Broad environmental factors such as the constant availability of food, high energy density of common foods, and large portion sizes will also directly affect energy intake, and appetitive traits and environmental factors will interact with each other to affect consumption. For example, the expression of heightened food cue responsiveness will depend on the character and level of external food cues in the environment, and portion sizes could have a stronger effect on individuals with low satiety responsiveness. Additionally, appetitive traits and environmental factors may have a direct influence on each other. For example, long-term exposure to and consumption of large portions may decrease satiety responsiveness. Alternatively, an individual who is high in food cue responsiveness might seek out environments with a high availability of palatable, energy-dense food. Evidence for associations between appetitive traits and weight in both adults and children is reviewed below.
Evidence in Adults
Behavioral Studies of Satiety Responsiveness in Adults
Scientific testing of the notion that individuals might differ in appetitive behaviors, leading to differences in weight, began with a series of innovative experiments in the late 1960s. Consistent with the view at the time that obese participants were qualitatively different, rather than simply sitting at one end of an adiposity continuum, most of these studies used a “case-control” design in which performance of obese individuals was compared with that of a lean group. Albert Stunkard and Stanley Schachter were among the first to propose that obese individuals showed impaired sensitivity to internal satiety cues. In one seminal study, Stunkard inserted gastric balloons in 37 obese and 37 normal-weight individuals and recorded gastric motility and hunger ratings every 15 minutes for 4 hours. Motility was equivalent between groups, but when the stomach contracted, obese individuals’ hunger ratings were less likely to correspond to the change, suggesting differences in responsiveness to gastric cues to satiety (8).
In another study, taking intake rather than appetitive sensations as the outcome, Schachter introduced a design that went on to become a cornerstone of appetite research. Obese and lean participants were given two 15-minute “taste tests” on two separate days. For each test, they were provided with a “test meal” of a large quantity of plain crackers and invited to eat to satiety. On one day the test was immediately preceded by a meal of roast beef sandwiches (“preload”), while on the other they were given no “preload.” Results revealed that the lean group ate fewer crackers in the preload than the non-preload condition, but this did not occur for the obese group, that is, they failed to compensate for the difference in preload energy consumed. Schachter concluded that this absence of “caloric compensation” in the obese indicated a lack of satiety sensitivity (9). A more recent preloading study measured ad libitum energy intake following a high-fat meal compared to a similar, low-fat version and found that lean individuals compensated for 86% of the difference in preload calories, while obese individuals compensated for only 70% (10). In a similar study comparing ad libitum lunch intake following a small, low-fat breakfast (20% of average daily energy requirement) and a large high-fat breakfast (55% of average daily energy requirement), obese individuals also showed relatively less compensation (11). However, other studies have shown no weight-related differences in compensation (12, 13). (See Figure 9-2 for examples of caloric compensation responses.)
Figure 9-2 Preload and test meal intake in the caloric compensation paradigm: Examples of perfect compensation and no compensation
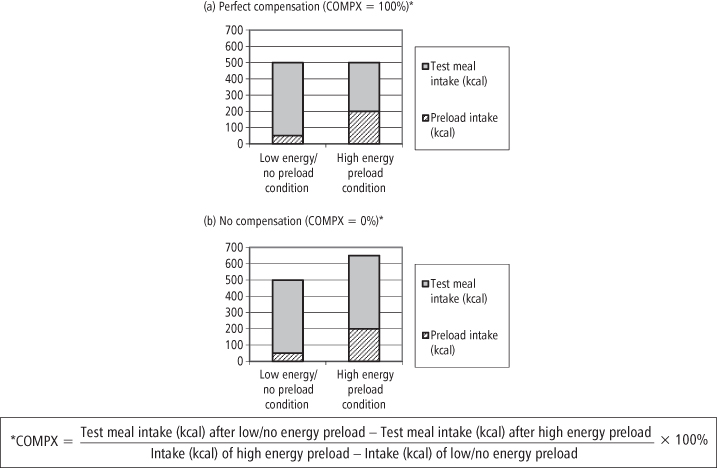
A separate line of research has examined patterns of eating rate. Faster eating may be thought of as reflecting greater responsiveness to food cues (i.e., the presence of the remaining food), but also as reflecting lower levels of satiety responsiveness, since we might think that responsivity to internal satiety cues triggered throughout the meal should result in a progressive slowing of eating rate as the meal progresses, and a slower overall average eating rate. Meyer and Pudel provided the first demonstration of obese/normal differences, finding that overweight adults were less likely to exhibit this pattern of deceleration; more typically, their eating rate increased throughout the meal (14). Differences were also uncovered in real-world settings—observation of eating in public places revealed that obese people ate faster than normal-weight individuals (15). More recent epidemiological studies using self-reported measures of eating rate have also demonstrated positive correlations with current BMI (16, 17) and BMI increases over time (16), even when adjusting for energy intake. However, other observational studies suggest that this finding may not hold across all settings. When intake patterns were recorded in adults over five laboratory meals, there was no evidence for an absence of deceleration, faster eating rate, or even greater intake in the obese group (18).
Behavioral Studies of Food Cue Responsiveness in Adults
Another line of work has focused on sensitivity to external cues to eat, such as the sight, smell, and taste of food. The construct of food cue responsiveness may be thought of as playing an important role in initiating eating, although any eating episode is likely to be influenced by both internal and external factors, and food cue responsiveness is likely to contribute to maintenance as well as initiation of eating. Corresponding to the fuzzy boundaries of the concept, studies in the area have come in several guises, tapping different elements of the proposed trait. One early experiment compared the change in obese and non-obese individuals’ intake when diet was changed from a general hospital diet to a bland liquid diet, with the striking finding that intake dropped drastically for the obese while remaining constant for the lean group (Hashim & Van Itallie, 1965, in (2)). This provided some initial evidence that obese subjects may be more responsive to the palatability of food than to internal cues to hunger, with the effect of actually lowering intake when the diet is bland and monotonous.
However, given that the modern diet is anything but limited, the effect of food cue responsiveness is more often to increase intake. This was neatly demonstrated in one study in which subjects were offered unlimited quantities of either a palatable or an unpalatable ice cream. Although there were no differences in intake of the unpalatable ice cream, overweight individuals ate significantly more of the palatable version than the lean group (2). Other clever studies demonstrated hyper-responsiveness to less immediate external cues such as the time of day (19). Schachter concluded in his influential paper in Science that “external or nonvisceral cues, such as smell, taste, the sight of other people eating, and the passage of time, affect eating behaviour to a greater extent in obese subjects than in normal subjects” (2).
Recent research on individual differences in food cue responsiveness has extended this work to look at biological markers. For example, Epstein and colleagues repeatedly presented tastes of a palatable lemon yoghurt to obese and lean women and monitored saliva production. Obese women demonstrated a slower decline in salivary response with subsequent presentations, implicating heightened cue responsiveness (20). The neurological correlates of cue responsiveness are also being explored, with recent functional neuroimaging studies revealing a pattern of greater activation in areas associated with reward, attention and motivation among obese compared with lean adults (21–24), and associations between questionnaire measures of cue responsiveness and altered connectivity within neurological reward circuits (25). Progress is also being made in the search for other biomarkers of obesity and appetite including peptide hormones such as leptin, ghrelin, PYY, CCK and GLP-1, although relationships are complex and it is far from clear whether dysregulation promotes or results from overweight (26).
Behavioral Studies of the Reinforcing Value of Food and Food Preferences in Adults
Two other aspects of appetite that may predict obesity are the reinforcing value of food for a person and his pattern of food preferences. The reinforcing value of food is a purely behavioral construct, which may be assessed by measuring the amount an individual will work to obtain a particular stimulus and is most commonly operationalized by presenting subjects with a task schedule specifying the amount of “work” they must do to obtain either a nominal food reward or a non-food reward. As the task schedule continues, the work required for the food reward becomes progressively larger, while the work for the non-food reward remains constant. Reinforcing value is indexed as the point at which the participant perceives the work for food to be too high a cost and crosses over to work for the non-food reward. One study using this technique found that, compared with lean females, obese females worked on a computer game task significantly longer for a food reward (a selection of palatable snack foods) than for an alternative reward (time spent doing sedentary leisure activities, e.g., viewing computer games, magazines, videos) (27). Similarly, overweight and obese (vs. lean) individuals work harder for high-calorie versus low-calorie foods, even when both foods are equally liked (28).
There is also some evidence that obese adults display a greater liking for foods that are high in energy density (e.g., high-fat) than do lean adults (29). This preference pattern is supported by epidemiological evidence for higher weight and risk of overweight among individuals with a pattern of eating high-energy density foods (30–32). However, clear differences in preferences have yet to be demonstrated (33), and the existence of a dissociation between liking (measured by preferences) and wanting (measured by amount of work done for food) (34–37), may explain the absence of studies reporting differences in preference using simple liking scales.
However, there may also be common roots to different types of eating behaviors. For example, one study found that obese women showed higher impulsivity in a behavioral test (38), suggesting obese eating behavior could be driven by a broad trait of impulsivity, while another found that overweight women scored higher on a psychometric test of sensitivity to reward (39). Others have highlighted the similarity between drug and food reward, suggesting that they may share origins in dopaminergic reward pathways (40). In support, PET studies of obese individuals have demonstrated a reduced level of striatal dopamine receptors (21), and other studies have reported a greater prevalence in obese people of the Taq1A1 allele on the DRD2 gene (41–42), which has been associated with decreased dopamine function across a range of subject populations.
Psychometric Studies of Appetitive Characteristics in Adults
Thus far we have focused on objective, behavioral rather than subjective, psychometric measures of eating behavior. However, the latter also have value in that they facilitate the rapid assessment of behavior patterns over a range of different situations among large groups of subjects and are less vulnerable to situation-specific influences. The Three Factor Eating Questionnaire (TFEQ; also known as the Eating Inventory, EI) is a well-known psychometric measure created by Stunkard and Messick (43). Reflecting the emergence of restraint theory as an explanation for obesity at the time of its development, the TFEQ contains scales measuring Cognitive Restraint (i.e., deliberate attempts to restrict intake in order to control body weight) and Disinhibition (i.e., a tendency to overeat in response to stress, failure to achieve dieting ideals, or external cues such as the presence of palatable foods). A third scale, Perceived Hunger, was designed to assess the general urge to eat. Between them, Disinhibition and Perceived Hunger capture a number of aspects related to lack of sensitivity to internal satiety cues, heightened food-cue responsiveness, and the rewarding value of food. A number of studies using the TFEQ have revealed differences by weight group, with obese individuals scoring higher on Perceived Hunger and Disinhibition (44–49), although other studies have failed to find the predicted associations (50, 51).
The Dutch Eating Questionnaire (DEBQ) (52) is another widely used eating behavior assessment tool and measures Restrained Eating (i.e., deliberate dietary restriction), Emotional Eating (i.e., eating in response to negative emotions), and External Eating (i.e., eating in response to external cues). Of these, External Eating is closest to the concept of food cue responsiveness, but has shown an inconsistent relationship to obesity, with some positive and some null associations (53, 54). (See Table 9-1 for example items for each scale.)
Table 9-1 Psychometric Measures of Eating Behavior: Example Items
Measures for adults (self-report) | |
Three Factor Eating Questionnaire (TFEQ, 35) | |
Cognitive Restraint | e.g., I deliberately take small helpings to control my weight |
Disinihibition | e.g., Sometimes when I start eating, I just can’t seem to stop |
Perceived Hunger | e.g., I’m always hungry enough to eat at any time |
Dutch Eating Questionnaire (DEBQ, 42) | |
Restrained Eating | e.g., Do you try to eat less at mealtimes than you would like to eat? |
Emotional Eating | e.g., Do you have a desire to eat when you are depressed? |
External Eating | e.g., If you see or smell something delicious, do you have a desire to eat it? |
Measures for children (parent report) | |
Child Eating Behavior Questionnaire (CEBQ, 70)* | |
Satiety responsiveness/Slowness in eating | e.g., My child gets full up easily/My child takes a long time to eat a meal |
Food Responsiveness | e.g., Given the choice, my child would eat most of the time |
Enjoyment of food | e.g., My child enjoys eating |
*Full CEBQ has four additional scales: Fussiness, Desire to drink, Emotional under-eating, Emotional overeating.
Although informative, the research described above does not enable us to draw conclusions about causation, because almost all of the data are cross-sectional, making it difficult to determine whether weight gain resulted from the eating traits or whether participants modified their eating styles as a result of their weight. The latter interpretation is particularly plausible for the case-control results (obese individuals may have been motivated to restrict their eating, leading to disinhibition and weight gain) but less so for the distribution-wide associations (normal- and low-weight individuals are less likely to have deliberately changed their eating habits). The causal ambiguity problem may be particularly apparent when interpreting results from the psychometric studies because many of the scales used were explicitly designed to assess the consequences of restraint—by definition an individual’s reaction to their weight. A similar issue may also explain interactions between different aspects of appetite. For example, Disinhibition may predict higher weight only when coupled with low actual levels of dietary restraint (44, 45, 48, 50, 55). Another problem is the possibility of biased reporting of eating behavior or anthropometric measurements where this information is obtained from the participants themselves. For example, if overweight adults minimize their endorsement of eating behaviors thought to be undesirable, and underestimate their weight and overestimate their height, this could decrease the chances of finding significant associations, producing the inconsistent results we have seen.
Evidence in Children
Behavioral Studies of Satiety Responsiveness in Children
Some of these problems can be overcome by conducting research in young children, preferably from community rather than clinical samples. Children are less likely than adults to alter their eating according to evaluations of their weight, and children from community populations may modify their behavior less than those from clinical samples, who show differences on a number of characteristics (56). Young children may also be less aware of societal norms and prejudices and hence less likely to strive for social desirability when reporting eating behaviors, although in practice parental reports are often used and these may also be biased. Measuring eating traits in children could prove useful clinically: if we can identify individuals who display “risky” behaviors in childhood, then we might be able to intervene early, preventing future weight gain and lowering obesity risk. This constellation of benefits makes research on appetitive traits in children particularly valuable.
As in adults, case-control studies have revealed a number of obese/lean differences in satiety responsiveness. For example, in a study using Schachter’s preloading paradigm, Jansen and colleagues (57) measured intake of a variety of palatable foods following either a preload condition, in which children were given a small amount of palatable snack food, or a control condition, where they received no preload. Lean children reduced their intake in the preload condition, but overweight children did not. Correlational studies have suggested that individuals vary along a continuum of responsiveness, with corresponding effects on weight. Johnson and Birch (58) assigned preschool children a compensation score (COMPX) based on their ability to compensate for a food preload by reducing intake later in the day. (See Figure 9-2 for examples and full definition of COMPX.) On this scale 100% represents perfect compensation and 0% no compensation, and in this study poorer compensation (lower COMPX score) was associated with greater sub-scapular skinfolds and BMI in girls. In a study of our own, conducted in a school setting and using two preloading tests in a sample of nearly 100 3–5-year-olds, we also demonstrated a trend toward a relationship between poorer compensation with higher BMI (59), although other studies have failed to find any association between compensation performance and weight in similar-aged or older children (60–62).
Interestingly, poor compensation may be associated with familial or genetic risk for obesity: one research group reported a decreasing degree of correspondence between energy density and food intake from 3 to 6 years of age in the diets of children with overweight mothers, suggesting that children at high risk for obesity may lose satiety responsiveness as they grow older (63). Another study reported poorer compensation ability in children who were inferred to be at higher risk for obesity based on BMI-associated polymorphisms in the PPARG (peroxisome proliferator-activated receptor) and ADRB3 genes (64).
Studies of eating rate in children report results as mixed as those reported for adults. For example, in the late 1970s, Drabman and colleagues (65) observed cafeteria meals and found that obese children ate at a faster rate, took more bites, and chewed each bite fewer times. More recently, another group compared lunch consumption among lean and obese 11-year-olds and found that the obese children ate faster and did not show any deceleration of eating rate towards the end of the meal (66). A lack of deceleration has also been demonstrated in obese 5–18-year-olds and those with Prader-Willi syndrome (67), although this study did not find a broader difference in average eating rate according to weight status. Another study found that the number of mouthfuls of food per minute in 4-year-olds predicted changes in BMI, skinfolds, and total fat from 4 to 6 years (68), and data from our own laboratory has revealed a graded positive association between eating rate and adiposity in children, which is evident throughout the BMI distribution (69).
Other research has suggested that associations between eating rate and weight may be evident even in infancy: one research group found that a vigorous sucking rate during feeding at 2 and 4 weeks of age predicted greater skinfolds and BMI at 1 and 2 years, and high pressure sucking predicted adiposity at 3 years (70); another study found that number of sucks per minute at 3 months predicted weight gain at 1 and 2 years, and was higher in the children born at high obesity risk, based on parental obesity (71, 72). The age of the children in these studies supports the idea that appetitive traits might be innate or genetically determined (see below).
Behavioral Studies of Food Cue Responsiveness and Reinforcing Value of Food in Children
Results from studies of children have shown that at least some indices of heightened food cue responsiveness are found in children as well as adults. The nearest thing to a test of this construct in the child feeding literature is the “Eating in the Absence of Hunger” test (EAH), designed by Birch and colleagues. In the usual paradigm, children are provided with a meal, asked to taste and rate 10 sweet and savory snack foods (e.g., potato chips, cookies, ice cream), then shown various toys and boxes containing generous amounts of the previously tasted snacks. Each child is then left alone with both toys and snacks for 10 minutes and intake is assessed. Given a range of palatable snack foods, most children are likely to eat a substantial amount when hungry, so, in order to avoid ceiling effects, only children describing themselves as “not hungry” after the meal are included in the analyses. The test therefore assesses the degree to which the child ignores their internal sensations in favor of responding to the external cue of the presentation of palatable food, and performance may be thought of as giving “an index of individual differences in responsiveness to the food cues in the environment” (73). Intake during this paradigm is higher among children who are overweight during middle childhood (74, 75), or who are at high risk of obesity on the basis of parental weight (76), and intake seems to be linearly associated with BMI across the distribution (77).
Other studies have attempted to assess biological indicators of food cue responsiveness. In a 2003 report, Jansen and colleagues (57) reported perceived hunger, salivary flow, and food intake in a taste test following either a control activity (playing) or 10 minutes of exposure to the sight and smell of palatable snack foods (large dishes of sweet and salty snacks). Hunger and salivation responses did not differ between obese and lean children, but the obese children exhibited a stronger behavioral reaction to exposure during the taste test, eating relatively more in this condition.
There has been less appetite-related neuroimaging research in children than adults, but in one recent study obese (vs. lean) children showed greater activation to food pictures in the prefrontal cortex (associated with executive function) and orbitofrontal cortex (involved in reward processing) both before and after a meal, together with lesser post-meal reductions in activation in the prefrontal cortex and other areas associated with emotion, and reward processing (nucleus accumbens, amygdala, putamen) (78). Obese (vs. lean) adolescent girls also showed greater activation in the gustatory cortex (insula, frontal operculum) and in somatosensory regions (parietal cortex/Rolandic operculum), but decreased activation in the caudate nucleus, in response to a milkshake compared with a tasteless solution (22). Children with Prader-Willi syndrome also show sustained activity in reward areas after a meal, further suggesting that obesity may be associated with abnormal responsivity in brain reward areas (79).
In terms of differences in the reinforcing value of food and in food preferences, Smith and Epstein (80) replicated in children the results from an experiment in adults (81), finding that obese participants initially chose to work for a highly liked high-calorie food, but when environmental constraints are substantially increased (via increased demands within a concurrent work schedule), they switch to a moderately liked low-energy food. Another study found that overweight children showed a slower decline in the amount of work done for food over a 20-minute period (82). A more recent study found that overweight (vs. lean) 8–12-year-olds worked harder for food than for non-food-related rewards (83), and in a one-year longitudinal study of 7–10-year-olds, higher relative reinforcing value of food at baseline predicted adiposity gain over the following year (84). Together, these studies suggest that a higher rewarding value of food may be associated with increased obesity risk.
Obesity-related differences in food preferences may be easier to discern for obese children than obese adults, as children are more likely to eat what they like and less likely to have developed patterns of eating influenced by dieting ideals or responses. Although we have not found evidence for differences in food preferences by child weight (33), we have observed higher preferences for high-fat foods and lower preferences for vegetables among children at high risk of obesity based on parental weight status (85).
As with adults, there is also some evidence that eating behavior may be related to behavior in other domains. For example, in one study a clinical sample of obese children displayed increased sensitivity to reward and reduced inhibitory control on questionnaire measures when compared with lean children (86). In another study, overweight children demonstrated less effective response inhibition than normal-weight children during a computer task, with correlations between task performance and greater ad libitum food intake (87).
Psychometric Studies of Appetitive Characteristics in Children
Most of the research in children has used behavioral tests to assess appetitive traits. These have proved essential to the process of identifying relevant eating styles and have the advantage of being objective measures of the traits in question. However, their disadvantage is that they are costly and time-consuming to run, keeping sample sizes small. They are also highly vulnerable to the variety of extraneous factors occurring on the day of testing. Both of these issues reduce statistical power to detect more modest relationships with weight. In contrast, psychometric tests, although lacking the objectivity of behavioral assessment, are designed to capture behavior patterns over a range of different situations. Children may not be able to report reliably on their behavioral tendencies, but a growing number of studies use parents’ reports of their children’s eating behavior, and parents have privileged observational access to their children, arguably making them the most accurate informants. Increasing evidence for associations between parent-report measures and child weight also speaks in their favor. For example, a parent-completed version of the DEBQ (DEBQ-P) was developed and showed significantly higher external cue responsiveness in a clinical sample of obese children (88), although this result was not replicated in a more recent community study (89).
An alternative parent-report instrument for assessing children’s eating behavior is the Child Eating Behavior Questionnaire (CEBQ) (90). The CEBQ includes scales assessing satiety responsiveness (i.e., sensitivity to internal satiety cues) and slowness in eating, food responsiveness (i.e., tendency to eat when palatable foods are available), and enjoyment of food. Each of these scales correlates well with behavioral tests designed to tap similar constructs (91) and shows continuity over time (92). Significant negative correlations between adiposity and Satiety Responsiveness/Slowness in Eating scores, and positive correlations between adiposity and Enjoyment of Food, have now been observed in a number of samples (93–95). (See Table 9-1 for example items for each scale.)
Finally, although these behavioral and psychometric studies are interesting, prospective research over the long term is required to see whether these traits play a causal role in the development of obesity. The question of whether there are interrelationships among the appetitive traits we have described must also be addressed. Although many of the suggested constructs are conceptually distinct, it is not clear whether they are functionally independent within individuals or in terms of their biological underpinnings. Multivariate analysis of behavioral and psychometric data, especially within genetically-sensitive studies, should shed some light on this area.
The Origins of Obesogenic Appetitive Characteristics
The causal model underlying appetitive differences is of substantial scientific and practical interest, and may incorporate both genetic (nature) and environmental (nurture) influences (see Figure 9-1). Evidence for possible genetic and environmental determinants of appetitive traits is described below.
Genetic Influences on Appetite and Weight
Heritability of Appetitive Characteristics
Family studies and twin designs have long been used by behavioral researchers to establish the heritability of weight-related traits. The essence of the method is to compare the magnitude of correlations between relatives on scores on a particular trait to the values that would be expected from their genetic similarity. If associations follow genetic relatedness, we infer genetic influence; if they do not, then environmental influences may be assumed. Twin data are particularly powerful, allowing the proportion of the variance in a trait that is attributable to additive genetic influences and environmental influences to be estimated. Shared environmental influences are common between twin pairs and make children growing up in the same family more similar; non-shared environmental influences are unique to each twin and make children growing up in the same family different (96). Around 55–85% of variation in adult BMI can be attributed to genetic influences (97–99), suggesting that the heritability of weight-related traits could also be high.
The small number of studies on the heritability of eating behavior traits have all used adult samples, and the majority have used data from the Three Factor Eating Questionnaire (TFEQ) (35), gathered as part of a larger cohort study. One such study of 28 Amish families estimated heritability at 28% for Cognitive Restraint, 23% for Perceived Hunger, and 40% for Disinhibition (100). Another analysis using 202 families from the Quebec Family Study produced much lower estimates of 6% for Cognitive Restraint, 28% for Perceived Hunger, and 18% for Disinhibition (101).
Twin studies have produced similarly mixed results. One study of 210 female twin pairs found heritabilities of 0% for Cognitive Restraint, 8% for Perceived Hunger, and 45% for Disinhibition (102). Shared environmental influences were significant only for Cognitive Restraint (31%) and Perceived Hunger (24%). In contrast, a study of 149 male and female twin pairs found significant heritability for Cognitive Restraint (44%) and Perceived Hunger (24%), but not for Disinhibition, which showed much greater shared environmental influence (40%) (103). A larger study of over 700 adult male twins reported much higher heritabilities using a modified version of the TFEQ—59% for Cognitive Restraint, 45% for Uncontrolled Eating, and 60% for Emotional Eating (104). It is unclear why these results vary so widely, but sample sizes may be too low to give robust estimates. Sex differences in TFEQ scores are also well documented (105–106) and could be another plausible explanation.
Another approach has been to examine the heritability of observed eating behavior, rather than scores on psychometric scales. De Castro and colleagues have conducted a number of studies using seven-day food intake records in adult twins, and have estimated that genetic influences account for around 42% of the variance in daily energy intake (adjusted for body weight), 28% of the variance in meal size, and 34% in meal frequency (adjusted for energy intake) (107). This group has also demonstrated significant heritability for a variety of other eating-related variables, which could contribute to several of the behavioral traits mentioned in the previous section, including energy density of the diet (108), subjective hunger (109), and pre-meal stomach contents (110).
With the exception of one recent report of 51% heritability of EAH intake in a cohort of 300 Hispanic families (74), research on the heritability of performance in eating behavior paradigms has focused on populations of adults, assessed at a time when families are likely to have lived apart since childhood. This substantially reduces the chance of detecting important shared environmental effects in childhood, which is reduced further in some cases by the small sample sizes, which reduce statistical power. However, obesity is highly heritable in children as well as adults (99, 111), suggesting that appetitive characteristics in children could also show genetic influence. Further, shared environment effects on weight appear to be greater when assessed in early life (112), suggesting an evaluation of genetic and environmental influence in children could give informative and substantively different results. Another problem is that data are most frequently gathered by self-report questionnaire measures or food diaries, which are vulnerable to inaccuracies and social desirability bias. The TFEQ scales represent rather complex behavioral traits containing attitudinal as well as behavioral components, which may make it more difficult to identify simple additive genetic effects. This problem is compounded in samples of adults, who may modify their eating behavior or reports according to socially prescribed attitudes.
In a study addressing some of these issues, we used preference data from a sample of 214 4–5-year-old twins to demonstrate modest heritability estimates for liking of dessert foods, vegetables, fruits, and protein foods (113). More recently, we used the parent-report CEBQ to assess the heritability of two fundamental eating traits—satiety responsiveness and food cue responsiveness—in a population-representative sample of 9–11-year-old twins. In a study of over 5,000 twin pairs, striking differences in correlations between MZ and DZ twins emerged on both Satiety Responsiveness/Slowness in Eating (MZ correlation = 0.62; DZ correlation = 0.09) and Enjoyment of Food scales (MZ = 0.80; DZ = 0.38), indicating substantial genetic influence. Model-fitting analyses indicated heritability estimates of 63% for genetic influence, 21% for shared environment influence, and 16% for non-shared environmental influence for satiety responsiveness; and 75% for genetic, 10% for shared, and 15% for non-shared for enjoyment of food (114). In another study we observed substantial heritability (62%) for eating rate assessed over a standard lunch meal in 10–12-year-olds (69). More recently, we have also shown that there is significant genetic influence at much younger ages: retrospective parental reports of milk-feeding behavior during the first three months of life revealed substantial heritability for slowness in eating (84%), satiety responsiveness (72%), food responsiveness (59%), and enjoyment of food (53%) (115). These studies suggest that eating behavior in children is highly influenced by genetic factors and that questionnaire measures may be a good way of measuring appetitive components of the obese phenotype.
Molecular Genetic Basis of Appetitive Characteristics
Having established that eating behaviors are heritable to some degree, the obvious next step is to ask which genes are responsible. A number of early studies attempted to do this, with some success. For example, Steinle et al. (100) performed a genome-wide linkage study for the three TFEQ scales in 624 participants from 28 Amish families, and found two chromosomal regions with linkage for Cognitive Restraint, two with linkage for Disinhibition, and another with linkage for Perceived Hunger. Another study reported data from the Quebec Family Study showing evidence for linkage on chromosome 15q24-q25—a region containing a gene encoding the peptide, neuromedin B—for both Disinhibition and Perceived Hunger (116). Other studies have found evidence for linkage regions for energy intake and macronutrient intake, some of which correspond to regions identified for other obesity-related phenotypes (116, 117).
Another approach has been to examine candidate genes with potential functional relevance for associations with eating behavior traits. For example, Aubert and colleagues (118) demonstrated that a polymorphism on the serotonin receptor gene was associated with energy intake in obese people, and this was replicated and extended to fat intake among children and adolescents from the Stanislas Family Study (119). In addition, polymorphisms of apolipoprotein A-II gene promoter have been associated with greater reported energy intake, specifically from fat and protein, suggesting it has a role in energy intake regulation as well as weight gain (120). More recent candidate-gene research has tested the relationship between reward-driven eating behavior and genes relating to dopamine function, and has revealed associations between the TaqIA1 allele of the dopamine D2 receptor (DRD2) gene and the reinforcing value of food (121), which could mediate the relationship between this polymorphism and obesity (122–124).
However, the most exciting recent advances in the genetics of appetite have come from genome-wide association studies (GWAS) assessing single nucleotide polymorphisms (SNPs). Using gene-chip technology, the A (vs. T) allele on the FTO gene (now known as the fat mass and obesity-associated gene), has been shown to be robustly associated with BMI and increased risk of becoming overweight and obese (125–127), as well as with weight gain in early infancy (128). Research on adults has since found that individuals with the risk allele consume more energy than those without the risk allele (129), although they do not expend more energy (129–130). FTO genotype may also influence macronutrient preference. One study found that 10–11-year-olds with the risk allele had higher intake of energy, specifically from fat, independent of BMI (131), while a study of adults found that self-reports of a high-fat, low-carbohydrate diet amplified the association between FTO genotype and obesity (132).
Other evidence suggests that FTO may affect appetite by influencing satiety sensitivity and food responsiveness traits: one study from our group reported reduced CEBQ satiety responsiveness scores in children with the AA genotype (133), while another found that the A allele was associated with greater eating in the absence of hunger, independent of BMI (134). Adult men and women with the A allele have also been shown to have significantly lower postprandial decreases in hunger and increases in satiety (135), while children and adolescents with the A allele have been shown to report more frequent episodes in which a loss of control of eating was experienced, as well as a preference for high-fat foods during a buffet meal (136). Together these studies suggest that alterations in appetitive behavior are at least one route by which FTO can affect weight.
Environmental Influences on Appetite and Weight: Parental Feeding Styles
Despite growing evidence that eating behavior is at least partly genetic, parental feeding styles have attracted by far the most attention as potential determinants of eating styles. Most studies have measured the feeding styles of parents and tested cross-sectional associations with eating styles among children; a small number have used retrospective measures of parental feeding style and related them to eating behavior and intake in adolescence and adulthood; while others have used prospective, longitudinal designs examining the prediction of eating behavior over time. Studies of the relationship between parental feeding and child weight have been reviewed elsewhere (137, 138); the focus of this chapter is on studies examining parental feeding and three aspects of eating behavior, which we have suggested may be relevant to weight: satiety responsiveness, food cue responsiveness, and food preferences.
Parental Feeding and Satiety Responsiveness
Earlier in the chapter we introduced the preloading design as a way to assess satiety responsiveness, in which poorer regulation is taken to indicate low responsiveness. In one of the first studies on parental feeding and eating behavior, Birch and colleagues (58) used this paradigm to test the idea that high levels of parental control over feeding might impair children’s satiety sensitivity by directing their focus towards external cues to satiety (e.g., the amount of food left on the plate, the amount of food that is permitted), instead of internal, physiological cues such as gastric distension and other post-ingestive satiety signals generated in the stomach and gut. Seventy-seven 3–5-year-old children participated in the test. Preloads were fruit drinks, with additional calories for the high-energy version provided by maltodextrin, a soluble glucose polymer, which makes negligible differences to taste. Lunches consisted of hotdogs, cheese slices, apple sauce, carrot sticks, Fig Newtons, and milk. Parental control was measured using a six-item Parental Control Index, derived by selecting from a wider set of parental feeding questionnaire items those items that showed high correlations with compensation. Significant negative correlations between control and compensation were found for both boys and girls (correlation (r) = −0.65, p < 0.001), with poorer compensation in children whose parents reported greater control over feeding. A subsequent study from the same group replicated this finding using a subscale specifically measuring parental restriction in a cohort of nearly 200 girls followed from the age of three years into early adolescence (75).
A few studies have also looked at how parental feeding relates to eating speed, with the hypothesis that parents of overweight children may be encouraging them to eat faster, and possibly greater amounts, via the application of particular feeding behaviors. For example, Drucker and colleagues (139) video-recorded 77 3–5-year-olds eating a laboratory-based buffet lunch accompanied by their mothers and found that average eating rate was positively associated with maternal encouragements to eat, although the expected link between faster eating and higher child weight was not apparent. Another study of older children did not measure parental feeding directly, but found that overweight children ate faster, took larger bites, and ate faster toward the end of a meal, but only when their mother was present (140). This evidence suggests parental efforts to promote eating may quicken children’s eating rate in the presence of the parent, but it should be noted that pressure to eat may not always be successful in securing greater intake: another group reported that an 11-week researcher-led intervention to pressure children to finish their food was associated with less intake and more negative attitudes toward the food (141). Others have examined parental restriction of high energy-density foods and found that a maternal restriction scale was positively correlated with daily energy intake in 5-year-old girls as assessed from mothers’ three-day, 24-hour recalls, consistent with the hypothesis that higher restriction may be associated with lower sensitivity to satiety, (75, 59).
Most parental feeding research thus far has looked at the eating behavior of children 3 years old and older, but a growing number of studies are examining the possible impact of very early feeding practices on eating behavior in infancy and beyond. For example, an association between duration of breastfeeding and lower infant weight has been observed in some studies (142), and it has been suggested that breastfeeding might teach the mother to be responsive to the infant, making her less likely to exert control over feeding and more likely to allow her infant to learn to feed according to his/her own satiety cues (143). Others have suggested that feeding “on demand” may engender a pattern of frequent snacking later in life, although it is hard to tell whether such a pattern would constitute evidence for an enduring impact of feeding style on the child’s appetite, or merely the child demonstrating eating habits learned from the parent (144). There may also be interactions between parental feeding style and child weight trajectories. One study found that if maternal control was low to moderate, then higher weight gain in the first six months was related to lower weight gain in the next six months, but if maternal control was high, then the child maintained a higher weight level, possibly indicating an absence of self-regulation (145).
Another subset of studies examines the effect of early nutrition on children’s eating behavior. This research is in its own infancy, but there are suggestions that not only over-feeding but also the low protein content of breast milk might lead to hyperphagia, disrupted energy balance, and ultimately to weight gain (146). Others suggest that the influence of maternal diet may even begin during pregnancy. For example, the “thrifty phenotype” hypothesis (147, 112) posits that both fetal and early postnatal malnutrition can lead to adaptations in glucose metabolism that give rise to a greater risk of metabolic syndrome and obesity, especially when they were followed by a period of plentiful nutrition. The mechanisms for these adaptations are not yet well understood, but may include epigenetic processes in which nutritional influences affect fetal DNA (148), e.g., maternal under- or over-nutrition may cause alterations in methylation of genes controlling lipid and carbohydrate metabolism, or neural networks controlling appetite–energy balance (149).
Parental Feeding and Food Cue Responsiveness
In one of the first studies to explore the effect of parental feeding on food cue responsiveness, Fisher and Birch (150) conducted the Eating in the Absence of Hunger (EAH) test with 71 preschool children (aged 3–5 years). Parents completed a scale assessing degree of restriction for each of the snack foods used. There was a significant interaction between child sex and restriction, such that child and maternal reports of restriction predicted higher snack food intake, but only in girls. Subsequent studies from the same research group found similar cross-sectional results in girls, and more recent studies from the same group used longitudinal analyses to demonstrate that parents’ reports of restricting their daughters’ access to foods at 5 years of age predicted EAH at 7 years (151), and that girls who were overweight at 5 years and received higher levels of restriction had the highest EAH scores at 9 years, and the greatest increases in EAH from 5 years to 9 years (73).
A few studies have also experimentally manipulated restriction and assessed effects on immediate intake. In one study, 3–5-year-old children participated in four unrestricted snack sessions followed by four restricted snack sessions (152). A “target” snack, initially liked very strongly, was selected for each child, reflecting the foods restricted by most parents. In the unrestricted sessions, children had unlimited access to both their target snack and an alternative well-liked snack. In the restricted sessions, they had free access to the alternative food, but only five minutes of access to the target food. Children made significantly more positively valenced comments about the target food, as well as more approach behaviors toward it, in the restricted condition, and both intake and snack selection were significantly greater for the target food, suggesting that restricting foods might increase their intake of those foods when they are available (i.e., increase responsiveness to those foods). Building on this evidence, a more recent behavioral study found similar results and further demonstrated that children whose parents imposed either very low or very high levels of restriction at home consumed more energy throughout the procedure (153). This suggests that influence of restriction on eating behavior could be non-linear, although parental responses to children’s eating behavior and weight, and/or qualitatively different styles of restriction at either end of the restriction scale, could also explain the results.
Other studies have used psychometric rather than behavioral measures of food responsiveness. For example, one study assessing 5-year-old girls’ perceptions of parental feeding styles reported a positive association between pressure to eat and dietary restraint and emotional eating, and between restriction and external eating (154). Another study showed a link between higher perceived pressure to eat and higher emotional and external eating among 7–12-year-old boys, and a positive association between perceived restriction and dietary restraint in both boys and girls, although perceived restriction was also associated with lower levels of emotional and external eating (155). In our own sample of nearly 400 twin children, we have shown positive cross-sectional associations between a number of parental feeding behaviors (restriction, using food as a reward [instrumental feeding], and using food to influence the child’s feelings [emotional feeding]), and food cue responsiveness as assessed by the CEBQ Food Responsiveness scale, while parental pressure to eat was positively associated with CEBQ Satiety Responsiveness, Slowness in Eating and Food Fussiness and negatively associated with Enjoyment of Food, independent of BMI (156). Together, these findings show some mixed support for a model in which some parental feeding practices could influence children’s appetitive traits although the data do not allow conclusions to be drawn regarding causation.
Parental Feeding and Food Preferences
There is a substantial literature examining cross-sectional associations between measures of parental feeding and children’s actual dietary intake. Some of this research has highlighted relationships between certain feeding strategies and intake of fruit and vegetables (157–162) and energy-dense items such as high-fat foods, sweets, and soft drinks (158, 159, 163, 164), all of which could be relevant to children’s weight. However, these results indicate only that parental feeding styles might affect children’s diets when they are under the control of the parent. We focus here on experimental studies and retrospective survey studies that hint that parental feeding styles might influence the development of enduring food preferences, i.e., underlying traits that could go on to determine dietary choices without the presence of the parent.
We have already discussed experimental evidence suggesting that restricting foods may increase preferences and intake (150). However, perhaps the most persuasive evidence that parental feeding influences food preferences comes from research on instrumental feeding, or use of food in a “means–end contingency.” A food item may occupy either or both places in a contingency. For example, it might be used as the route to a non-food reward (“If you eat your peas, I’ll take you to the movies”), or as a reward in itself, used to reinforce desirable behavior (“If you tidy your room you can have an ice cream”). Parents commonly employ healthy foods as means, and less healthy foods as ends, sometimes simultaneously (“If you eat your peas, you can have an ice cream”).
To date, the literature suggests that using a food as a reward increases preference for that food, while using food as a means to an end decreases preference for it. For example, Birch, Zimmerman, and Hind (165) presented children with food rewards for performing certain behaviors and found that preference for the reward food increased and was maintained for six weeks following the intervention. Birch and colleagues have also tested the effect of using food as a means to an end. Birch, Marlin, and Rotter (166) used movie tickets and verbal praise to reward preschool children for consuming an initially novel beverage (flavored milk) and found that post-intervention preferences for the milk were lower than those of children in a mere exposure condition. Newman and Taylor (167) demonstrated similar effects with food rewards, finding that 4–7-year-olds whose consumption of a target snack (Snack A) was rewarded by consumption of another snack (Snack B) showed decreased preferences for Snack A relative to Snack B, compared to similar temporal order and mere exposure control groups. One survey study found that instrumental feeding (using food as a reward) and emotional feeding (in response to a child’s distress) were positively associated with snacking behavior assessed by food frequency questionnaire in 6–7-year-olds, while encouragement to eat was negatively associated with snacking (168), and another found that using food as a reward was associated with increased intake of unhealthy foods in 1–10-year-olds, while parental modeling of healthy food intake was associated with increased intake of healthy foods (169). These findings suggest that, when parents use less healthy foods to reward the consumption of healthy foods, they may increase preferences for the former, while simultaneously decreasing preferences for the latter.
Three studies have used a retrospective design to investigate the association between adult weight and reported parental feeding. One study examined the influence of pressuring children to eat, finding that 72% of college students who had experienced forced consumption in childhood reported that they would not willingly eat the target food on the day of questioning (170). However, it is not clear whether forced consumption caused dislike or dislike motivated the parent to try to force consumption. Another study surveyed a community sample of 122 males and females, and found that recollection of parents’ use of controlling feeding strategies, such as rewarding and punishing eating behavior, was associated with binge-eating and restraint in adulthood. Recollection of parental restriction was additionally associated with binge-eating as an adult (171). However, Brunstrom, Mitchell, and Baguley (172) found no relationships in university students between recalled parental feeding strategies and current dietary restraint or overeating.
Current Thinking in Parental Feeding Research
These results provide some support for parental influence on eating behavior traits, but each method has its limitations. The experimental evidence uses only analogues for parental control; the cross-sectional evidence cannot tell us about cause and effect; and the retrospective evidence may be biased. Even the prospective results do not give conclusive proof: it is always possible that a third variable (e.g., genetic influences on development) may influence eating traits and parental feeding strategies, while feeding strategies may actually result from parents responding to their child’s eating behavior, an instance of genes (of the child) affecting the immediate environment (parental behavior); this process can be considered an example of evocative gene–environment correlation) (134).
A good example of the ambiguity of such results can be seen in the literature examining the relationships between parental restriction and pressure to eat, and child eating behavior and weight. Although parental behavior is traditionally conceived as the independent variable and child variables as dependent, associations between higher restriction and higher weight are increasingly interpreted as possible reflections of parents’ attempts to modify eating behavior in their overweight child (137, 173) in much the same way as restraint is considered an individual’s attempt to modify his or her own weight. Similarly, the association between higher pressure to eat and lower weight is most parsimoniously interpreted as the parent attempting to increase intake in a child they see as too thin (174, 175). If parental feeding styles are responses to, as much as causes of, child weight, we would expect concern about child weight to mediate the relationship between parental restriction and child weight, and this is indeed what we find (176). Further, if parental feeding styles are partly influenced by children’s genetically influenced characteristics, we would also expect parents with more than one child to report responding differently to a sibling, and this has been illustrated by qualitative data (177). The “maternal responsivity” perspective is consistent with previous reports of parental restriction being related to concern about overweight but not related to overweight itself (178) and the failures to observe longitudinal effects of feeding practices on children’s fat mass in ethnically and socioeconomically diverse samples (179).
The potential importance of the interaction between parental feeding behavior and the child’s disposition is neatly illustrated by one observational study that found that obese mothers did not prompt their children to eat any more frequently than lean mothers did, but that prompts were more likely to be associated with BMI z-score in these children, suggesting that some children may be more responsive to environmental cues to eat and hence more likely to become obese (180). Longer-term prospective studies and genetic designs may be able to shed further light on causal mechanisms.
There is also a groundswell of interest in expanding research on the influence of parental feeding to include more subtle, child-responsive, authoritative feeding strategies, in addition to the overt attempts to control feeding that have been the focus of many of the studies. For example, one group used scores on their Caregiver Feeding Styles Questionnaire to classify parents along dimensions of demandingness and responsiveness in feeding into authoritative, authoritarian, indulgent, and uninvolved feeding styles (181). A subsequent study demonstrated that authoritative parents had children with the lowest BMI z-scores (182), while another found that indulgent feeding was associated with higher child BMI (183). Ogden and colleagues (184) propose a new measure of “covert control” of children’s snacking behavior, designed to tap subtle control of the availability and accessibility of particular food types, and including items such as “Do you avoid buying sweets and crisps and bringing them into the house?” “Do you avoid going to cafés or restaurants with your children which sell unhealthy foods?” “Do you try not to eat unhealthy foods when your children are around?” Musher-Eizenman and Holub have combined constructs from a variety of questionnaires together to give more comprehensive coverage of parental feeding styles in one measure (185).
Other studies have found that individual preferences for certain foods can be modified through taste exposures and modeling (186). For example, teacher-led (187) and parent-led (188) exposure to a particular vegetable occurring daily for two weeks increased children’s liking and intake for that vegetable. Additionally, early introduction and parental consumption of fruits and vegetables have been associated with increased fruit and vegetable consumption in 2–6-year-olds (189), while a survey of 550 families with preschool children found that parental intake of fruits and vegetables was the strongest predictor of their child’s intake (190). Taking a broader view of parental feeding style may therefore allow us to understand more fully the potential for both desirable and undesirable effects on children’s eating styles.
Implications for Clinical Practice
We have described a variety of studies suggesting that certain eating behavior traits are associated with individual differences in weight, and other studies suggesting that these traits are influenced partly by genetic factors and partly by early nutrition and parental feeding practices. The clinical implications of this array of evidence are wide-ranging. For example, it may already be possible to identify individuals with a phenotypic profile predisposing them to weight gain using behavioral and psychometric tests. With advances in neuroimaging and genetic research we may one day be able to identify risk by more objective means. For example, several studies have now shown that polymorphisms in FTO may have some of their effects through appetite (129, 133–136). The cumulative discovery of other genetic variants contributing to appetite and weight may one day make genetic screening a possibility, although since each new variant discovered seems to explain only a tiny additional increment of the variance (191), this still seems a long way off.
Nevertheless, early identification of “high-risk” individuals would make it possible to intervene before weight has crept up and overweight has become intractable. For example, if satiety responsiveness is low, then portion control will be crucial and serving low-energy density foods may help to limit the damage of eating everything on the plate. If innate preferences push individuals toward obesogenic food choices, then finding similar-tasting, low-energy versions of liked foods may prove useful. If individuals show heightened responsivity to food cues, then learning to limit cue exposure (i.e., stimulus control) will be critical, and if eating is relatively more rewarding than other activities for those individuals, then it may help to encourage conscious substitution of an enjoyable but less “risky” alternative behavior (192).
There is also scope for targeted treatments to alter dispositions. For example, Johnson (193) successfully improved intake regulation in preschoolers using a doll to demonstrate how to eat according to internal satiety cues (see Box 9-1 for more details). Another trial in which extremely obese adolescent girls were trained to regulate their eating rate with the aid of computerized feedback has shown promising results in terms of both satiety sensations (as shown by reduced portion size) and weight loss (194, 195). It may also be possible to improve self-control using cognitive strategies to regulate physiological and neurological responses to reward (195).
Box 9-1 Improving Preschoolers’ Self-Regulation of Energy Intake (147): Intervention details
In this innovative study, preschoolers participated in a six-week intervention taking place in their normal childcare setting. On the first day, researchers performed a skit illustrating cues that signal hunger (rumbling in the stomach), fullness (stomach extension, satisfaction), and overeating (stomach distension, discomfort). Children were introduced to the words “mouth” (where you chew food), “esophagus” (where food goes when you swallow), and “stomach” (where swallowed food goes). One week later children watched a video of “Winnie the Pooh and the Honey Jar” and the researchers talked about hunger and the implications of overeating for weight gain. During the next week children were introduced to dolls with nylon “stomachs” (filled to varying degrees with salt) to help them think about hunger and satiety. Then, for one day per week over a four-week period, children were seen at snack times. During the snack researchers talked to children about how hungry or full they felt, and before and after the snack they were asked to identify the doll stomach that was most like the state of their own. Caloric compensation was assessed before and after the intervention and significantly improved, suggesting that interventions like this may help to improve children’s intake regulation.
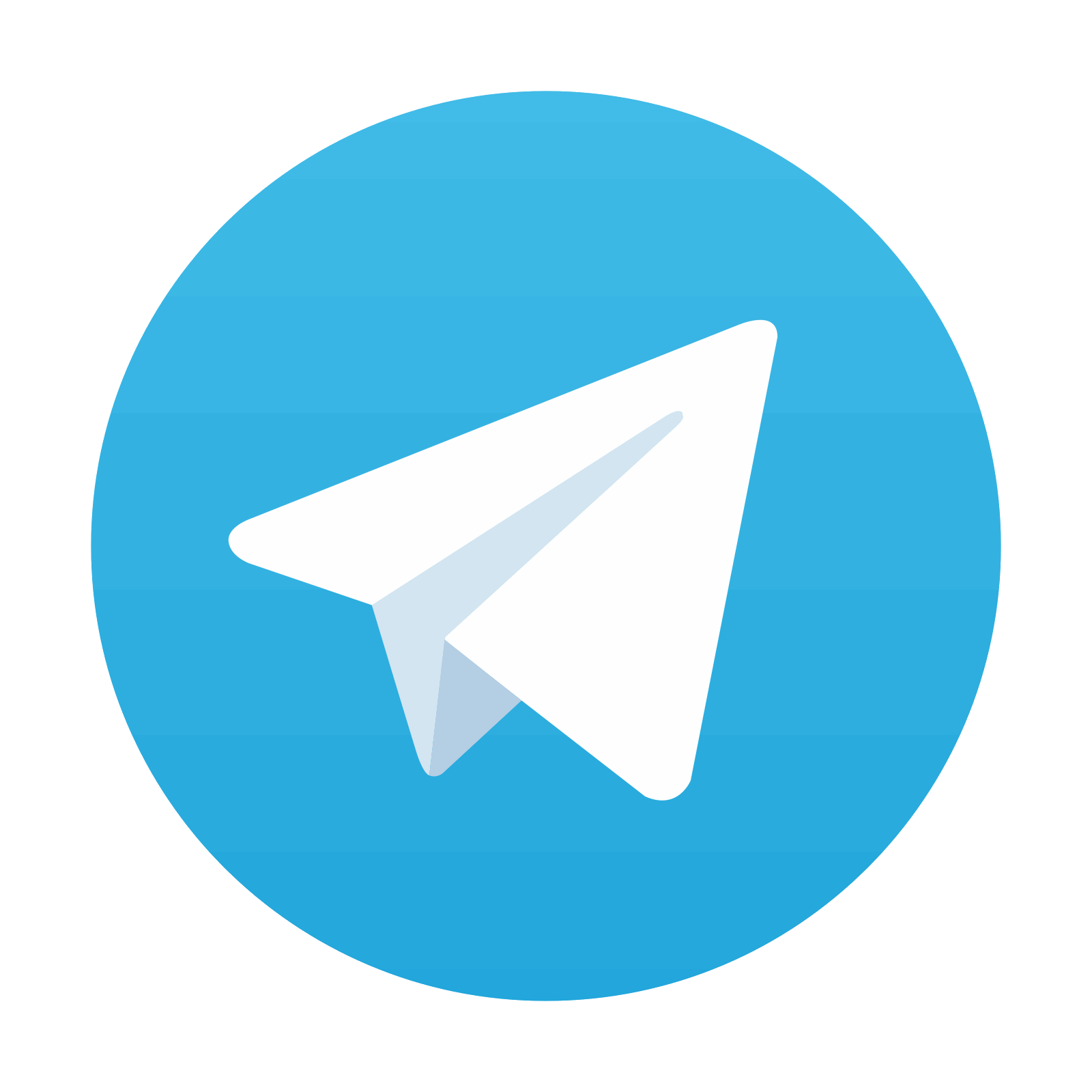