Summary of Key Points
- •
Anatomy of the pedicle varies between levels; knowledge of this anatomy is essential for proper pedicle screw placement.
- •
Other than intraoperative fluoroscopy, navigation systems and EMG monitoring may be used for proper screw placement.
- •
Polyaxial heads made the pedicle screw more versatile and became standard. Some of the hardware designs, such as side loading, translating screws, or rods with multiple diameters, allow for near endless possibilities for connecting polyaxial screws.
- •
In case of osteoporosis, screws may be augmented with vertebroplasty.
- •
Percutaneous pedicle screw fixation has found popularity, but it has the disadvantage of exposing the surgical team to more radiation.
The popularity of pedicle-screw fixation has significantly increased since the 1980s. Pedicle-screw fixation is the only spinal fixation strategy that engages all three columns of the spine. The pedicle screw–bone junction provides the strongest point of attachment of instrumentation to the spine. Thus, pedicle-screw fixation systems can resist motion in all planes. In addition, pedicle-screw systems do not require the presence of intact dorsal elements. A historic cohort study with the participation of 303 surgeons and 3498 patients has shown that the use of pedicle screws is a safe and effective form of treatment for spinal disorders.
History
Tourney in 1943 and King in 1948 provided the first descriptions of the use of bone screws to obtain internal fixation at the time of fusion. Their techniques for lumbosacral fusion involved passing a screw from medial to lateral across the facet of the involved level bilaterally. Their screws were short and designed to cross the facet joint only. Pseudarthrosis rates were unacceptably high with this method of fixation. For this reason, Boucher modified their technique by using a longer screw that crossed the facet joint into the pedicle and body of the vertebra below; these were the first pedicle screws. The pseudarthrosis rate for this technique, including multilevel fusions, was 14% to 17%. Magerl introduced another variation of facet screws in which a screw was passed from one side of the spinous process into the opposite lamina between its two tables, across the facet joint to the base of the transverse process. One disadvantage of the Magerl technique was that it required intact laminae.
Harrington initially used facet screws at multiple levels to correct scoliosis in patients with polio but found that this instrumentation eventually failed. This failure led to the development of Harrington instrumentation. Harrington first used screws inserted into the pedicles of L5 attached to Harrington distraction rods by heavy stainless steel wire for the reduction and stabilization of spondylolisthesis. Roy-Camille was the first to use pedicle screws connected to a dorsal plate. Beginning in 1963, Roy-Camille used pedicle-screw fixation in the thoracic and lumbar spine for fractures, for instability after the resection of vertebral tumors, and in lumbosacral fusions. A biomechanical study by Panjabi and colleagues demonstrated that facet-screw fixation stability was relatively low in flexion-extension and lateral bending compared with pedicle-screw fixation systems. Because pedicle fixation systems proved to be biomechanically superior for segmental fixation, numerous variations were developed. Universal spinal instrumentation (USI) systems were developed for the treatment of scoliosis through application of multiple corrective forces at different points on a rod. USI systems use both pedicle screws and hooks as anchors to the spine and connect them via rods. The first USI prototype was the Cotrel-Dubousset (CD) device (see Fig. 84-1 ), designed by Cotrel and Dubousset in France between 1978 and 1982. This was followed by the Texas Scottish Rite Hospital (TSRH) and the Isola systems. Perhaps the most important and unique feature of the TSRH system is the variable-angle screw that facilitated coupling to the rod regardless of the angle of screw insertion (see Fig. 84-2A ). Next, the CD system has been updated via the compact CCD and the CD Horizon spinal systems. The characteristics of the CD, TSRH, and Isola systems, and polyaxial screw systems as well as their advantages and disadvantages, are depicted in Tables 84-1 and 84-2 . Later generation/current generation posterior instrumentation (such as Styker Xia, Medtronic Horizon, or DePuy Expedium) have facilitated adaptability and have provided more options for performing posterior fusion by offering pedicle screws with polyaxial heads, variable diameter rods, and side-to-side connectors (see Fig. 84-3 ). They are made of composite alloys (typically, chromium cobalt or titanium), as opposed to stainless steel.


Cotrel-Dubousset | Texas Scottish Rite Hospital | Isola | Polyaxial New Systems | |
---|---|---|---|---|
Main advantages | Knurled rod | Rod contouring is minimized Fewer parts Removal is easier Titanium available Top and side loading available | V-groove attachment is easy and strong construct Top loading is easy Titanium available | More flexible screw head-rod connection Top loading and titanium Some screws are cannulated to introduce bone cement |
Main disadvantages | Extensive rod contouring is necessary Revision and removing is impossible without drilling or cutting implanted parts Multiple parts are confusing | Eye bolts should be preplanned and applied before connecting to screws and hooks Top-loading hooks are difficult to use | Adapters for connection can introduce points of movement | Multiple parts can cause confusion and may be associated with difficult assembly |

Pedicle-Screw Fixation
The pedicle is the sole bridge between the posterior column and the middle and the anterior columns. Hence, pedicle screws traverse all three columns and as such can rigidly stabilize both the ventral and dorsal aspects of the spine, as apposed to hook-rod systems, which affix only to the posterior spinal elements. For these reasons, pedicle-screw fixation systems, at least in the lumbar spine, are more commonly used than hook-rod systems. Commonly used implant systems are listed in Box 84-1 .
Cotrel-Dubousset (CD)
Texas Scottish Rite Hospital (TSRH)
Variable Screw Placement (VSP)
Isola
AO internal fixator
MOSS-MIAMI
Xia Spinal System
Synergy Variable Locking Screw (VLS)
Advantages of Pedicle-Screw Fixation
The nature of the pedicle screw–bone junction is much more secure than the wire or hook-bone junction. A single screw provides stability in five planes of motion. With transverse connection to the other side, stability is achieved in all six planes of motion. The rigidity of pedicle fixation allows for the incorporation of fewer normal motion segments to achieve stabilization of an abnormal level. Because the pedicle represents the strongest point of attachment to the spine, significant forces can be applied to the spine without failure of the bone-metal junction.
Pedicle-screw fixation systems are superior for restoring and maintaining spinal alignment. Because they traverse all three columns of the spine, they provide a longer lever arm through which the longitudinal member can transmit greater corrective forces than are achieved with spine fixation systems that attach to the posterior elements alone. Just as pedicle fixation systems resist loads in multiple planes, they are able to apply multidirectional corrective forces.
Another advantage of pedicle fixation systems is that they do not require intact dorsal elements. Thus, they can be used after laminectomy or traumatic disruption of the laminae, spinous processes, or facets. Pedicle fixation systems also avoid placement of instrumentation in the spinal canal, unlike sublaminar wires and hooks. Finally, fusion rates are thought to be improved with the pedicle-screw systems compared with earlier devices, as well as with noninstrumented fusions.
Disadvantages of Pedicle-Screw Fixation
A prolonged learning curve is required for the safe implantation of pedicle screws. Caudal or medial penetration of the pedicle cortex can result in durotomy or neural injury. Implantation of pedicle screws requires extensive tissue dissection to expose the entry points and to provide the required lateral to medial orientation for optimal screw trajectory. Lengthy operations with significant blood loss and an increased risk of infection are not uncommon. Significant osteoporosis is a relative contraindication to the use of pedicle screws because a solid screw purchase and subsequent fusion are difficult to achieve. Postoperative imaging techniques (especially magnetic resonance imaging [MRI]) are, in part, obscured by the implant. Rigid fixation can accelerate adjacent motion segment degeneration.
Pedicle Anatomy
The pedicle is a very strong, cylindrical, anatomic bridge between the dorsal spinal elements and the vertebral body. It is composed of a strong shell of cortical bone and a core of cancellous bone.
Pedicle size and angulation varies throughout the spinal column. The transverse pedicle width is less than the sagittal pedicle height except in the lower lumbar spine. Pedicle width is more important than pedicle height for pedicle-screw placement ( Fig. 84-4A ). The transverse pedicle width increases from L1 to S1. Most of the pedicles below T10 are greater than 7 mm in transverse diameter, and most below L1 are greater than 8 mm in diameter. In a study measuring the pedicle diameters with computed tomography (CT), Bernard and Seibert found that 20% of pedicles were less than 7 mm at L2, 15.6% at L3, and 1.9% at L4. No pedicles were less than 7 mm in diameter at L5 and S1. They concluded that all surgeons should perform preoperative CT scans when instrumenting pedicles above L4.

The transverse pedicle angle or coronal plane angulation ( Fig. 84-4B ) decreases as one descends caudally in the spine until the lumbar region. The angle increases as the lumbar spine is descended. The sagittal pedicle angle ( Fig. 84-4C ) is steep throughout the midthoracic spine and in the upper lumbar spine.
The intrathecal nerve roots course along the medial aspect of the pedicle. At T12, the dural sac is 0.2 to 0.3 mm away from the pedicle. Below L1, the medial side of the pedicle is almost touching the cauda equina. The nerve root occupies the ventral and rostral one third of the foramen. As a result, violation of the medial or caudal cortex of the pedicle risks injury to the nerve root.
Biomechanics
Rigid pedicle fixation techniques, such as rigid plate or screw-rod combinations, apply force to the spine by fixed moment arm cantilever beam fixation. A cantilever is a projecting beam that is supported at one end only. It resists axial loads by rigidly buttressing the spine. In the absence of load sharing with the anterior column, significant stress occurs at the screw-plate or screw-rod junction. These constructs tend to fail by screw fracture at this junction. However, modifications of the spinal implants (as discussed subsequently) have reduced the incidence of screw breakage.
Pedicle-screw fixation systems in which the screws are not rigidly affixed to the plate or rod allow toggling of the screws ( Fig. 84-5A ). These systems constitute nonfixed moment arm cantilever beam fixation. They are unable to resist axial loads without the assistance of an anterior column, which is capable of axial load bearing. Because these systems allow toggling of the screws, they may fail by screw pullout ( Fig. 84-5B ). For this reason, a fixed moment arm system more effectively resists sagittal translation.

Pedicle-screw fixation can be applied with either a flexion component or an extension component to the applied moment arm, which is usually a rigid pedicle fixator. These constructs are used to reduce deformities. Extension moment arm application is their most common mode of application in this regard.
Pedicle fixation techniques may fail during axial loading. This is in part due to a tendency toward the development of a parallelogram-like translational deformity. Toeing-in of the screws and the use of transverse connectors help prevent this mechanism of construct failure.
The most frequently encountered problems with pedicle-screw fixation are hardware failure or failure at the screw-bone junction. Screw pullout, breakage, and toggle are often the result of biomechanically inappropriate applications.
Screw Characteristics
Most pedicle screws have a cancellous thread pattern. In general, screw outside diameters range from 4.5 to 7 mm. Screw lengths are measured from the tip of the screw to the base of the screw head. Pedicle-screw lengths typically range from 30 to 55 mm, with 5-mm increments. There are two predominant types of pedicle screws: self-tapping and nontapping screws. With nontapping screws, a separate tap is used to cut threads into the pedicle.
Screw strength is proportional to the cube of the core (minor) diameter. The larger the minor diameter, the greater the resistance to screw bending or breakage. The outside (major) diameter is an important factor in screw pullout resistance. Other important components of pullout resistance are the thread depth, pitch, and shape. Thread pitch is the distance from one point on the screw thread to the corresponding point on the adjacent thread. Pullout resistance is directly proportional to the volume of bone between the threads that is determined by the thread depth and pitch. The angle or shape of the thread can affect the interthread volume and hence the pullout resistance. Some systems use screws with a conical inner diameter. This conical shape minimizes screw fracture by increasing the diameter of the screw where it is most likely to fail.
Polyaxial heads have made the pedicle screw more versatile. Therefore, the screws may be easily connected to the rod. However, polyaxial pedicle screws have lower strength than conventional fixed head (monoaxial) pedicle screws, and they are vulnerable to fatigue failure or fracture. Despite inherent weakness compared to monoaxial screws, polyaxial screws are much more frequently used due to the improved ease of rod placement at various head angles. A biomechanical study has demonstrated that the polyaxial head coupling to the screw is the first to fail and may be a protective feature of the pedicle screw, preventing pedicle-screw breakage.
Factors Affecting Screw Holding Power
The main factors influencing pullout resistance of a screw are metal properties of the screw, bone mineral density, the geometry of the screw, and the screw insertion technique.
Self-tapping screws are used to increase the holding power of the screw. In a biomechanical study, it was reported that while tapping of a pilot-hole does not increase the pullout resistance, undertapping can increase the pullout resistance of the screw, even to values higher than those of the self-tapping insertion.
Another point that may negatively influence the screw’s resistance to pullout is the technique of insertion, removal, and reinsertion of a screw into the same hole. A biomechanical study by Delfino evaluated the strengths of cylindrical (5-mm outer diameter) and dual-core screws (5.2-mm outer diameter). The researchers found that insertion and reinsertion of either cylindrical or dual-core pedicle screws have compromised insertion torque and pullout strength of the implants.
Preoperative Planning and Surgery
Usually, preoperative routine radiographs and a CT scan should be examined to determine bone quality, pedicle transverse diameter, and screw trajectory. Because intraoperative radiographs are usually necessary, preoperative planning should include preparation for anteroposterior (AP) and lateral radiographs or fluoroscopy. Lateral radiographs can usually be obtained without difficulty whether either a table or frame is used. Special radiolucent spinal frames are useful, particularly for AP radiographs.
The patient is placed prone on rolls, a frame, or a table with the abdomen hanging as freely as possible to indirectly decompress the epidural venous plexus. A midline incision is used and carried down to the level of the spinous processes. An adequate incision length facilitates proper trajectory during screw hole preparation and screw placement. All soft tissue is dissected off subperiosteally to minimize blood loss. Muscle dissection should allow for a lateral exposure of the transverse processes on both sides, leaving the intertransverse membrane intact at all levels of the planned instrumentation. Release of the retractor every 30 minutes reduces muscle necrosis and thereby decreases the risk of infection. The spinal level is identified either anatomically or radiographically, and a decompressive laminectomy is performed if appropriate.
After placement of the pedicle screws, the dorsolateral bone graft bed is prepared by decorticating the lateral aspect of the facet joints and the transverse processes with a combination of a high-speed drill with a cutting bur and sharp, cupped curets. The synovium can be removed from the facet joints that are included in the fusion and packed with cancellous bone. The importance of meticulous decortication to achieving bony fusion cannot be overstated.
Pedicle Screw Entry Sites and Trajectory
Every spine surgeon should have a thorough knowledge of pedicle anatomy, including the coronal and sagittal plane angulation of the pedicle (see Fig. 84-4 ). The sagittal pedicle angle increases in the thoracic spine from an average of 0 degrees at T1 to 10 degrees at T8 and then decreases to 0 degrees at T12. Usually the L4 sagittal pedicle angle is 0 degrees, and subsequent rostral and caudal levels are associated with progressively greater sagittal angles. The lordotic curvature of the lumbar spine produces a rostral angulation for upper lumbar screws. The L5 pedicle is 5 to 10 degrees caudally inclined. Coronal plane angulation decreases from the cervical spine to the thoracolumbar region and then increases as the lumbar spine is descended. The coronal plane angulation at T1 is 10 to 15 degrees and at T12 is 5 degrees. A 10-degree medial angulation is satisfactory at L1. A wider angle in the coronal plane is necessary to avoid lateral penetration of the pedicle in the lower lumbar spine. The coronal plane angle increases approximately 5 degrees per level from L1 to the sacrum.
In the thoracic spine, the transverse process commonly does not align directly with the pedicle in the axial plane. For this reason, the anatomic landmarks that are used for lumbar pedicle-screw insertion cannot be used in the thoracic spine. The transverse process is rostral to the pedicle in the upper thoracic spine and caudal to the pedicle in the lower thoracic spine. The crossover occurs at T6-7. Because of the variability of the relationship of the pedicle to the transverse process, fluoroscopic guidance or direct vision and palpation of the pedicle via a laminotomy is recommended for insertion of thoracic pedicles. At the T1 to T3 levels, Louis has suggested the use of 4.5-mm diameter screws that are 25 to 30 mm in length. At the T4 to T10 levels, screws are usually 4.5 mm in diameter and 30 to 35 mm in length.
The conventional entry site for pedicle-screw placement in the lumbar region is at the junction of the lateral facet and the transverse process ( Fig. 84-6 ). Although the midline of the transverse process corresponds to the location of the pedicle at L4, this relationship does vary at different lumbar levels. Above L4, the midline of the transverse process is rostral to the pedicle, and at L5, it is an average of 1.5 mm caudal to the pedicle.

Several nuances should be considered for accurate cannulation of the pedicle, as well as to enable proper screw trajectory. The muscle dissection is performed as lateral as possible to allow palpation of the transverse processes. The lateral aspect of the pedicle is palpated with a nerve hook over the transverse process. If a decompressive laminectomy is not being performed as part of the operative procedure, a small laminotomy may be performed to palpate the medial aspect of the pedicle and its rostral and caudal borders. Palpation of the pedicle helps guide accurate placement of the screw into the pedicle and increases the safety of the placement. It is strongly recommended for placement of pedicle screws at the level of the conus medullaris and above.
A relatively new insertion technique for pedicle screw trajectory has been described, the cortical bone trajectory (CBT). CBT was originally described by Santoni as an alternative for osteoporotic patients due to higher amount of interface between screw and cortical bone. This technique requires a more medial entry point and cephalad-lateral trajectory. The starting point is in the inferior pars. If no decompression is performed, the inferior spinous process needs to be removed to allow for the lateral trajectory of the screw. Figure 84-7 demonstrates the appropriate anatomic landmarks and angles. Aside from the different landmarks, the CBT will require shorter screws; otherwise, the techniques of drilling, probing, and tapping are the same as with traditional pedicle screw insertion. The medial entry point allows for less soft tissue dissection. Additionally, single level or multilevel fusions can be a combination of CBT trajectory and traditional pedicle screws, as long as the CBT screws are in the rostral level. Given the significantly different trajectories between CBT and traditional pedicle screws—it is possible to have one of each type in the same pedicle—this technique could be used as a salvage technique or to treat adjacent level disease without requiring exposure of all previous hardware. One of the concerns with this technique is possible weakness in preventing rotation, especially if no interbody instrumentation is performed.

Screw Insertion
The entry site should be decorticated by using a bur and a high-speed drill or a rongeur. A bur or awl is also used to penetrate the dorsal cortex of the pedicle. A pedicle probe is used to develop a path for the screw through the cancellous bone of the pedicle into the vertebral body. The advancement of the pedicle probe should be smooth and consistent. A sudden plunge suggests breaking out of the pedicle, typically laterally. An increase in resistance indicates abutment against the pedicle or vertebral body cortex. After cannulation of the pedicle and vertebral body with the pedicle probe, the pedicle sounding probe is placed into the pedicle, which is then palpated from within to make sure there is not a medial, lateral, rostral, or caudal disruption in the cortex of the pedicle. The sounding probe should also be used to determine that there is bone at the bottom of the pilot hole, verifying that penetration of the ventral cortex of the vertebral body has not occurred.
One possible technique, after the pedicles have been probed, is that different-length Steinmann pins or K-wires may be placed bilaterally into the pedicular hole at each level. Using different-length pins on each side helps distinguish right and left on the lateral plain radiograph in case the pedicle hole trajectory or depth needs to be corrected. A lateral and an AP radiograph or fluoroscopic image is used to verify the trajectory of the pins. This, however, does not guarantee accurate screw placement. An alternative technique would be, after probing of the pilot hole ensured no cortical breach on all sides and bottom, to then tap the holes, reprobe, and then place the pedicle screws without the use of Steinmann pins. Using this technique, intraoperative x-rays are obtained after the pedicle screws are placed. With slightly oblique AP views, a pin located in the middle of the pedicle has a characteristic target sign. Direct AP views demonstrate the lateral-to-medial orientation of the screws. Excessive medial orientation of the screws seen on an AP film raises the concern of medial penetration of the pedicle by the screw. Lateral imaging is useful to view the depth of penetration into the vertebral body and the sagittal angulation of the trajectory. Ventral screw penetration is usually between 50% and 80% of the AP diameter of the vertebral body. Ventral screw penetration greater than 80% of the vertebral body on a lateral plain radiograph raises the concern of ventral penetration of the vertebral body cortex because of the convex shape of the vertebral bodies. To avoid vascular and visceral injury, the ventral cortex of the vertebral body must not be penetrated, although this would enhance the overall strength of the construct.
After confirmation of correct placement of the K-wires or Steinmann pins, the pedicle screw path is tapped if non–self-tapping screws are used. Some tapping screws are hollow and are slid over K-wires into the pedicles. This helps guide the tap along the same trajectory that the pedicle was previously probed. The screw is then guided into the pedicle by its purchase of the threads tapped into the pedicle. However, tapping is less desirable in cancellous bone because tapping weakens the implant-bone junction. It is of questionable value in the insertion of pedicle screws because pedicle screws rarely obtain cortical purchase within the pedicle. To maximize the bone purchase of the permanent screws, a tap that is smaller in diameter than the screw is used. Tapping is usually not performed at a greater depth than the pedicle.
After tapping of the pedicles, the permanent screws with the largest diameter that will fit and not fracture the pedicle are placed. The length of the screw is determined by measuring the length of the Steinmann pin or K-wire from the pedicle entry site to a depth of 50% to 80% of the vertebral body. The screw length should also be estimated based on measurement from preoperative imaging, usually CT or MRI. Screws in the lumbar spine usually have a 4.5- to 7-mm diameter and a 35- to 50-mm length. Next, the transverse processes and lateral aspect of the facet joints are decorticated to increase the likelihood of subsequent bone fusion. The screws are connected to a longitudinal member, usually a rod. A bone graft is then placed on the previously prepared fusion bed; the bone graft can be autograft from bone harvested during decompression or allograft.
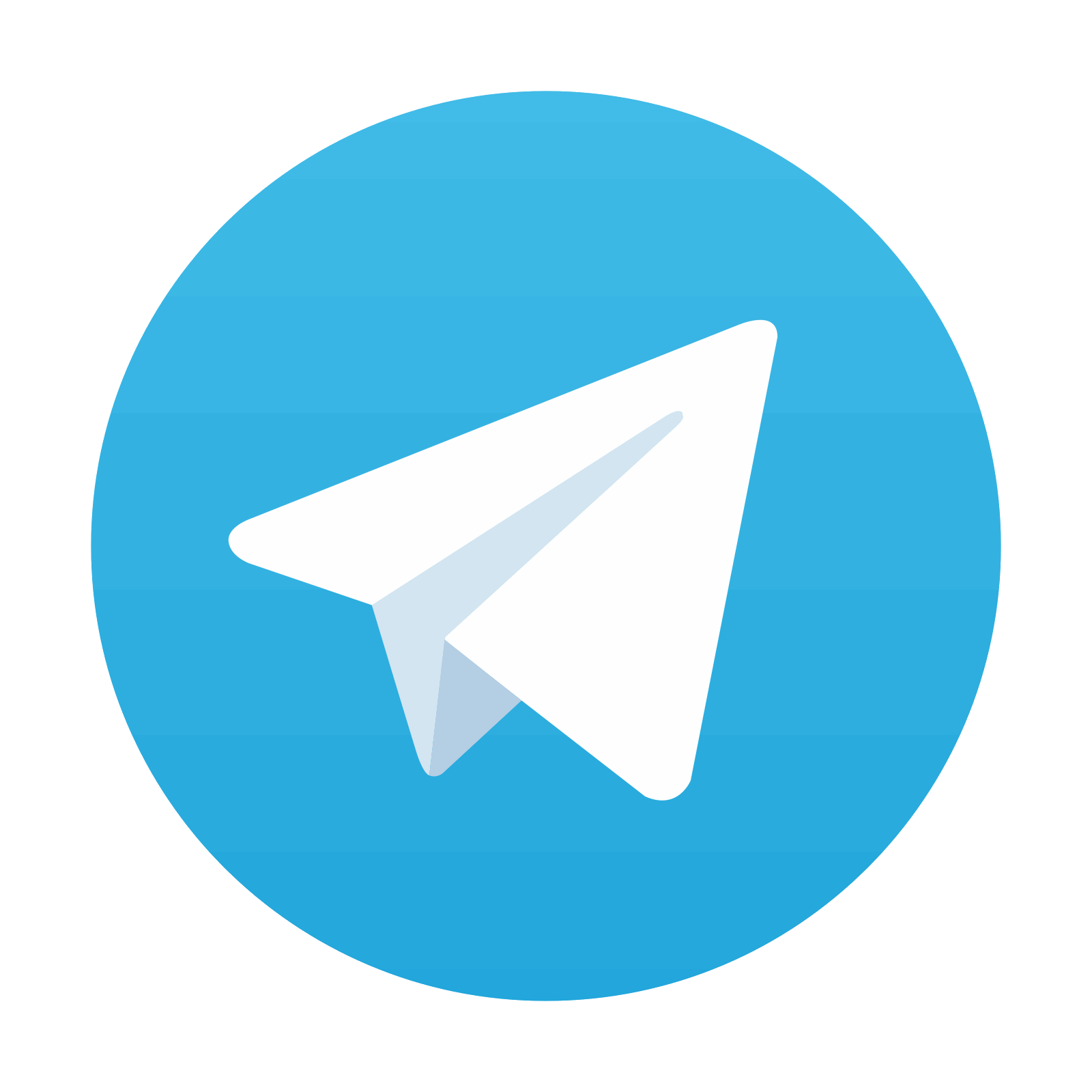
Stay updated, free articles. Join our Telegram channel

Full access? Get Clinical Tree
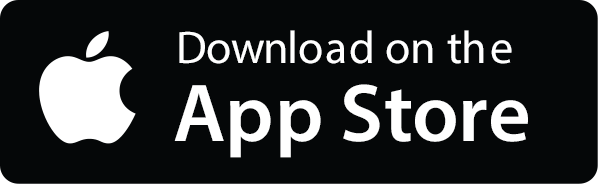
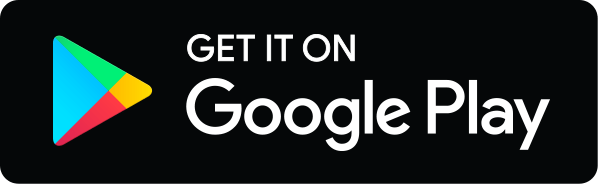