EEG and Epilepsy
Thoru Yamada
Elizabeth Meng
Paroxysmal Discharges and Seizure Diagnosis
Since the introduction of CT (computerized axial tomography) in the early 1970s, neuroimaging diagnostic tests such as MRI (magnetic resonance image), fMRI (functional MRI), PET (position emission tomography), and SPECT (single photon emission computerized tomography) have made remarkable advancements in revealing the anatomical as well as functional disturbances of brain lesions. Despite these significant contributions to the diagnosis of various neurological disorders, EEG continues to play a pivotal role in the diagnosis and management of patients with epilepsy.
In evaluating the EEG of a patient with possible seizures, we may see interictal epileptiform discharges (IEDs) and/or nonspecific paroxysmal discharges, with or without focal or diffuse slowing. IEDs, represented by spike or spike-wave discharges, are the most sensitive and specific markers for the diagnosis of seizures. Other paroxysmal discharges represented sharp, alpha, theta or delta pattern is less specific for diagnosis of epilepsy. In a routine EEG (a recording of ˜30 minutes), the chance of recording a clinical seizure (ictal) event is rather rare, unless the patient is having frequent seizures or is in status epilepticus. Thus, we often rely primarily on IEDs for the diagnosis of epilepsy.
The likelihood of detecting IEDs varies depending on seizure type, age, and seizure frequency. An EEG that includes sleep or that is recorded after sleep deprivation increases the yield of IEDs. Generally, greater seizure frequency is associated with a higher yield of IEDs.1 IEDs are also recorded more often in children than in adults. Detection of IEDs differs depending on the origin of the epileptiform activity: if a relatively small area of the cortex is involved as the epileptogenic zone, IEDs may not be detected by scalp electrodes. Also, epileptiform activity arising from deep brain structures such as the medial temporal lobe, subfrontal lobe, or interhemispheric medial cortex may not be readily recorded using scalp electrodes.
The specificity of IEDs is determined by the incidence of IEDs in the normal populations (false positive) compared with that in patients with epilepsy. IEDs are found in 1.9% to 3.5% of healthy children2,3 and 0.5% of healthy adults.4 Specificity also varies depending on the type of IEDs: only about 40% of patients with benign Rolandic spikes of childhood or BECTS (benign epilepsy of childhood with central midtemporal spikes) and 50% of patients with childhood epilepsy with occipital paroxysms (benign occipital spikes of childhood) have a history of seizure.5 Also, IEDs elicited by photic stimulation and generalized spike-wave discharges are less correlated with seizure history compared to focal spikes. Multifocal IEDs and focal IEDs, especially at the midline, frontal, and anterior temporal regions, are highly (75% to 95%) correlated with a history of seizures.5,6 Overall, the incidence of detecting IEDs during the first EEG in adult epilepsy patients is about 30% to 50%.7,8 In children less than 10 years old, the incidence is about 80%. Repeating the EEG once increases the yield by an additional 20% to 30%.7,8
A frequently asked question is whether anticonvulsant medication affects the incidence of IED detection. It is the general consensus that the use of oral phenytoin (Dilantin) or carbamazepine (Tegretol) does not decrease IEDs.7 Thus, the patient does not need to discontinue anticonvulsants prior to having an EEG. However, valproate suppresses generalized spikewave bursts and photoparoxysmal responses.9 The intravenous administration of benzodiazepine such as lorazepam (Ativan), diazepam (Valium), or clonazepam (Klonopin) is a potent treatment regimen for status epilepticus abolishing generalized spike-wave discharges,10 but oral administration of benzodiazepines has little effect on IED. The newer anticonvulsants such as levetiracetam (Keppra),11 topiramate (Topamax),12 and lamotrigine (Lamictal)13 are reported to decrease IEDs, while oxcarbazepine (Trileptal)14 and vigabatrin (Sabril)15 may enhance generalized spike-wave discharges with an increase of generalized seizures in patients with focal seizure. The effects on IEDs by other anticonvulsants including gabapentin (Neurontin), pregabalin (Lyrica), felbamate (Felbatol), and zonisamide (Zonegran) are still unclear. Lacosamide (Vimpat), introduced recently for the treatment of pharmacoresistant epilepsy or status epilepticus, was reported to show no significant EEG changes in terms of power spectrum, mean frequency, and interictal abnormalities.
Although it is preferable to do the first EEG recording without anticonvulsants, these medications should not be discontinued for the purpose of an EEG study because abrupt discontinuation of the anticonvulsants may put the
patient at increased risk of seizure, even worse, into status epilepticus. Therefore, withdrawing anticonvulsants for the purpose of capturing seizures, especially for poorly controlled seizure patients, must be done in an inpatient setting.
patient at increased risk of seizure, even worse, into status epilepticus. Therefore, withdrawing anticonvulsants for the purpose of capturing seizures, especially for poorly controlled seizure patients, must be done in an inpatient setting.
The Epilepsies
Certain forms of epileptic seizure disorders have special clinical and EEG characteristics irrespective of their etiologies. The International Classification of Epilepsies and Epileptic Syndromes was proposed by the Committee on Classification and Terminology of the International League Against Epilepsy.16 This classification is based on two principles: distinguishing first between localized (focal) and generalized epilepsies and secondly between idiopathic and symptomatic etiologies. However, the classification, which includes detailed categories of the epileptic syndromes, has elicited some disagreement and controversy among epileptologists.
Normal or near-normal background activity is characteristic of idiopathic epilepsy, and slowing of background activity or multifocal epileptiform activity is suggestive of symptomatic epilepsy. Also, focal background abnormality and/or focal polymorphic delta activity is likely correlated with symptomatic epilepsy.
LOCALIZED (FOCAL) EPILEPSIES AND SYNDROMES
In the localized epilepsies and syndromes, examples of the idiopathic type include benign childhood epilepsy with centrotemporal spikes (Rolandic spikes or BECTS) and childhood epilepsy with occipital paroxysms (benign childhood occipital lobe epilepsy). Symptomatic localized epilepsies and syndromes include frontal or temporal lobe seizures that are secondary to focal pathology.
The localization of an epileptogenic focus often determines the character of the seizures. To some extent, it is possible to speculate on the clinical manifestation of seizures based on the localization and waveform of the epileptiform activity. Conversely, it may be possible to postulate the localization and waveforms of epileptiform activity based on clinical seizure types.
A focal spike or sharp wave commonly has surface-negative polarity associated with a distant field of positivity, thus forming a dipole field distribution. When spikes arise from the crown of a cortical gyrus, a radially oriented dipole is created with the negative field projecting to the cortical surface and positivity in the deeper areas of the brain (see Fig. 8-22A). When spikes arise from the sulcus of the cortical surface, a tangentially oriented dipole is created, with both positive and negative poles appearing on the cortical surface (see Fig. 8-22B). Discretely localized negative spikes likely arise from the cortical surface near the detecting electrodes, whereas widespread spikes may arise from the deeper structures. Unfortunately, predicting the source of spikes from scalp electrodes is limited because cortical spikes are much attenuated and the field distribution is distorted when detected at the scalp by intervening nonhomogeneous volume conductive media (scalp, skull, CSF, etc.) between the cortex and the scalp. In general, however, reasonable correlates exist between the partial seizure type and sites of interictal discharges.
Of the following sections in this category, the first and second sections are idiopathic and third through sixth are generally symptomatic.
Benign Epilepsy of Childhood with Central Midtemporal Spikes
Spikes maximally recorded from central or midtemporal electrodes have been described as BECTS or “benign Rolandic epilepsy.” The term “benign” in this sense means a good prognosis for this seizure type. The waveform is more commonly “sharp” rather than a true spike, having a triphasic configuration with a prominent negative peak, preceded and followed by small positive peaks. The negative field centered at the central or midtemporal electrode is commonly associated with positive fields in the frontal region (Fig. 10-1A and B). These discharges are often unilateral, but one third of the patients have bilateral independent foci. Sleep (non-REM) greatly increases the spikes (see Fig. 9-13A and B) and about one third of the patients have spike discharges only in sleep.17 Also, unilateral spikes in the awake state may progress to bilaterally independent spikes in sleep. Rolandic spikes tend to decrease progressively with age and eventually disappear by the midteen years.18 The onset of seizures usually occurs between 4 years of age and adolescence. Consistent with the typical dramatic increase of spike discharges in sleep, about 80% of seizures occur exclusively in sleep.17
Clinically, seizures initially consist of unilateral paresthesias of tongue, lips, cheek, and gum and/or unilateral tonic-clonic activity of facial and pharyngeal/laryngeal muscles, contralateral to the side of the spike focus. The ictal discharges often start with an initial electrodecremental pattern (EEG flattening) followed by rhythmic spike bursts with subsequent spikewave discharges. The seizure ends without postictal slowing unless the seizure evolves to a generalized tonic-clonic convulsion, which is not uncommon.
Not all spike discharges from central regions are benign. Some features help distinguish benign Rolandic epilepsy from symptomatic (non-benign) epilepsy. As shown in Figure 10-1A and B, benign Rolandic spikes are tangentially oriented (see Fig. 8-22B), with a negative field just behind the Rolandic fissure either at or close to the central or midtemporal electrode and a positive field over the frontal region. In symptomatic epilepsy, spikes often have a radially oriented distribution; thus, the negative field spreads diffusely over a wide scalp region (see Fig. 8-22A).19 Focal slowing (corresponding to the side of spikes) is absent in benign Rolandic epilepsy but is often present in symptomatic epilepsy (Fig. 10-2). When a Rolandic spike is maximum at the midtemporal electrode, differentiation of temporal lobe epilepsy with spikes at the T3 or T4 electrode from benign Rolandic epilepsy can be more difficult. In this case, additional electrodes between C3 or C4 and T3 or T4 (C5 or C6 according to the expanded 10 to 20 system: see Fig. 2-4) help distinguish the two: Rolandic spikes are maximum at C5 or C6, whereas symptomatic temporal lobe seizures likely have a maximum at T3 or T4.20
Childhood Epilepsy with Occipital Paroxysms and Other Occipital Spikes
There are two types of seizures categorized in this entity: late onset, originally described by Gastaut21 (Gastaut type), and early onset, more recently described by Panayiotopoulos,22 and
now referred to as Panayiotopoulos syndrome. Affected children are neurologically normal in both types. Family history of epilepsy is positive in more than one third of patients for the late-onset type but negative for the early-onset type. In the late-onset variant, age of seizure onset ranges from 15 months to 17 years with peak age of 7 to 9 years.23 In the early-onset variant, onset is between 1 and 14 years with peak age of 3 to 6 years.24
now referred to as Panayiotopoulos syndrome. Affected children are neurologically normal in both types. Family history of epilepsy is positive in more than one third of patients for the late-onset type but negative for the early-onset type. In the late-onset variant, age of seizure onset ranges from 15 months to 17 years with peak age of 7 to 9 years.23 In the early-onset variant, onset is between 1 and 14 years with peak age of 3 to 6 years.24
In the late-onset type, seizures almost always begin with visual symptoms (blindness, scintillating scotoma, visual hallucinations, or illusions). The seizure is usually brief, lasting only a few to several seconds. Half of the patients complain of severe migraine-like headache associated with nausea and vomiting.21 Thus, differentiation from migraine headache is sometimes difficult. The prognosis is good overall but less favorable than that of BECTS.
In the early-onset type, seizures lack characteristic visual symptoms but consist of a variety of autonomic symptoms including “feeling sick,” paleness, nausea and vomiting, cyanosis, miosis or mydriasis, and cardiopulmonary irregularities. The seizure lasts much longer (5 to 10 minutes) than the lateonset type and often ends with a hemiconvulsion, Jacksonian march, or generalized motor activity. About one third of seizures occur in sleep. Nearly all patients become seizure free by age 12.24
EEG abnormalities are indistinguishable between these two types of seizures. Spikes consist of high-amplitude surface-negative spikes (200 to 300 µV), often followed by a small positive and negative slow wave (Fig. 10-3). These occur singly or more commonly in serial, semirhythmic bursts with a unilateral or bilaterally independent appearance. Eye opening tends to abolish the spike wave, and eye closing may precipitate the burst. However, neither repetitive photic stimulation nor hyperventilation has an effect.23 As with BECTS, sleep (NREM) increases the discharges, but less prominently.
About one fourth to one third of patients have other epileptogenic abnormalities including generalized spike wave or BECTS23,25 (Fig. 10-4). The ictal EEG consists of rhythmic spike discharges starting from one occipital region, evolving into rhythmic theta-delta that spreads to the contralateral occipital region (Fig. 10-5).
Benign childhood occipital lobe epilepsy must be differentiated from other occipital spikes. Many patients with congenital or acquired amblyopia are found to have occipital spikes, but the morphology of the spike is much faster (“needle-like spike”) than that of benign childhood occipital epilepsy.26 Occipital spikes may also occur in idiopathic, generalized seizure patients with a photoparoxysmal response27 (Fig. 10-6A and B). Irrespective of age, patients may have localizationrelated seizures with vivid visual hallucinations.28 Although many “pure” partial/focal seizures fail to show ictal discharges, partial seizures of occipital origin tend to show well-localized ictal EEG activity, and the patient is often able to describe his or her visual hallucinatory event in detail (Fig. 10-7A-D; see Video 10-5A).
Temporal Lobe Seizures
Temporal lobe seizures are far more common than any other seizure type. Clinical manifestation of temporal lobe seizures reflects the multitude of temporal lobe functions. In these seizures, the patient often maintains consciousness but has impaired awareness. A very common clinical finding is the presence of automatisms. Automatisms are stereotyped, repetitive movements such as picking at clothing, lip smacking, and chewing (Video 10-1 with Fig. 10-8). Because of characteristic psychic and motor symptoms, temporal lobe seizures were previously called “psychomotor seizure” but are now classified as “complex partial seizure” or “focal seizure with impaired awareness.*” Some patients may remain conscious and have
seemingly normal behavior despite EEG showing widespread ictal discharges (Video 10-2 with Fig. 10-9).
seemingly normal behavior despite EEG showing widespread ictal discharges (Video 10-2 with Fig. 10-9).
Temporal lobe seizures can be divided into those originating in the mesial temporal lobe and those from the neocortex. Because many candidates for epilepsy surgery have temporal lobe seizures, it is important to differentiate these two foci before surgery. This may be difficult with scalp EEG alone, but there are some clinical and EEG features that help distinguish the two types.29
MESIAL TEMPORAL LOBE EPILEPSY. Details of the clinical manifestation of temporal lobe seizures are beyond the scope of this chapter. More than 80% of the patients with mesial temporal lobe epilepsy (MTLE) have a history of febrile seizures in early childhood. Seizures often begin with a variety of prodromal symptoms, that is, “aura,” which most often consists of gustatory (e.g., a metallic taste) or olfactory (e.g., a smell of burning rubber) hallucinations. The patient may have a variety of illusional or hallucinatory experiences. An illusion is the distorted perception of an object, such as micropsia (e.g., an elephant looks as small as an ant), macropsia (e.g., a child looks as big as a giant), or another distortion of form. A hallucination is the perception of nonexisting objects. This may be visual, auditory, olfactory, or gustatory. Other relatively common symptoms are “déjà vu,” in which unfamiliar objects or experiences seem familiar, and “jamais vu” in which familiar objects or experiences seem unfamiliar. Other relatively uncommon symptoms include “ideational symptomatology,” which consists of sudden, forced thought processes, and “affective symptomology,” which consists of states of extreme sadness or pleasure.
The interictal EEG of MTLE shows spike or sharp discharges of maximum negativity at the anterior temporal electrodes (F7/F8 or T1/T2). This relatively discrete negative field is accompanied by a more widespread positive field over the contralateral central-parietal region (Fig. 10-8). About one third of MTLE patients have bilateral IEDs that occur independently on the two sides.30,31 MTLE is usually associated with mesial temporal sclerosis, which is often revealed by MRI.32 Video 10-3 A&B show beta onset seizures arising from left or right temporal region independently secondary to mesial temporal sclerosis. The ictal EEG is often characterized by rhythmic theta or delta discharges from anterior/midtemporal electrodes at the onset, with subsequent spread to other electrodes (see Videos 10-1 and 10-2). The onset of the ictal discharge usually starts within 30 seconds after the onset of clinical symptoms or signs.33,34
NEOCORTICAL TEMPORAL LOBE EPILEPSY. Clinical manifestations of neocortical temporal lobe epilepsy (NTLE) are less well characterized than those of MTLE. Epigastric auras are less common in NTLE, as are oroalimentary automatisms, visual and auditory hallucinations, head movement, and contralateral dystonic posturing.35
The interictal EEG features of NTLE are also less consistent and less specific than MTLE. Interictal spikes of NTLE tend to have a broader negative field and lack a positive field over the contralateral central-parietal regions.36 An ictal onset with localized beta activity generally implies that seizure arises from the electrode nerby the beta onset zone. But this rule does not necessarily applies to NTLE as shown in Video 10-3 A&B. An example of NTLE secondary to a posterior temporal lesion is shown in Figure 10-10.
Distinction between MTLE and NTLE usually cannot be made with confidence based on scalp-recorded EEG alone because of the overlap in ictal or interictal EEG discharges between the two entities. Also, there are similarities in the seizure semiology. Some differences have been reported; history of febrile seizure and congenital malformation are more common in MTLE than NTLE.37 Abdominal aura is more common in MTLE, while ictal dystonia is more common in NLTE. Maximum interictal spikes involving sphenoidal or F9 or F10 electrodes (modified 10 to 20 system nomenclature; see Fig. 2-4) occur more likely in MTLE. The maximum spike location in NTLE tends to be more posteriorly located, involving T7 or T8, TP7 or TP8, and P7 or P8 electrodes.37 Despite some of these differences between MLTE and NLTE, the correct diagnosis must rely on the findings derived from EEG data recorded by subdural (cortical) and/or depth electrodes.
Frontal Lobe Seizures
The clinical symptoms of frontal lobe seizure are reflected by heterogenic functions of the frontal lobe and propagation of seizure
activity from its origin to different areas of the frontal lobe. There are essentially four manifestations of frontal lobe seizures:
activity from its origin to different areas of the frontal lobe. There are essentially four manifestations of frontal lobe seizures:
Simple partial seizures arising from the primary motor cortex manifesting as clonic twitching of contralateral muscles of the body, without loss of consciousness. According to the somatotopic arrangement of the precentral gyrus, the clonic movements are initially limited to the corresponding area of the body and then spread to other areas; for example, from finger to arm, to shoulder, and then to the face. This is known as a Jacksonian march.
Seizures arising from the supplementary motor cortex, consisting of deviation of head and eyes to one side (usually contralateral to the seizure focus), associated with tonic elevation of contralateral arm and downward extension of the ipsilateral arm. Thus, the patient appears to look at the raised arm. Depending on the extent of spread, the patient may or may not lose consciousness.
Immediate unconsciousness followed by a generalized tonic-clonic convulsion with minimal or no lateralizing signs. This may arise from the superior frontal region.
Complex motor manifestation characterized by vigorous body rocking, bicycling, dystonic posturing, repetitive movements, laughing, crying, etc. (Video 10-4). Because these movements or behaviors appear very bizarre and the EEG recording during seizures may not show any EEG change or ictal pattern, misdiagnosis as a nonepileptic spell or pseudoseizure is not uncommon. These seizures typically emanate from the medial frontal or orbital-frontal areas, but may arise anywhere within the frontal lobe.
As frontal lobe seizures often arise from the orbital-frontal cortex, interhemispheric convexity (medial frontal lobe), or cingulate cortex—all of which are relatively inaccessible to scalp EEG—it is not uncommon to miss interictal or even ictal discharges on scalp EEG. If IEDs are recorded, however, they may appear widespread and less localized because of the deep epileptogenic source. About two thirds of patients, especially those with medial frontal foci, show secondary bilaterally synchronous discharges.38 Because spread from one hemisphere to the other is so rapid, it is not always possible to distinguish if the discharges are of frontal lobe origin or represent a primary generalized seizure. About half of the patients with frontal lobe epilepsy have IEDs in one frontal lobe only or with consistently unilateral dominance (Fig. 10-11A and B).38,39
The ictal EEG is nonlocalizing in more than half of the patients with frontal lobe epilepsy.40 Even if there are ictal EEG changes associated with clinical seizures, they are often obscured by large muscle and movement artifacts that result from the seizure. This contrasts to temporal lobe epilepsy in which patients are typically quiet and motionless at the time of seizure onset; thus, artifacts are less problematic.
At the onset of a frontal lobe seizure, EEG often shows diffuse or bifrontal dominant voltage attenuation, which correlates with tonic posturing. This is followed by bilateral frontal or diffuse rhythmic theta-delta or spike-wave bursts correlating with clonic movements. In contrast, seizures of dorsolateral frontal origin are usually associated with focal or lateralizing rhythmic fast activity at seizure onset.41
Parietal Lobe Seizures
Parietal epilepsy is far less common than temporal or frontal lobe epilepsy. As with frontal lobe epilepsy, scalp EEG is often falsely negative, nonlocalizing, or falsely localizing.42 Seizures of parietal origin have no typical clinical features. Seizures often present with visual disturbances such as scintillations or oscillopsia. If the seizure arises from the sensory cortex, symptoms may include a variety of sensations such as tingling, “pins and needles,” numbness, pain, burning, or cold.43 Strictly, sensory focal seizures are rather uncommon. Pain as an ictal sensation may be seen in temporal lobe seizures rather than in parietal lobe seizure.44
Occipital Lobe Seizures
Reflecting occipital lobe function, occipital lobe seizures may exhibit blindness or visual field loss or visual misconception such as macropsia, micropsia, misconception of spatial orientation, and hallucination such as seeing simple flashing light or color45 or various more complex objects.46 Interictal spikes
are localized to the occipital electrodes, and ictal events often start with beta activity with progressive amplitude increase and slowing in frequency as ictal discharges spread to other region (see Figs. 8-8 and 10-7). Unlike other simple partial seizures, which often show no EEG change even when the patient is having a seizure, the simple partial occipital seizures often show electrographic ictal (seizure) patterns, and the patient is able to describe clearly what he or she is seeing (Video 10-5A and B).
are localized to the occipital electrodes, and ictal events often start with beta activity with progressive amplitude increase and slowing in frequency as ictal discharges spread to other region (see Figs. 8-8 and 10-7). Unlike other simple partial seizures, which often show no EEG change even when the patient is having a seizure, the simple partial occipital seizures often show electrographic ictal (seizure) patterns, and the patient is able to describe clearly what he or she is seeing (Video 10-5A and B).
Although most of photic induced spike-wave bursts (photoparoxysmal response) are associated with primary generalized seizures and not focal seizures, parieto-occipital spikes are exception (see Fig. 9-7). The seizure semiology is similar to other occipital seizures with a sequence of visual symptoms, but it is sometimes accompanied by headache and vomiting.47 Therefore, this could be misdiagnosed as migraine headache with visual symptoms. Another type of occipital seizure is the benign nature of childhood epilepsy with occipital spikes (see Figs. 10-3, 10-4 and 10-5; see also section of Occipital Epilepsy with Occipital Paroxysms and other occipital spikes in this chapter). The symptoms also include migraine-like headaches.21,22,23,24 In some congenitally blind patients, occipital spikes with needle-like morphology can be observed, but these spikes are asymptomatic and usually disappear during childhood or adolescence.26,48 Another rare condition showing interictal occipital spikes/polyspikes is Dravet syndrome, which presents with severe myoclonic seizures in infancy.49,50 Occipital spikes eventually become generalized spike/polyspike. Some show photoparoxysmal response.
Other EEG Abnormalities Associated with Focal Epilepsy
MULTIFOCAL SPIKE DISCHARGES. Multifocal spikes, either within one hemisphere or from heterogeneous areas of each hemisphere (Fig. 10-12; see also Fig. 8-18), generally correlate with multifocal or diffuse brain diseases such as the intellectually disabled or various neurological deficits. Slowing of the background rhythm as well as diffuse slow waves is common in these patients. The majority of patients have frequent seizures that are often intractable to treatment. Generally, increased spike frequency correlates with increased incidence of seizures and a greater degree of intellectual disability.51 Multifocal spikes are also seen in patients with West syndrome (infantile spasm with hypsarrhythmia; see “Infantile Spasms, Salaam Spasms, West Syndrome” in this chapter; see Fig. 10-25A and B). In some cases, however, a strikingly abnormal EEG with multifocal spikes may be found in neurologically normal children with normal EEG background activity such as patients with BECTS and/or benign occipital epilepsy of childhood (see Fig. 10-4 and 10-5).52
Experimental animal studies clearly established that a seizure focus created by alumina cream or cobalt injection in the cortex results in the development of a secondary epileptogenic focus at the homotopic area of the contralateral hemisphere.53 At first, spikes from the secondary focus (mirror focus) are dependent on the primary focus and disappear after the excision of the primary focus or after a corpus callosum resection. However, in time, spikes from the mirror focus become progressively more independent from the primary focus and can no longer be abolished with the resection of the primary focus. The existence of a mirror focus in human epilepsy has long been debated, but substantial evidence indicates that secondary epileptogenic foci exist in humans. For example, it is not uncommon to find bilateral independent interictal spike foci in both temporal lobes of patients who are evaluated for intractable seizure with
implanted electrodes, even if ictal discharges arise only from one temporal lobe. Also, the patients with intractable epilepsy secondary to brain tumors occasionally showed additional independent spike foci in the hemisphere opposite the side of the lesion.54
implanted electrodes, even if ictal discharges arise only from one temporal lobe. Also, the patients with intractable epilepsy secondary to brain tumors occasionally showed additional independent spike foci in the hemisphere opposite the side of the lesion.54
MIDLINE SPIKES. Midline spikes appearing maximally at Fz, Cz, or Pz electrodes must be differentiated from physiologic K complexes or vertex sharp waves of sleep. A “spiky” waveform morphology or appearance during the awake state may assist in distinguishing between them. A Pz spike is relatively easy to differentiate from a vertex sharp wave (Fig. 10-13), but the differentiation is often difficult for Cz spikes (Fig. 10-14). These midline spikes are most likely a very localized expression of bilaterally symmetric, parasagittal dominant spikes or spike-wave discharges. Therefore, some patients have localized spikes at the midline as well as generalized and symmetric spikes or spike-wave discharges. Most of these patients, especially children, have generalized tonic-clonic seizures.6 Midline spikes, especially in an adult, may correlate with structural lesions of the parasagittal regions or in the deeper mesial surface.55
GENERALIZED EPILEPSIES AND SYNDROMES
Idiopathic generalized epilepsies and syndromes include absence seizures (childhood and juvenile) and juvenile myoclonic epilepsy (JME). West syndrome and Lennox-Gastaut syndrome (LGS) are examples of generalized epilepsy of a symptomatic type.
Generalized epileptiform discharges appear in both hemispheres simultaneously with similar configuration, symmetric amplitude, and synchronous timing between homologous electrodes. Timing between anterior and posterior discharges may differ slightly within the same hemisphere. When discharges have consistently higher amplitudes in one hemisphere or generalized discharges are consistently preceded by focal discharges, this may suggest a focal onset to a secondarily generalized seizure type, but clear-cut differentiation between a primary and secondary generalized seizure pattern is not always an easy task. Distinction between ictal and interictal patterns is also not as clear as that of a focal seizure. Often, the EEG associated with a clinical seizure may simply be a longer and more rhythmic repetition than interictal IEDs.
Absence Seizures
The discovery of 3-Hz spike-wave bursts associated with petit mal or absence seizures by Gibbs et al.54 in 1935 was the first major epoch in the history of EEG and for the electrographic diagnosis of epilepsy. This is characterized by rhythmic cycles of spike and wave complexes at a frequency of about 3 Hz, usually lasting a few to several seconds. The spike-wave complexes are generally maximum in the frontal region and may start with 3 to 3.5 Hz and end with 2 to 3 Hz (Fig. 10-15; see also Figs. 6-6A and 9-3, Video 9-2). The initial complex may have a polyspike-wave pattern. Although the bursts seem to be synchronous between the two hemispheres, detailed analysis with a faster sweep recording shows that the spikes in one hemisphere may randomly precede those in the other hemisphere
by a few milliseconds.55 The discharges tend to be inhibited by eye opening or increased vigilance. Hyperventilation can often precipitate the bursts associated with clinical absence seizures in 50% to 80% of patients56,57,58 (see Video 9-2). Intermittent photic stimulation induces spike-wave bursts in about one fifth of the patients with absence epilepsy.59 NREM sleep also increases the number of spike-wave complexes, which tend to become more irregular with polyspike-wave patterns.60 REM sleep decreases the number of bursts to a frequency slightly less than the waking state.60 Valproic acid and ethosuximide, commonly used medications for absence seizures, tend to decrease the number of spike-wave bursts61 and also attenuate activation by photic stimulation.62
by a few milliseconds.55 The discharges tend to be inhibited by eye opening or increased vigilance. Hyperventilation can often precipitate the bursts associated with clinical absence seizures in 50% to 80% of patients56,57,58 (see Video 9-2). Intermittent photic stimulation induces spike-wave bursts in about one fifth of the patients with absence epilepsy.59 NREM sleep also increases the number of spike-wave complexes, which tend to become more irregular with polyspike-wave patterns.60 REM sleep decreases the number of bursts to a frequency slightly less than the waking state.60 Valproic acid and ethosuximide, commonly used medications for absence seizures, tend to decrease the number of spike-wave bursts61 and also attenuate activation by photic stimulation.62
In patients with absence epilepsy, background activity is usually normal, but slowing can occur in a minority of patients. Generally, about 20% to 40% of patients with absence seizures show 3-Hz rhythmic delta bursts in the occipital regions (OIRDA, occipital intermittent rhythmic delta activity) (see Fig. 8-15; see also Video 10-6), and the incidence of OIRDA is much higher in children between 6 and 10 years old.63 Visible clinical seizures can usually be observed when spike-wave bursts last more than 4 to 5 seconds. Symptoms are characterized by staring with impaired responsiveness and behavior arrest. Impaired responsiveness can occur with spike-wave bursts as short as 3 seconds. Responsiveness returns abruptly to normal at the end of the spike wave. Detailed psychophysiological testing of such patients found decreased reaction time even during a brief spike-wave burst without an associated overt clinical seizure.64 Automatisms with lip smacking, chewing, fumbling, or mild myoclonus (eyelid twitches) are common.65 This may make it difficult to differentiate from temporal lobe seizures clinically. Some patients may have decreased postural tone. It is a technologist’s role to examine the presence or absence of impaired responsiveness and to note the patient’s behavior during spike-wave bursts. Absence seizures are classified into two types by age of onset.
CHILDHOOD ABSENCE EPILEPSY. Onset of seizures is from 3 to 12 years. EEG and clinical presentation can assist in predicting the prognosis. Patients with an EEG showing 3-Hz OIRDA have a smaller risk of developing tonic-clonic seizures in the future.66 Absence seizures without myoclonus have a higher chance of remission than those with myoclonus.58
JUVENILE ABSENCE EPILEPSY (JAE). The onset of this type of epilepsy is around 9 to 13 years or even later. The spike-wave bursts tend to start with slightly faster frequency than 3 Hz and may have polyspike components (Fig. 10-16; Video 10-6). This group of patients is more likely to develop JME (see Fig. 10-21A and B).59
EYELID MYOCLONIA WITH ABSENCE (JEAVONS SYNDROME). Repetitive blinking is a common symptom during childhood absence seizures, but eyelid myoclonia typically occurs immediately after eye closure associated with brief generalized spike-wave bursts of 4 to 6 Hz. Photoparoxysmal discharges are common, inducing repetitive
eyes blinking (Video 10-7). The same patient may also have typical absence seizure with 3-Hz spike-wave bursts.67
eyes blinking (Video 10-7). The same patient may also have typical absence seizure with 3-Hz spike-wave bursts.67
Generalized Tonic-Clonic Seizures
Multiple spike-wave (polyspike-wave) bursts lasting less than one to several seconds are usually an interictal expression of a generalized seizure. The interictal EEG of idiopathic (primary) generalized tonic-clonic seizures consists of a variety of waveforms that are more irregular and of faster frequency than the 3-Hz rhythmic spike-wave discharges seen in absence seizures (Fig. 10-17A). In addition, spike-wave bursts often include multiple spikes (polyspikes) (Fig. 10-17B). Spike-wave bursts are usually, but not always, symmetric and synchronous. Asymmetric bursts do not necessarily exclude the possibility of primary generalized epilepsy. In fact, it is often difficult to differentiate primary from secondary generalized epilepsy, especially in the case of frontal lobe epilepsy. In some patients, focal spike discharges, such as “Rolandic spikes,” may coexist with generalized spike-wave bursts. In such cases, it is not possible to determine if the patient has a seizure of focal onset with secondary generalization or has both partial and primary generalized epilepsies. There are two features that may help to point toward a diagnosis of primary generalized epilepsy. One is a photoparoxysmal response (Fig. 10-18A and B), and the other is generalized spike-wave bursts resembling K complexes (Fig. 10-19). Like K complexes, spike-wave bursts act as an arousal pattern and the patients may wake up or open their eyes coincident with the bursts. In some, generalized spikewave bursts may be precipitated by arousal stimuli, similar to the K complex. In some patients, especially in children who tend to have “spiky” K complexes, it may be difficult to differentiate between the two. These features were studied in detail by Niedermeyer, who introduced the concept of “dyshormia,” in which primary generalized spike-wave bursts and K complexes share the same generating mechanism, producing generalized burst activity.68
Onset of the ictal event in a generalized seizure consists of low-voltage rhythmic beta-range fast activity, with progressively increasing amplitude and decreasing frequency (Fig. 10-20A and B). This is followed by generalized spike-wave bursts, which become progressively slower in frequency and less rhythmic toward the end of the seizure. The EEG becomes suppressed during the immediate postictal period and is then followed by the appearance of postictal delta activity. Clinically, the initial fast activity corresponds to the tonic phase and the subsequent spikewave bursts coincide with the clonic phase of the seizure. During the ictal events, both at onset and during the progress of the seizure, EEG activities are largely obscured by muscle and movement artifacts, making it difficult to recognize the EEG changes and to differentiate genuine seizure from pseudoseizure. The presence of postictal flattening or slowing provides evidence of genuine seizure (Video 10-8). Conversely, normal EEG immediately after the event favors pseudoseizure, especially if the patient remains unconscious or confused.
Juvenile Myoclonic Epilepsy
This was originally described by Janz as “impulsive petit mal”69 and may be referred to as “juvenile myoclonic epilepsy of Janz.” JME is the most common type of seizure among idiopathic generalized epilepsies. Close to half of the patients have a family history of epilepsy.69 As the name implies, the seizures usually begin in adolescence. But many patients start with absence seizures at a younger age. The majority of patients (>90%) also have generalized tonic-clonic convulsions. Both myoclonic and generalized tonic-clonic convulsions tend to occur within 1 to 2 hours after awakening. A history of absence seizures coexists with or precedes myoclonic seizures in about one third of patients.70
Interictal EEG patterns consist of generalized polyspike and polyspike-wave discharges with frontocentral predominance (Fig. 10-21A). These patterns are not distinguishable from other idiopathic generalized epilepsies but may include more polyspike components. Like other idiopathic seizures, background activity is usually normal. Spike-wave or polyspikewave bursts are usually faster than the typical 3-Hz spike waves seen in absence seizures, but in some patients, 2.5- to 3-Hz spike-wave bursts may occur that are indistinguishable from typical absence seizures (Fig. 10-21B).
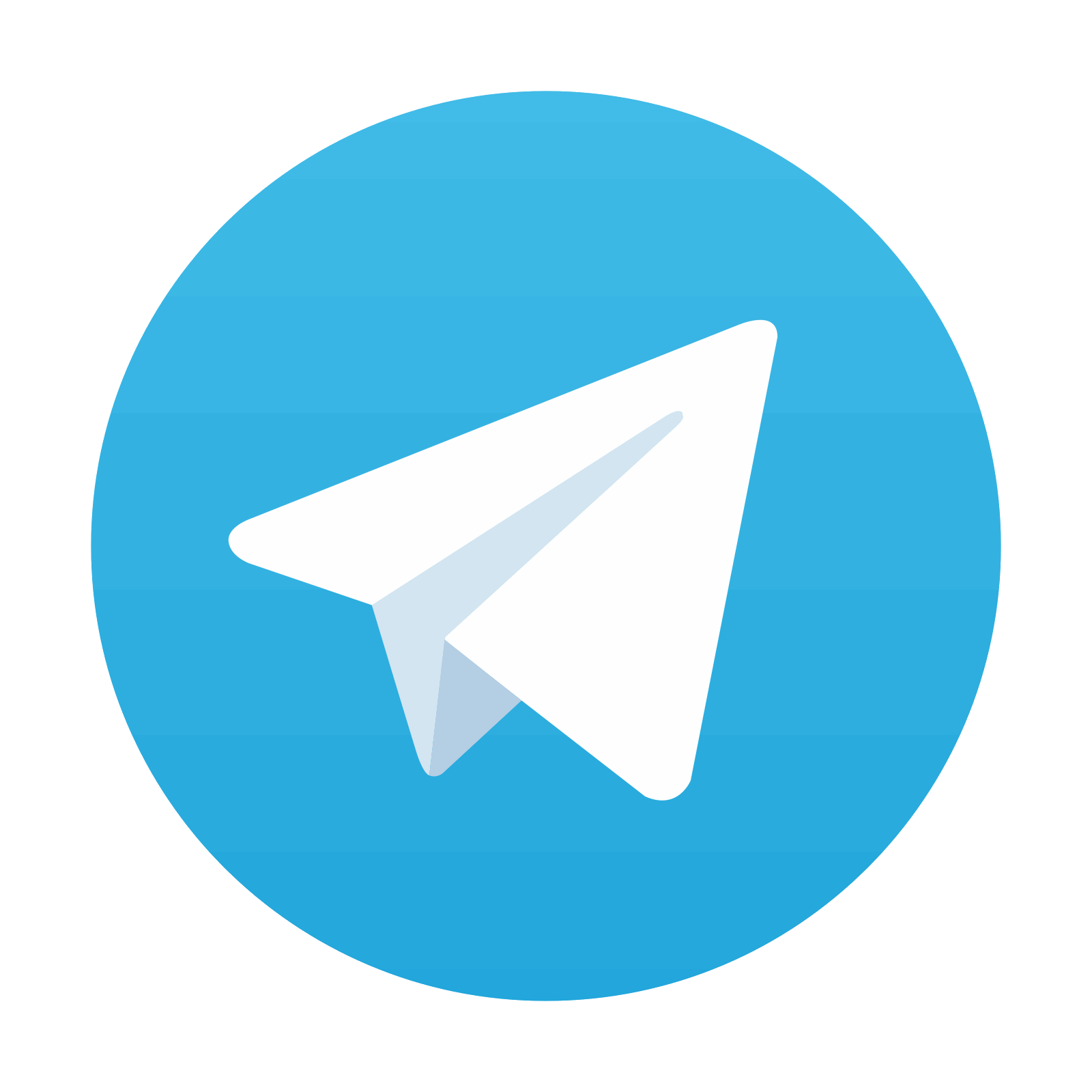
Stay updated, free articles. Join our Telegram channel

Full access? Get Clinical Tree
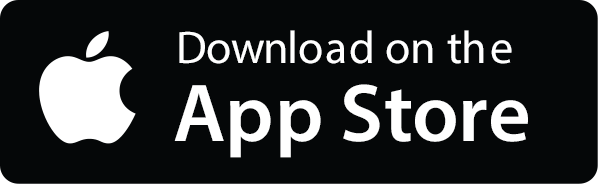
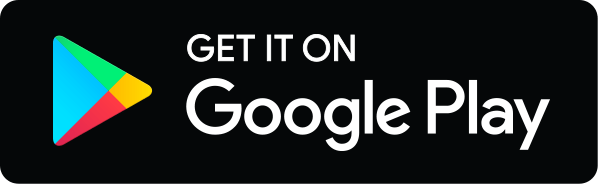
