Historical context and biological basis
Early detection of solid tumors is vital for extending survival and possibly curing patients with cancer. This idea is especially relevant to patients with the most common and most lethal malignant brain tumor, glioblastoma (GBM), because earlier detection would likely increase not only survival but also quality of life. Historically, the more common malignancies, such as breast and colon cancer, paved the way for early discovery of these solid tumors, with mammogram and colonoscopy screenings saving countless lives. However, detecting solid tumors of the brain is a far more difficult task given that it is enclosed in the calvarium and imaging studies are not yet at the technical level to be considered fully diagnostic. In this regard, population-based screening with neurologic examinations and imaging is neither beneficial nor cost-effective. As with other cancers, screening for GBM would require a diagnostic test that has low false-positive rates in order to prevent unnecessary and costly imaging studies and further clinical work-up. Thus, clinicians and researchers have been pursuing biomarkers in the serum and cerebrospinal fluid (CSF) in order to detect GBM early enough for meaningful treatment.
Analyzing biofluids for markers of malignancy was initially pioneered in colorectal cancer with the detection of increased carcinoembryonic antigen (CEA) levels in the serum. However, levels of this normal physiologic protein were not always increased and were associated with numerous other cancer types, limiting its use as a diagnostic biomarker. Other biomarkers soon came to the forefront, such as prostate-specific antigen for prostate cancer and cancer antigen 125 (CA-125) for ovarian cancer; however, the specificities of these tests again failed to meet requirements for a diagnostic test. At present there are more than 20 US Food and Drug Administration–approved tumor biomarker proteins, most of which are used to monitor disease progression and response to therapy. However, GBM does not have a reliable serum biomarker, and initial detection currently relies solely on the presentation of symptoms related to the tumor size and/or location. For this reason, early detection is essential in order to initiate treatment before the patient is symptomatic, which is likely to affect quality of life and progression-free survival.
The ability to detect GBM early, ideally before clinical symptoms arise, is beneficial in 2 distinct ways in the current paradigm of therapeutic management. First, early discovery results in surgical resection of a smaller tumor volume, with likely less impact on surrounding structures compared with that of a larger tumor that is detected only after clinical symptoms are presented (eg, symptoms of speech difficulty, seizures, paralysis). Given that GBM invades into the surrounding tissue, the primary resection of a smaller tumor may necessitate less removal of normal brain parenchyma to prevent recurrence. Secondly, on a molecular genetic level, the total cell count of smaller tumors indicates less genetic variation between the tumor stem cells and their progeny, which can affect their ability to escape both chemotherapy and radiation treatment. Larger tumors have more genetic diversity in their cells, some of which aids in resistance to therapy, evasion of immunosurveillance, and initiation of recurrence.
Specific surface markers and mutations present in GBM may allow early detection in the serum and/or CSF. CSF is especially interesting given that the brain is bathed in this fluid, and there is a greater chance of finding relevant biomarkers because there is no blood-brain barrier to cross. However, collecting CSF is a more invasive process than collecting blood, hence discovery of a serum biomarker would be ideal. In addition, not all GBMs are alike and some respond better to different chemotherapeutic/radiation therapeutic regimens, so an ideal biomarker would be able to distinguish between the various molecular subtypes. Certain aberrations found in many GBMs, such as in the epithelial growth factor receptor (EGFR) and RNA profiling, as well as circulating tumor cells (CTCs) and extracellular vesicles (EVs), have the potential to diagnose GBM and also discriminate between classic, mesenchymal, and proneural subtypes. Thus, a reliable liquid biopsy from the serum or CSF would serve not only to improve GBM diagnosis but also would also affect treatment and surveillance for recurrence.
Development and evidence for biomarkers
Early detection of GBM has focused on the search for tumor-specific biomarkers in the blood and CSF. In this regard, current research concentrates on identifying 3 distinct tumor-related elements; extracellular macromolecules, EVs, and CTCs. Investigations into these 3 categories span the range of biological locales for tumor-specific biomarker discovery, and combined have the potential to affect GBM screening and diagnosis.
Detection of Extracellular Macromolecules
Detection of free nucleic acid species in the serum and CSF is of particular interest in GBM because tumor-specific aberrations in DNA, messenger RNA (mRNA), or microRNA (miRNA) may be used to distinguish malignancy from normal tissue. Furthermore, nucleic acid signatures may be able to speciate between the various subtypes of GBM, provide prognostic information, and direct tumor-specific therapies.
Recently, the presence of circulating tumor DNA (ctDNA) in the serum of patients with brain tumors was reported by several groups. As cells within the tumor begin to die and release intracellular content, ctDNA can be isolated from the serum and can give tumor-specific information about the molecular genetics of the malignancy. Specifically, methylation status of tumor suppressor genes and loss of heterozygosity (LOH) can be determined by analyzing ctDNA from patients with high-grade gliomas (HGGs). Serum ctDNA analysis can determine the methylation status of O 6 -methylguanine methyltransferase (MGMT) and phosphatase and tensin homolog (PTEN), as well as LOH in chromosomes 1p, 19q, and 10q, with a sensitivity of 50% to 55% and a specificity of 100%. Similarly, serum ctDNA can detect the presence of methylation in MGMT, RASSF1A, p15INK4B, and p14ARF in patients with malignant gliomas, with a sensitivity of 50% and a specificity of 100%. The poor sensitivity for these biomarkers in detecting intracranial tumors is likely related to the low concentration of tumor-specific ctDNA in serum, and suggests that CSF analysis could improve false-negative rates. Analysis of CSF has shown significantly higher concentrations of tumor-specific ctDNA compared with serum. Isolated circulating tumor DNA (ctDNA) has been shown to detect tissue-concordant mutations in the following genes: NF2, AKT1, BRAF, NRAS, KRAS and EGFR. Although the concentration of total ctDNA is less in CSF than in serum, the concentration of mutation-specific ctDNA and the mutant allelic frequency are higher in the CSF. Furthermore, mutations in EGFR, PTEN, ESR1, IDH1, ERBB2, and FGFR2 are readily detectable in CSF ctDNA, with a sensitivity of 58%, compared with 0% for serum. Together, the results of these studies indicate that analysis of free ctDNA in biofluids may be useful for early detection of mutation-specific intracranial brain tumors.
Because of the heterogeneity of tumors, not all malignant cells express the same genetic mutations, and investigating the RNA expression profile from biofluids may provide a more accurate real-time representation of the malignant process. Investigations into serum miRNA profiles show that patients with GBM have greater than 100 miRNAs that are significantly upregulated (highest: miR-340, miR-576-5p, and miR-626), as well as greater than 20 that are significantly downregulated (lowest: let-7g-5p, miR-7-5p, and miR-320), compared with normal healthy controls. Others have shown that the serum levels of miR-185 are significantly increased in patients with GBM, and that these levels returned to normal after surgery and subsequent chemotherapy and radiation. In addition, serum levels of miR-210 in patients with GBM correlate with both tumor grade and patient outcomes, compared with healthy controls. In contrast, serum levels of miR-205 are significantly decreased in patients with gliomas, and correlate with tumor grade, Karnofsky Performance Scale, and overall survival. Analysis of miRNA in the CSF of patients with GBM has also yielded some interesting results. CSF levels of miR-10b and miR-21 are significantly increased in patients with GBM, and miR-200 levels are only increased in patients with metastatic tumors of the central nervous system (CNS), enabling clinicians to distinguish metastases from primary malignancies with an accuracy of 91% to 99%. Similarly, levels of miR-223, miR-451, and miR-711 are also significantly increased in the CSF of patients with gliomas. The results of these studies indicate that the panels of miRNAs in the CSF may be used to not only diagnose GBM but also distinguish it from other CNS tumors in the future.
The search for protein biomarkers in GBM has focused on aberrations in levels of normal physiologic secreted proteins, given that no tumor-specific proteins have been identified. This approach relies on the detection of proteins secreted into the serum or CSF from cells within the malignancy, in hopes of identifying levels at which clinicians can diagnose lesions as GBMs.
By far the most studied serum protein in patients with GBM is glial fibrillary acidic protein (GFAP), an intermediate filament highly expressed in glial cells. Several reports have found that increased GFAP levels in the serum can accurately diagnose GBM. Overall, a GFAP level of greater than 0.05 ng/mL yields a sensitivity of 75% to 85% and a specificity of 70% to 100% for diagnosing patients with malignant gliomas. Furthermore, increased GFAP levels correlate with a lack of IDH1 mutation, and patients with non-GBM tumors did not have any detectable GFAP in their serum. Several other studies have focused on proteins other than GFAP. Increased serum levels of both YKL-40 and matrix metalloproteinase 9 correlated with active intracranial tumors and decreased overall survival. In addition, detecting increased serum levels of BMP2, HSP70, and CXCL10 results in a sensitivity of 96% and a specificity of 89% for diagnosing patients with HGGs. The analysis of serum EGFR levels appeals to GBM researchers given that most of these tumors have increased DNA amplifications and RNA expression of wild-type EGFR ( wt EGFR). Patients with GBM with increased serum EGFR levels have poorer outcomes and decreased overall survival. As with the other extracellular macromolecules, the search for protein biomarkers in the CSF may yield more GBM-specific proteins than in the serum. GFAP levels of greater than 0.04 μg/mL in the CSF correlate with the diagnosis of GBM, distinguishing this from other CNS malignancies and healthy controls. Similarly, myelin basic protein (MBP), a protein unique to the CNS, is of interest as a biomarker in the CSF of patients with GBM. MBP levels greater than 4.0 ng/mL in the CSF correlate with active tumors, and these levels decreased after surgery and chemotherapy. Other growth factors and cytokines have also been identified as possible CSF biomarkers of GBM. Most patients with malignant gliomas have increased levels of vascular endothelial growth factor (VEGF) and interleukin-6 (IL-6) in the CSF, distinguishing them from patients with low-grade gliomas and normal healthy controls. Thus, these proteins may serve as a basis for improving CSF biomarkers of GBM.
Recently, investigators have also started studying tumor metabolomics in serum and CSF. Increased serum levels of the cysteine metabolite correlate with GBM diagnosis, enabling distinction from oligodendrogliomas, which have increased levels of lysine and 2-oxoisocaproic acid metabolites. In addition, increased serum levels of myoinositol and hexadecenoic acid metabolites at the time of GBM diagnosis were of prognostic value in terms of long-term survival. In the CSF, metabolites from the amino acid, lipid, pyrimidine, and central carbon metabolism pathways are significantly different compared with those of healthy controls. Note that CSF levels of 2-hydroxyglutarate are increased in patients with GBM, indicating the presence of the IDH1 mutation in the tumor. Clinically, increased CSF levels of metabolites involved in tryptophan and histidine metabolism can be used to distinguish primary GBM from recurrent disease. Although metabolomic biomarkers are fairly new, they have the ability to study the inner workings of the malignant process, and have potential as a biomarker for GBM.
Detection of Extracellular Vesicles
Detection of EVs as a biomarker of GBM in both the serum and CSF is not as well investigated as that of extracellular macromolecules. However, given the harsh environment of the serum, and CSF to some extent, free circulating nucleic acids, and even proteins, are more prone to degradation than are EVs. The secretion of EVs is a normal physiologic process described in nearly every cell type, and is a broad term that includes microvesicles and exosomes. Microvesicles, typically 200 to 500 nm in diameter, are released via blebbing of the cell membrane and can contain elements in the cytoplasm at that time, such as mRNA and miRNA. Exosomes, typically 40 to 100 nm in diameter, are packaged and secreted via the endosomal system within the cell, and the mRNA and miRNA content is more regulated compared with microvesicles. However, both subgroups can contain elements of the cell membrane that are tumor specific and that, in addition to nucleic acid species, can be used as diagnostic biomarkers for GBM in the serum and CSF.
Given that EVs contain a measurable amount of nucleic acids, researchers have analyzed these profiles for GBM-specific biomarkers. The mRNA expression pattern of serum-derived EVs in patients with GBM contains significantly lower levels of genes associated with ribosome functions: RPL11, RPS12, TMSL3, and B2M. Similarly, mRNA expression of the EGFR variant III (EGFRvIII) mutation can be detected in serum-derived microvesicles, which can direct receptor-specific therapy in addition to surgery. Given that noncoding RNA can regulate mRNA expression, it is also prudent to investigate their utility as a GBM biomarker. Increased levels of RNU6-1, miR-320, and miR-574-3p in serum-derived EVs correlate with the diagnosis of GBM with a sensitivity of 87% and a specificity of 86%. Similar to free circulating macromolecules, GBM-specific EVs are more likely to be found in the CSF compared with the serum. CSF-derived EVs from patients with GBM express an average of 10-fold more miR-21 compared with those of nononcologic controls, and are able to diagnose this malignancy with a sensitivity of 87% and a specificity of 93%. Although microvesicles contain more total RNA, most of the miRNA in CSF-derived EVs is enriched in exosomes; greater than 40 miRNA compared with ∼10 miRNA in microvesicles. These intriguing results indicated that the miRNA profile of CSF-derived EVs may be used to diagnose GBM in the future.
Proteins within serum-derived EVs can also potentially be used as a biomarker for GBM. Serum-derived EVs of patients with GBM express significant levels of angiogenin, FGF-alpha, IL-6, VEGF, TIMP-1, and TIMP-2. In addition, using micro nuclear magnetic resonance (μNMR) technology on a quad panel of proteins (EGFR, EGFRvIII, PDPN, and IDH1) in serum-derived EVs has a sensitivity of 85% and a specificity of 80% for diagnosing GBM, and is able to predict response to temozolomide chemotherapy. More recently, researchers have also investigated CSF-derived EVs for the expression of GBM-specific genetic aberrations. Our group has recently found that the EGFRvIII mutation can be detected in CSF-derived EVs from patients with GBM, with a sensitivity of 60% and a specificity of 98% for diagnosing EGFRvIII-positive GBMs (Figueroa and colleagues, unpublished data, 2016). In addition, EGFRvIII-positive CSF-derived EVs have significantly increased expression of wt EGFR, which is known to be overexpressed in the classic subtype of GBM. These novel diagnostic tests have potential for not only diagnosing GBM but also determining EGFRvIII status and distinguishing between GBM subtypes.
Detection of Circulating Tumor Cells
The least studied strategy for the early diagnosis of GBM is the detection of CTCs. Typically, researchers investigate methods to isolate rogue malignant cells that have separated from the primary tumor mass and entered either the circulatory system or the CSF. However, the term CTC does not only apply to malignant cells but also includes subverted cells within the tumor stroma, such as endothelial cells and immune cells. At present, there are reports of serum CTCs identified in patients with GBM, but there are no studies investigating CTCs in the CSF.
The search for CTCs in GBM is difficult because there is no established tumor-defining cell surface marker. Instead, researchers have relied on various antibody cocktails that may target GBM-specific CTCs. A panel of antibodies, anti-CD14, anti-CD16, and anti-CD45, validated by analysis of known genetic aberrations in GBM, is able to detect CTCs in the serum that correlate with specific GBM subtypes. Similarly, using an adenoviral probe for human telomerase reverse transcriptase, which is known to be at increased levels in GBM, serum CTCs were isolated and validated by analysis for the overexpression of nestin, GFAP, and EGFR, as well as the absence of epithelial cell adhesion molecule, in patients with malignant gliomas. These promising results indicate that CTC detection is possible in GBM, and may be improved in the future to become a useful diagnostic protocol.
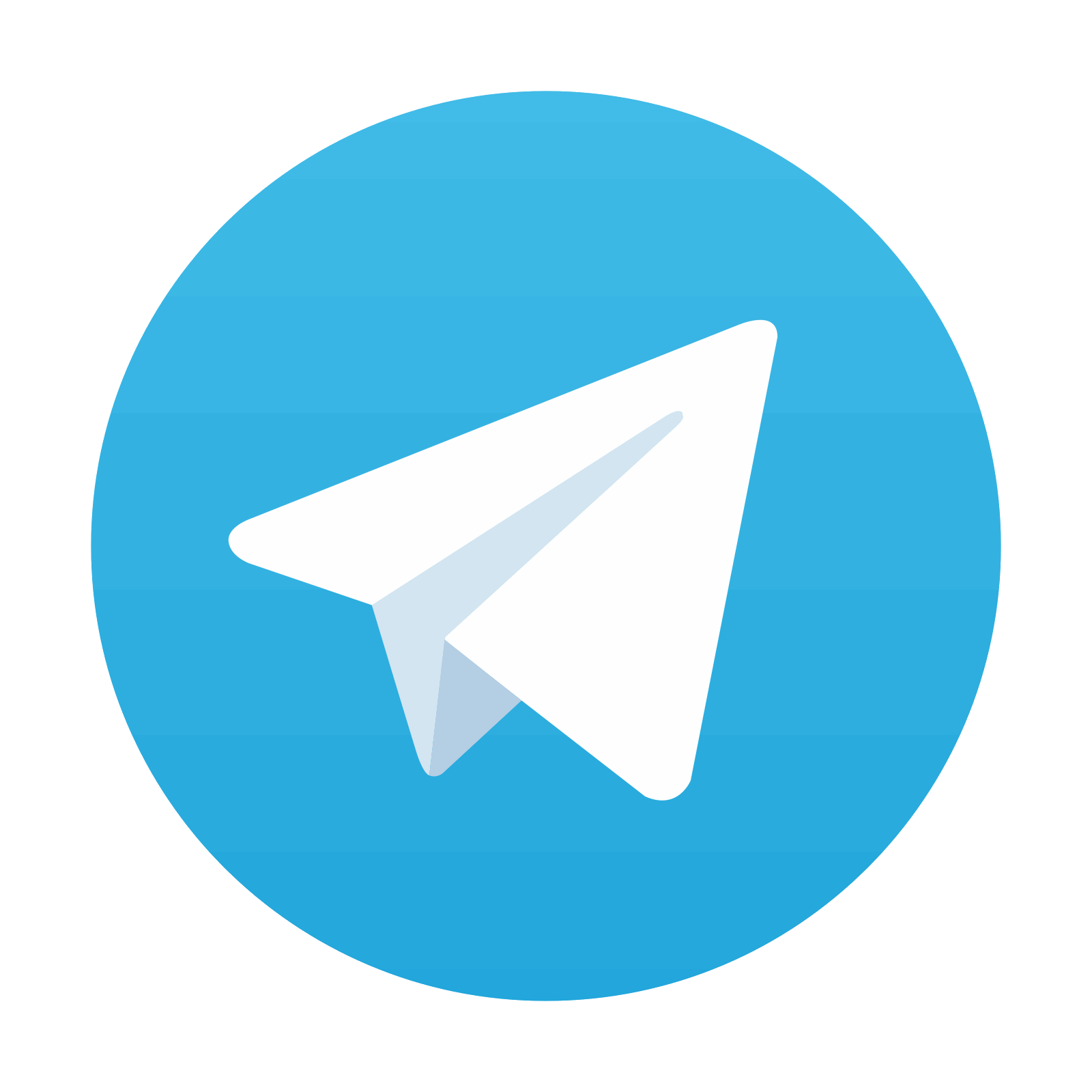
Stay updated, free articles. Join our Telegram channel

Full access? Get Clinical Tree
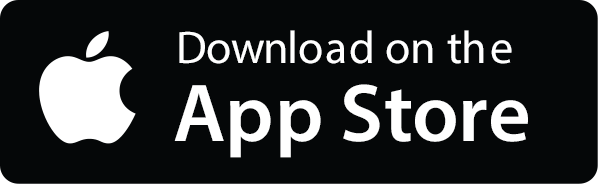
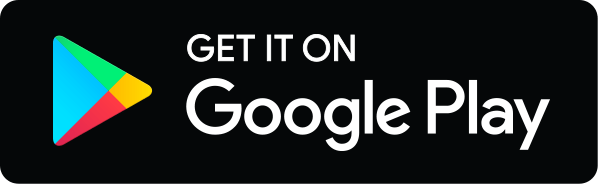