Recent advancements have made radiation and chemotherapy the standard of care for newly diagnosed glioblastomas. The use of these therapies has resulted in an increased diagnosis of pseudoprogression and radiation-induced necrosis. Standard MRI techniques are inadequate in differentiating tumor recurrence from posttreatment effects. Diagnosis of a posttreatment lesion as glioma recurrence rather than radiochemotherapy or immunotherapy treatment effect is critical. This increase in accuracy plays a role as newer immunotherapies incurring posttreatment effects on MRI emerge. Advancements with magnetic resonance spectroscopy, diffusion-weighted imaging, and functional positron emission tomography scans have shown promising capabilities. Further investigations are necessary to assess the imaging algorithms and accuracy of these modalities to differentiate true glioma recurrence from radiotherapy or immunotherapy treatment effect.
Despite advances in treatment for glioblastoma (GBM), the survival of patients with malignant gliomas has not significantly improved over the past 2 decades. Radiotherapy and chemotherapy (radiochemotherapy) is currently the standard of care for patients with GBM, followed by serial imaging to monitor posttreatment GBM progression. Increased posttreatment imaging has led to incidental diagnosis and awareness of progressive imaging lesions with or without clinical sequelae that do not clinically behave long-term as glioma recurrence. Imaging findings of treatment effect and GBM tumor recurrence are not easily distinguishable with conventional contrast-enhanced standard MRI and hence present challenges to investigators and clinicians. This article reviews the recent advances in radiology imaging modalities that have the potential to distinguish GBM recurrence from treatment effect after radiochemotherapy and immunotherapy.
Treatment-effect necrosis
Since randomized trials in the 1970s showed the clinical benefits of radiotherapy in malignant gliomas, a total of 60 Gy fractionated radiation to the target glioma and tumor margins has become standard care for these glioblastomas. Radiation-induced necrosis is the necrotic damage associated with radiation-based treatment modalities of malignant glioblastomas and is frequently identified in patients who undergo surgical treatment of progressive lesions within 6 months after radiochemotherapy. The clinical course for radionecrosis is highly variable and can present with focal deficits, increased intracranial pressure, or can be asymptomatic. Typically, radiation-induced necrosis occurs 3 to 12 months after radiochemotherapy, but occasionally does occur years after radiation treatment.
On MRI, treatment-effect necrosis commonly appears as a contrast-enhancing mass lesion on T1-weighted imaging with gadolinium, and on T2-weighted imaging with increased signal intensity in the necrotic central component of the mass lesion. This appearance on conventional MRI is indistinguishable from tumor progression or pseudoprogression using standard imaging techniques. Treatment-effect necrosis does appear to have an increased incidence in the periventricular white matter, which may be due to the vulnerability of frail blood supply to these areas.
The reported incidence of treatment-effect necrosis is approximately 3% to 24% after radiochemotherapy for glioblastomas with a direct association to increased radiation dose and larger irradiated brain tissue volume. Treatment-effect necrosis occurs most commonly at the site of maximal radiation exposure, typically in the surrounding area near the tumor or resection cavity. After 50 Gy radiation to two-thirds of the total brain volume or 60 Gy to one-third of the brain using fractionation, the approximate five-year risk for radiation induced necrosis is 5%. However, the real risk of treatment-effect necrosis may be underestimated because many of these patients die earlier than 5 years due to GBM tumor progression. Several clinical factors such as higher radiation doses, hyperfractionation, interstitial brachytherapy, reirradiation, and radiochemotherapy have been reported to increase the risk for treatment-effect necrosis.
Treatment-effect necrosis is a severe local tissue reaction to adjuvant radiochemotherapy, which manifests as a concomitant disruption of the blood brain barrier, necrosis, edema, and mass effect on MRI. Histopathologic characteristics in addition to the necrosis include gliosis, endothelial thickening, hyalinization, and fibroid deposition. Furthermore, these areas of necrosis are commonly scattered with tumor cells with uncertain viability on histologic review.
Pseudoprogression
Pseudoprogression is the early subacute treatment reaction with edema, contrast enhancement, mass effect, and sometimes with associated clinical symptoms, which suggests GBM progression—but these lesions and their associated clinical symptoms resolve and recover spontaneously without intervention. In early reports, pseudoprogression was identified and defined when, after radiochemotherapy, patients with malignant GBM were found to have new areas of contrast-enhancing lesions on MR imaging, mimicking glioma progression—but these lesions would subsequently improve without any further treatment. Because these lesions appeared as tumor progression, these early subacute effects of treatment were coined pseudoprogression. Pseudoprogression lesions differ from treatment-effect necrosis in that pseudoprogression typically is identified on early MRI done within the first 2 months after adjuvant treatment. Conversely, treatment-effect necrosis is a more late effect, typically occurring 3 months to a year after treatment.
The reported incidence of pseudoprogression in patients with malignant GBM treated with radiation alone is roughly 10%, with a significant increase in incidence when radiochemotherapy treatment is used. On follow-up imaging, these pseudoprogression lesions usually stabilize or shrink in size and contrast enhancement.
It has been suggested that pseudoprogression represents a spectrum of treatment-induced injury to neuronal tissue. With milder forms of injury, this mechanism may produce pseudoprogression on subacute MRI that will resolve without treatment, but more severe levels of treatment-effect injury may lead to true necrosis. Both pseudoprogression and treatment-effect necrosis have been noted to increase in frequency with the combination of chemotherapy with radiation.
Pseudoprogression
Pseudoprogression is the early subacute treatment reaction with edema, contrast enhancement, mass effect, and sometimes with associated clinical symptoms, which suggests GBM progression—but these lesions and their associated clinical symptoms resolve and recover spontaneously without intervention. In early reports, pseudoprogression was identified and defined when, after radiochemotherapy, patients with malignant GBM were found to have new areas of contrast-enhancing lesions on MR imaging, mimicking glioma progression—but these lesions would subsequently improve without any further treatment. Because these lesions appeared as tumor progression, these early subacute effects of treatment were coined pseudoprogression. Pseudoprogression lesions differ from treatment-effect necrosis in that pseudoprogression typically is identified on early MRI done within the first 2 months after adjuvant treatment. Conversely, treatment-effect necrosis is a more late effect, typically occurring 3 months to a year after treatment.
The reported incidence of pseudoprogression in patients with malignant GBM treated with radiation alone is roughly 10%, with a significant increase in incidence when radiochemotherapy treatment is used. On follow-up imaging, these pseudoprogression lesions usually stabilize or shrink in size and contrast enhancement.
It has been suggested that pseudoprogression represents a spectrum of treatment-induced injury to neuronal tissue. With milder forms of injury, this mechanism may produce pseudoprogression on subacute MRI that will resolve without treatment, but more severe levels of treatment-effect injury may lead to true necrosis. Both pseudoprogression and treatment-effect necrosis have been noted to increase in frequency with the combination of chemotherapy with radiation.
Identifying treatment effect on imaging after immunotherapy
With the development of novel clinical trials using immunotherapy as a potential treatment modality, evaluation of posttreatment enhancement on MRI is problematic. Contrast-enhancing pseudoprogression and treatment-effect necrosis is increased with multimodality radiochemotherapy, and these problems with poor diagnostic specificity of contrast enhancement will likely increase with addition of other novel modalities such as immunotherapy, anti-vascular therapy, and gene therapy. Addition of immunotherapy and other novel treatment modalities to the standard radiochemotherapy may synergistically enhance multiple apoptotic pathways through several metabolic pathways, which may explain the increased incidence of contrast-enhancing pseudoprogression and treatment-effect necrosis with multimodality therapy. Recent cases of dramatic early onset contrast-enhancing pseudoprogression in the first 2 months after immunotherapy and radiochemotherapy have been reported to present with dramatic size and contrast enhancement, followed with almost complete regression in 6 months—consistent with pseudoprogression.
Because pseudoprogression may represent a local inflammatory or immune response, immunotherapy posttreatment lesions might be more likely to present as pseudoprogression or treatment-effect necrosis. Immunotherapy specifically with immune stimulation may be ultimately found to increase the incidence of contrast-enhancing pseudoprogression lesions, which ultimately regress without altering treatment. Careful posttreatment imaging is then critical because these pseudoprogression and treatment-effect, contrast-enhancing lesions are more common with multimodality therapies and possibly further elicited by immunotherapy. Radiographic advances in imaging modalities are critical, if not even more important, for patients receiving immunotherapy to accurately distinguish glioma recurrence from treatment effect with greater sensitivity.
Radiographic advances in distinguishing tumor recurrence from treatment effect
Standard conventional MRI cannot reliably distinguish between the similar imaging characteristics of glioma tumor recurrence and treatment-effect necrosis or pseudoprogression. The determination of active tumor infiltration and recurrence is of utmost clinical importance, and making this radiographic distinction has important consequences. As novel therapies such as immunotherapy are investigated and developed, the incidence of treatment-effect necrosis and pseudoprogression may increase as more modalities are added to the standard multimodality of radiochemotherapy. Several recent advancements in radiographic modalities have shown promise in the noninvasive differentiation between glioma tumor recurrence and treatment effects, but these techniques have yet to be proven in large prospective trials.
Diffusion-weighted MRI (DWI) using the differences in apparent diffusion coefficients (ADC) is a nonstandard MR sequence that has shown promise in distinguishing treatment-effect necrosis from tumor recurrence after radiochemotherapy, but the specificity for DWI has yet to be fully characterized. This DWI is a physiology based technique that measures the diffusion of Brownian water movement in vivo. Increased cellularity decreases ADC values, which has been suggested as a method to distinguish glioma recurrence from treatment effects. A recent prospective study of 44 patient using DWI indicates that DWI-positive imaging in the immediate postoperative period is associated with imaging findings consistent with pseudoprogression or radiation necrosis in MRI scans 2 to 4 months later. A recent retrospective review of 18 patients with contrast enhancement indicated that lower ADC and ADC ratios were significantly more associated with glioma recurrence than treatment effect. This imaging differentiation suggests some role for DWI to help distinguish tumor recurrence from treatment effect, but the full clinical application of DWI requires further investigations.
Noninvasive, functional imaging using proton-based magnetic resonance spectroscopy (MRS) has also been used to distinguish necrosis from tumor residual or recurrence by characterizing the metabolic properties of the lesion on MRS imaging. Single voxel, early applications of MRS have had difficulty in heterogeneous lesions and differentiating between glioma and tumor mixed with necrosis. Recent multivoxel, three-dimensional proton MRS techniques have been applied to the brain and demonstrate the feasibility of MRS to distinguish between treatment effects and glioma. Using the most modern three-dimensional multivoxel application of MRS, a sensitivity of 94% and specificity of 100% was reported in an MRS evaluation of 28 glioma patients. Floeth and colleagues have demonstrated the feasibility and usefulness of MRS imaging to also distinguish between pseudoprogression and tumor recurrence after radiochemotherapy and immunotherapy. Although this functional proton-based MRS has shown promise, further investigations with larger samples are needed to confirm its reliability, feasibility with longer scan times, and usefulness in posterior fossa lesions, and sensitivity in distinguishing glioma recurrence from treatment effects. These advances in radiographic imaging are used to distinguish tumor recurrence from treatment effect in other novel treatment modalities, such as immunotherapy, and exhibit great promise for this clinical utility in all brain tumor treatments.
It has been suggested that a combination of two-dimensional MRS and DWI may increase the accuracy of distinguishing between tumor and treatment effect. In a study of 55 patients, the addition of ADC ratios permitted a more broad analysis, not possible with MRS alone, with a 96% rate of correctly differentiating between tumor recurrence and treatment-effect injury. Verma and colleagues have demonstrated this feasibility of combining several MR modalities, including DWI, to improve the tissue characterization of brain neoplasms.
Recently, biologic functional scanning with positron emission tomography (PET) has shown potential in identifying tumor recurrence. 18-Fluorodeoxyglucose PET can be used to distinguish tumor from necrosis—although with limited sensitivity and specificity because of the baseline high glucose use of the normal brain. Use of amino acid tracers and PET imaging improve on this inherent biology and have shown potential in discriminating between tumor and necrosis. This imaging modality shows as much promise or specificity as the MRS-imaging modality described above.
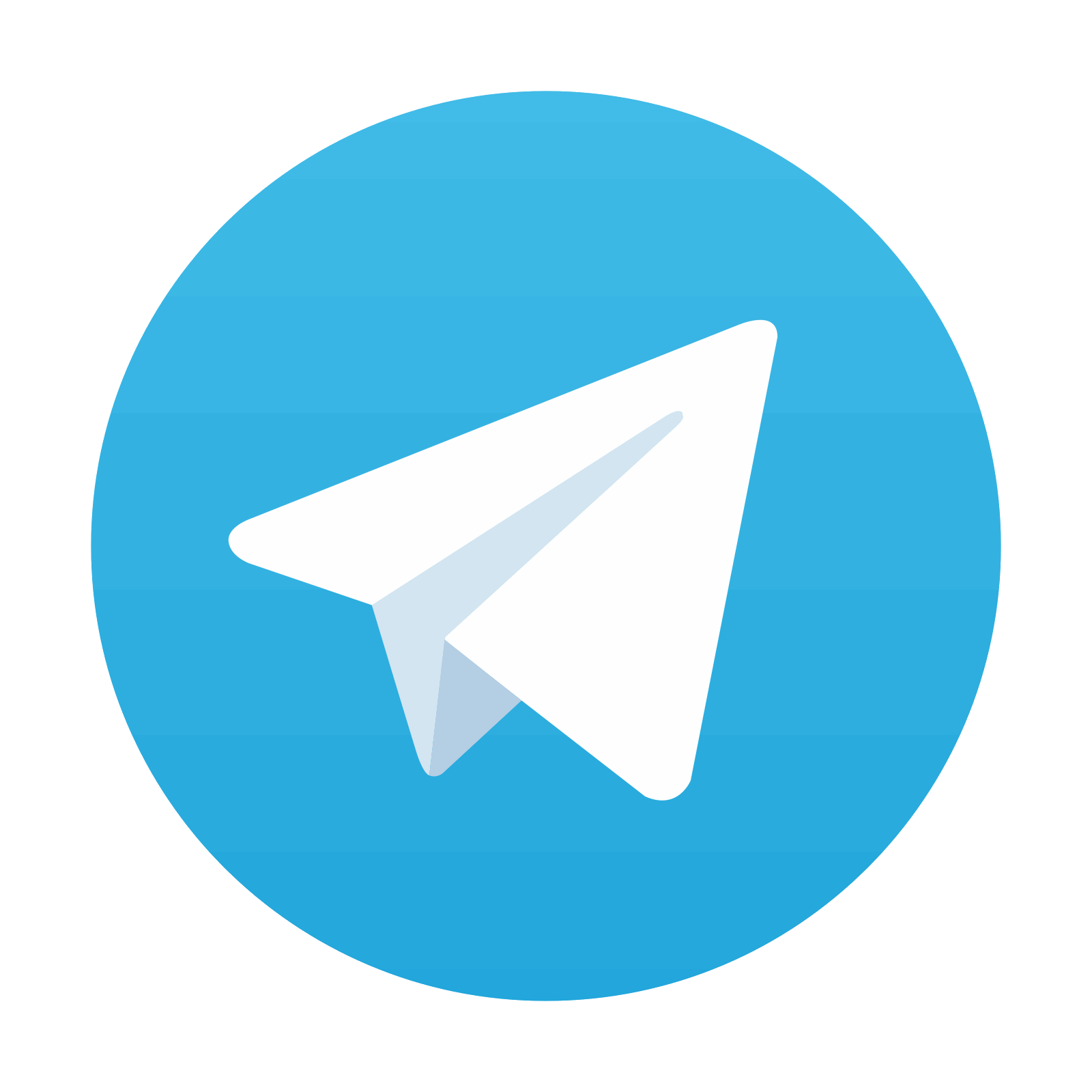
Stay updated, free articles. Join our Telegram channel

Full access? Get Clinical Tree
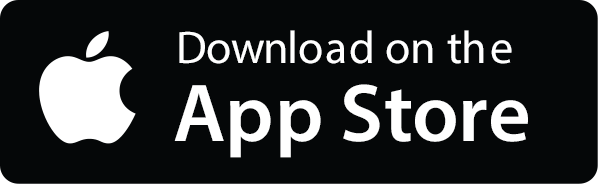
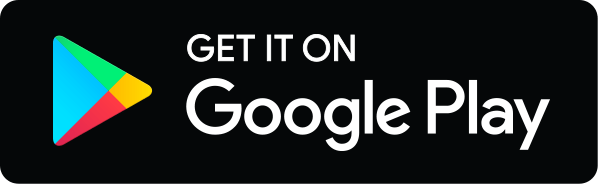