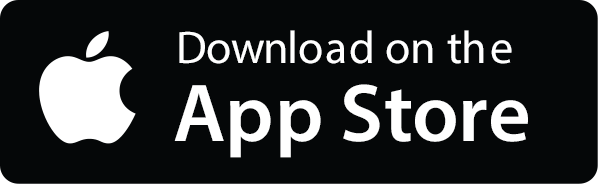
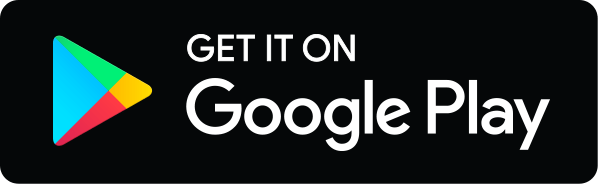