© Springer International Publishing Switzerland 2015
Raymond A. Huml (ed.)Muscular Dystrophy10.1007/978-3-319-17362-7_44. Duchenne and Becker Muscular Dystrophies: Underlying Genetic and Molecular Mechanisms
(1)
Department of Pediatrics and Clinical Neurosciences, University of Calgary, Alberta Children’s Hospital, 2888 Shaganappi Trail NW, Calgary, AB, Canada, T3B 6A8
Keywords
DystrophinDuchenne MDBecker MDCreatine kinaseAspartate aminotransferaseAlanine aminotransferaseCorticosteroidsRespiratory interventionDiagnosisPositive pressure ventilationGene therapyExon skippingNonsense mutationMyostatinTransforming growth factor-βCardiomyopathyCardiac arrhythmiaIntroduction
Duchenne and Becker muscular dystrophy are allelic disorders caused by mutations of the Duchenne muscular dystrophy (DMD) gene located on Xp21, which encodes for the dystrophin protein. DMD is the most common form of muscular dystrophy (MD) in childhood, with an estimated incidence of 1 per 3,500 live-born males [1] and a pooled prevalence of DMD of 4.78 (95% CI 1.94–11.81) per 100,000 males worldwide [2]. A brief review of the historical development serves to highlight the key clinical features associated with this disease. The condition was first described by Edward Meryon (1809–80), a British physician, in the 1850s [3]. His detailed clinical descriptions of eight affected boys including difficulty walking from an early age and later climbing stairs, with loss of ambulation and death occurring teenage years without any intervention are consistent with the progression and natural history of DMD. By microscopic exam, Meryon correctly surmised that the defect lies in the muscle cytoskeleton. A decade later, Duchenne described a young boy suffering from a progressive condition characterized by muscular weakness and calves hypertrophy; he was credited with the name for this disease [4]. In 1879, Gowers wrote a detailed review of the literature on DMD, including his own series of 20 cases. He provided clear illustrations of the method utilized by children with DMD to rise from the ground to a standing position; to this day, this phenomenon is still referred to as the Gowers’ sign [5].
Becker muscular dystrophy (BMD) is a generally milder and more variable form of dystrophinopathy, with an incidence of 1 in 18,518 male births [1], and a pooled prevalence of 1.53 (95% CI 0.26–8.94) per 100,000 males worldwide [2]. Similar to DMD, boys with BMD present with progressive muscle weakness and calf hypertrophy; however, the onset is often later and the progression is slower in BMD [6], with affected boys usually walking beyond the age of 16 years. Other presenting complaints of dystrophinopathies include myalgia and cramps [7], developmental delay, cognitive dysfunction [8], or dilated cardiomyopathy [9].
Case Example
A two-year-old boy was referred for gross motor and speech delay. He was born at 36 weeks with a birth weight of 3.2 kg; there were no postnatal complications. He rolled at 6 months, sat at 12 months, and walked at 19 months of age. His speech was delayed, with only five words at two years. Family history was unremarkable. On examination his growth parameters were normal. Cranial nerves II to XII were intact. Motor exam revealed symmetrical bulk and tone, with prominent calf muscles. Spontaneous antigravity strength was present in all four limbs, but he needed help to get up from the floor. Deep tendon reflexes were 2+ throughout, with down-going plantar responses. Sensation and gait were grossly intact. Investigations including brain MR imaging, TSH, hearing, and metabolic screen were normal. Serum creatine kinase (CK) levels were elevated, ranging from 7,975 to 17,988 U/L. Molecular genetic testing showed deletion of exons 46–53, an out-of-frame mutation in the dystrophin gene, consistent with a diagnosis of DMD.
In addition to motor developmental delay, proximal more than distal muscle weakness, and calf hypertrophy, this case illustrates that boys with DMD may have variable degrees of speech delay and/or cognitive impairment. Serum creatine kinase is usually markedly elevated, and muscle biopsy shows a dystrophic process with absent immunostaining for dystrophin. Progressive muscle degeneration is associated with scoliosis, cardiomyopathy, and respiratory insufficiency. With appropriate supportive strategies such as noninvasive positive pressure ventilation, survival beyond the third decade of life is now possible for many young adults with DMD [10, 11].
Genetics
The DMD gene is one of the largest known human genes. It contains 79 exons, including an actin-binding domain at the N-terminus, 24 spectrin-like repeat units, a cysteine-rich dystroglycan binding site, and a C-terminal domain [12, 13]. The extremely large size of the gene contributes to a high spontaneous mutation rate [14]; approximately one-third of cases occur as a result of de novo mutations [15]. Two-thirds of DMD mutations are due to large deletions, while duplications occur in about 10% of cases; the remaining 25% include small deletions, insertions, point mutations, splicing mutations, as well as other complex mutational spectra [16, 17]. Details on dystrophin mutations are available from the Leiden muscular dystrophy database (www.dmd.nl). According to the reading frame hypothesis, the phenotype for the majority (90%) of dystrophinopathies can be predicted by whether the genetic alteration results in an in-frame or out-of-frame mutation [18]. In-frame mutations produce a semifunctional truncated dystrophin protein resulting in BMD, whereas DMD is the result of out-of-frame mutations leading to a severely truncated and non-functional protein [19]. Three full-length isoforms are derived from independent promotors in brain, retina, and Purkinje cerebellar neurons. These and other tissue-specific isoforms are responsible for the extramuscular manifestations, including cognitive and learning issues [20].
Pathogenesis
Dystrophin is a 427 kDa cytoskeletal protein which is essential for muscle fiber stability. It binds to F-actin via its N-terminus and a cluster of basic repeats in its rod domain [20]. It also binds to dystroglycan via its cysteine-rich domain, and to dystrobrevin and syntrophin via its C-terminal domain; the latter is associated with nNOS, which is important in regulating the vasomotor response of the muscle, particularly during periods of exercise [21]. The dystrophin glycoprotein complex confers structural stability by forming a bridge across the sarcolemma and connecting the basal lamina of the extracellular matrix to the inner cytoskeleton; it is also essential for cell survival via its transmembrane signaling function [22]. Loss of dystrophin as a result of DMD gene mutations disrupts the dystrophin glycoprotein complex, leading to a cascade of events resulting in progressive muscle degeneration with diminished regenerative capacity, satellite cell depletion, and connective tissues replacement. In the absence of proper membrane-matrix attachment, mechanical stress from muscle contraction produces defects in the sarcolemma, with influx of calcium through the membranous lesions or through ion channels [21, 23]. Calcium dysregulation activates calcium-dependent proteases to further degrade muscle membrane proteins [24]. Furthermore, loss of nitric oxide results in increased oxidative stress, tissue ischemia, and reparative failure [25]. The progression is clearly visible with more severe fibrosis and fatty replacement, as well as more variation in fiber size in the later muscle biopsies [26].
Diagnosis
The diagnosis of Duchenne and Becker muscular dystrophy is based on careful review of the clinical features and confirmed by additional investigations including muscle biopsy and/or genetic testing [27, 28]. Suspicion of the diagnosis of DMD is usually triggered in one of three ways, including (1) most commonly, the observation of abnormal muscle function with signs of proximal muscle weakness in a male child; (2) the detection of elevated serum creatine kinase as part of routine screening; or (3) the presence of elevated liver enzymes including aspartate aminotransferase and alanine aminotransferase, both of which are produced by muscle as well as liver cells. The presence of gross motor or speech delay in a male child should trigger the order of serum creatine kinase as initial diagnostic screening for DMD, especially if the child also has an abnormal gait [29]. A positive family history is not required as up to one-third of cases may occur as a result of spontaneous mutation.
Detection methods for DMD mutations include multiplex PCR that examines the most commonly deleted regions of the gene, and other assays that interrogate all 79 exons, such as the multiplex ligation-dependent probe amplification (MLPA) or comparative genomic hybridization (CGH) microarray [17, 30]. If the presence of a disease-causing deletion or duplication is not identified by a state-of-the-art DNA diagnostic technique, complete gene sequencing is helpful to define the precise mutational event. A muscle biopsy can also be obtained for dystrophin immunostaining plus extraction of cDNA and RNA. Using all available diagnostic methods, it is possible to identify the dystrophin mutations and confirm the clinical phenotypes in nearly all patients with dystrophinopathy [31]. Identification of a specific dystrophin mutation is important for accurate diagnosis, prognosis, and treatment for patients with DMD/BMD as well as genetic counseling for their families. Most heterozygous female carriers of DMD mutations are asymptomatic. Approximately 2–8% of these carriers are manifesting carriers (MCs) who develop mild to moderately severe progressive DMD-like muscular dystrophy [32–34]. Rarely, they can also present exclusively with cognitive and/or cardiac dysfunction [35].
Treatment
There is presently no cure for DMD. Current strategies include promoting proper nutrition, delaying the onset of complications, and optimizing health outcomes through on-going support [27, 28]. Pharmaceutical interventions include the use of corticosteroids for skeletal muscle weakness and afterload reduction for cardiomyopathy. The introduction of noninvasive positive pressure ventilation has prolonged the survival of individuals with DMD. The mean age of death from DMD was 14.4 years in the 1960s, compared to 25.3 years since the advent of home ventilation in the 1990s, with improvement in patients’ health-related quality of life [11, 36].
Therapeutic Strategies
Recent scientific advances have led to potential disease modifying treatments for many neuromuscular diseases including DMD [37, 38]. Updated information about DMD clinical trials is available at http://www.clinicaltrials.gov. Treatments are also discussed in Chapters 7 and 12 of this book. Identification of coordination centers and patients eligible for specific DMD trials has been greatly facilitated by the establishment of TREAT-NMD and other international DMD disease registries [39]. The main therapeutic strategies include: (a) muscle membrane stabilization and upregulation of compensatory proteins [40, 41]; (b) enhancement of muscle regeneration and reduction of the inflammatory cascade [42, 43]; and (c) gene therapy to restore protein production [44, 45]. Examples of current therapeutic strategies are highlighted below.
Gene Therapy
(a)
Gene-replacement using virus vectors: Previous attempts to develop gene therapy for DMD have been complicated by the enormous size of the dystrophin gene. Subsequently, deletion of multiple regions of the dystrophin protein led to generation of highly functional mini- and micro-dystrophins; injection of adeno-associated viruses carrying micro-dystrophins into dystrophic muscles of canine model of DMD results in a striking improvement in the histopathological features of this disease [26, 46]. Clinical trials designed to replace defective genes in DMD are in progress [47].
(b)
Exon-skipping: Exon-skipping uses synthetic antisense oligonucleotide sequences to correct the dystrophin gene deletion by causing the muscle cells to “skip over” the reading of specific exons, and then produce an internally truncated protein similar to the dystrophin protein expression seen in BMD. An earlier phase 1 clinical trial using PRO051, an antisense oligonucleotide, showed partial restoration of dystrophin after a single intramuscular injection into the tibialis anterior muscles of four boys with DMD [48]. This was followed by other phase 2 studies including repeated injections of drisapersen, a 2′-O-methyl-phosphorothioate antisense oligonucleotide, into a larger number of DMD boys over a 48-week study period, with similar promising results [49]. Antisense therapies that induce single or multiple exon-skipping could potentially be helpful for other types of dystrophin mutations [50, 51].
(c)
Nonsense suppression therapy: Approximately 10–15% of dystrophin mutation is due to point mutation leading to a premature stop codon [52]. Premature stop codons are nucleotide triplets within mRNA that signal the termination of translation by binding release factors which cause the ribosomal subunits to disassociate, releasing the amino acid chain, and causing any resulting protein to be abnormally shortened. This often results in a loss of function in the protein, as critical parts of the amino acid chain are no longer created. Ataluren, also known as PTC124, is an orally bio-available drug candidate designed to overcome premature nonsense mutations [53]. It binds to the large ribosomal subunit, where it causes a conformational change and thus allows ribosomes to read through the premature stop codon in mRNA to produce a modified dystrophin protein. Phase 2 and 3 studies of ataluren show promise for the treatment of boys with premature stop codon mutations [54, 55].
Cellular Targets
(a)
Cytoskeleton protein upregulation: The dystrophin glycoprotein complex forms a bridge across the sarcolemma and flexibly connects the basal lamina of the extracellular matrix to the inner cytoskeleton. It also acts as a transmembrane signaling complex which is essential for cell survival. Compensatory upregulation of cytoskeleton proteins including utrophin [56], alpha-7-beta-1 integrin [57], biglycan [58], and sarcospan [59] have been shown to stabilize the sarcolemma in the absence of dystrophin in mdx mice. Further clinical trials are pending.
(b)
Nuclear factor-kappa B (NF-κB): In DMD mouse models and patients, the IκB kinase/NF-κB (IKK/NF-κB) signaling is persistently elevated in immune cells and regenerative muscle fibers [60]. As well, activators of NF-κB such as tumor necrosis factor-α (TNF-α) and interleukins (IL-1 and IL-6) are upregulated in DMD muscle. As pharmacological inhibition of NF-κB using the NEMO-binding domain (NBD) peptide resulted in improved pathology and muscle function in murine model of MD [61, 62], additional research is needed to identify the role of selective NF-κB modulators for use in DMD. Potential therapies include N-acetylcysteine [63], green tea extract [64], idebenone [65, 66], and melatonin [67, 68] for DMD.
Muscle Regeneration
(a)
Insulin-like growth factor (IGF-1): The therapeutic potential of upregulating a positive regulator of muscle development and regeneration using IGF-1 has been demonstrated in the dystrophic mouse models, especially when combined with mesenchymal stromal cells [69, 70]. Due to its regeneration-enhancing mechanism, this combinational approach may have general applicability for other MDs.
(b)
Myostatin inhibition: Myostatin is a negative regulator of muscle mass. Inhibition or blockade of endogenous myostatin offers a potential means to compensate for the severe muscle wasting that is common in many types of MDs including DMD. A phase I/II multicenter clinical trial using a myostatin blocking antibody (MYO-029) for adult subjects with Becker muscular dystrophies and other dystrophies demonstrated safety, but was not sufficiently powered for efficacy [71]. Clinical trials using follistatin and other myostatin inhibitors are on-going [72, 73].
(c)
Transforming growth factor-β (TGF-β): Elevated levels of TGF-β in MDs stimulate fibrosis and impair muscle regeneration by blocking the activation of satellite cells. A number of anti-fibrotic agents have been tested in murine models of MD, including losartan, an angiotensin II-type 1 receptor blocker that reduces the expression of TGF-β [74–76]. Other potential fibrosis inhibitors include halofuginone [77] and targeted microRNAs [78, 79]; further clinical trials are pending.
Standard of Care for DMD
Until there is a cure, current treatment strategies for DMD focus on promoting well-balanced diet, participating in regular physical activity as tolerated, delaying the onset of complications via pharmaceutical treatments, and optimizing health outcomes through appropriate medical and psychosocial support. Recent publications have provided comprehensive reviews on the diagnosis and multidisciplinary management of DMD, including the use of prednisone or deflazacort (a corticosteroid anti-inflammatory product) to preserve muscle strength [80, 81], optimizing growth and development, surveillance for spinal deformities [82], managing respiratory complications [83, 84], and treating cardiomyopathy [85, 86]. As well, bone health, nutrition, learning disability, behavior problems, access to wheelchairs, and other adaptive technology should be included as part of the comprehensive treatment plan. The purpose of the DMD standard of care recommendations is to provide a framework for recognizing the primary manifestations and for planning optimum treatment across different specialties with a coordinated multidisciplinary team [27, 28]. Multidisciplinary coordination of care including respiratory, cardiac, orthopedic, and rehabilitative interventions has led to improvements in function, quality of life, health, and longevity.
Current pharmaceutical interventions include the use of corticosteroids for skeletal muscle weakness. Corticosteroids such as prednisone and deflazacort are the only medications currently available to fight against the rate of progression of muscle weakness and the development of secondary complications as part of the natural history of DMD. Deflazacort is an oxazoline derivative of prednisone, with similar side effects except for weight gain. Prednisone and deflazacort are being compared in a head-to-head fashion in the current FOR-DMD clinical trial. Historically, corticosteroids offer benefit to DMD boys by improving muscle strength and function [87, 88], prolonging independent ambulation [89, 90], plus slowing the progression of scoliosis [91] and cardiomyopathy [92]. On the basis of this literature and clinical experience, the current standard of care guideline strongly urge the consideration of corticosteroid therapy in all DMD patients [27, 28]. Continued treatment after the patient becomes non-ambulatory has also been shown to be beneficial [93].
A high index of suspicion for steroid-related side-effects needs to be maintained at all times, including the development of short stature, obesity, cataracts, and skeletal fractures [90, 94]. In particular, boys with DMD are at risk of developing multiple vertebral fractures due to combination of long-term corticosteroid use, muscular weakness, and immobility [95]. Given the impact of vertebral fractures on quality of life, cyclical intravenous pamidronate treatment should be considered to help minimize back pain and rebuild bone mass; however, long-term efficacy of bisphosphonate therapy for DMD remains limited [96, 97]. Current recommendation reserves the use of bisphosphonates to children with reduced bone mass plus symptomatic vertebral collapse and/or recurrent fragility fractures in the extremity, particularly in the context of persistent or multiple risk factors [27, 28].
Cardiac complication is common in DMD; most often it manifests as a cardiomyopathy and/or cardiac arrhythmia [98]. Cardiomyopathy is one of the leading causes of mortality in DMD [36, 99]. Despite the high prevalence of cardiac disease, most affected individuals are asymptomatic [100]; cardiac symptoms were reported in approximately 50% of DMD patients under 18 years of age, likely due to their low physical capability. Baseline assessment of cardiac function including electrocardiography (ECG) and echocardiogram should be done at diagnosis or by the age of six years, with reassessment at least once every two years until the age of ten years, and with annual complete cardiac assessments afterwards [27, 28]. Common ECG abnormalities include sinus tachycardia; as the posterior wall of the left ventricle is often most affected in DMD, abnormally tall R waves in V1 and deep Q waves in the inferolateral leads, ST depression, prolonged QT interval, and increased QT dispersion can also be found [101]. Echocardiographic evidence of structural heart disease in DMD patients includes LV hypertrophy, regional wall motion abnormalities, dilation of the cardiac chambers, valvular abnormalities, and LV systolic dysfunction [102]. Furthermore, LV wall motion abnormalities progress in a set sequence in DMD patients, initially involving the posterior wall and the apex, followed by the interventricular septum and finally the anterior wall [103]. Boys on corticosteroids will need additional monitoring for hypertension, especially when adjustment in the dose of corticosteroids is made periodically to compensate for growth. Afterload reduction therapy such as angiotensin-converting enzyme inhibitor or beta-blocker for cardiomyopathy may be indicated as early preventive treatment [27, 28, 101].
Patients with DMD are also at increasing risk of respiratory complications over time due to progressive loss of respiratory muscle strength. The earliest signs of respiratory insufficiency often manifest in sleep and include ineffective cough, nocturnal hypoventilation, sleep disordered breathing, and eventually daytime respiratory failure. Death is due to respiratory failure in the majority of individuals with DMD [36, 99]. The treatment of choice for sleep apnea and nocturnal hypoventilation in DMD patients is positive pressure ventilation, which can be delivered using nasal mask noninvasively. The use of noninvasive positive pressure ventilation has led to prolonged survival of individuals with DMD [104]. Benefits of noninvasive positive pressure ventilation include improved sleep quality, decreased daytime sleepiness, improved daytime gas exchange, and a slower decline in the pulmonary function, leading to reduced hospitalization and improved health-related quality of life. Additional supportive strategies may include mechanical insufflation–exsufflation and lung volume recruitment exercises [105, 106]. Treatment with oxygen alone should be avoided without ventilatory support as individuals with respiratory insufficiency secondary to DMD may develop worsening of their hypoventilation and hypercarbia [27, 28].
Individuals with DMD develop increasing joint contractures and loss of muscle extensibility as a result of progressive weakness, immobility, muscular imbalance about a joint, and fibrotic replacement in muscle tissue. Affected boys and their families should be taught how to do active, active-assisted, and/or passive stretching daily or at least of 4–6 days per week, initially focusing on the ankles and then to other joints or muscle groups as tolerated [27, 28]. Night splints can be worn to help minimize heel cord contractures, especially when present during the early ambulatory phase of DMD. Serial casting may also be tried for short periods of time as long as it does not significantly affect mobility [27, 28]. Ankle-foot-orthotics are generally not indicated for use during daytime ambulation as they may limit compensatory movements needed for efficient ambulation, add extra weight that can compromise ambulation, and make it difficult to rise from the floor [27, 28].
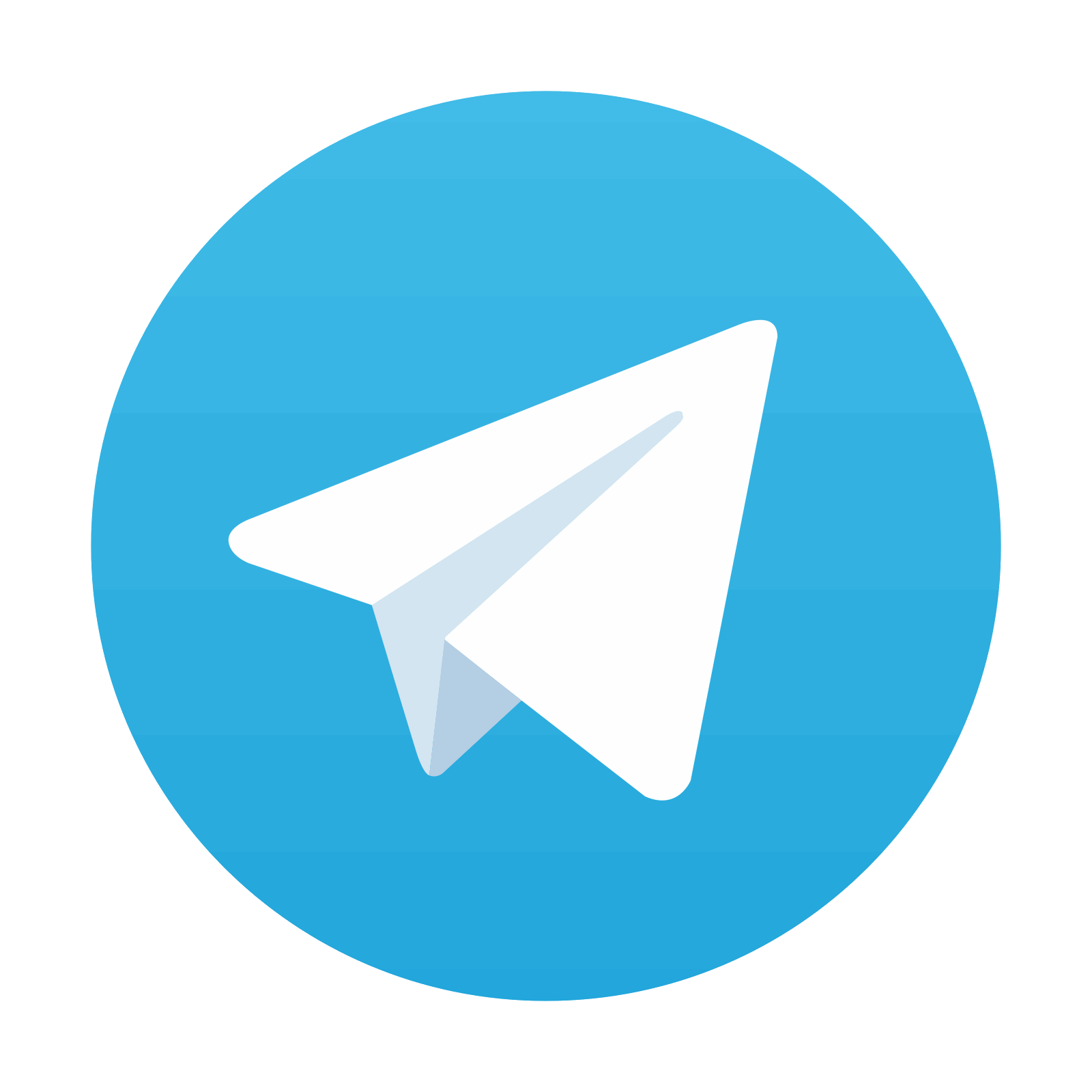
Stay updated, free articles. Join our Telegram channel

Full access? Get Clinical Tree
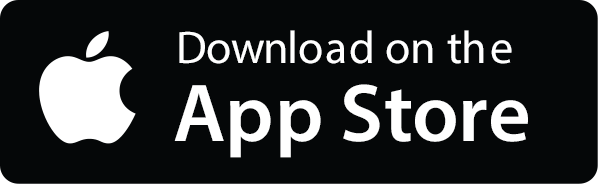
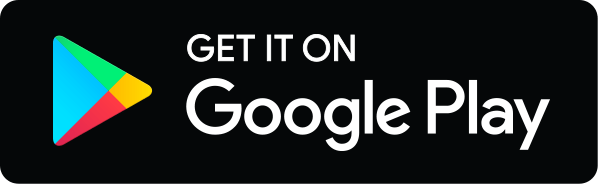