Fig. 15.1
Explanation of the 2 × 10 task. In this task, 2 out of 16 (4 × 4 matrix) LED buttons (set) were illuminated simultaneously and participants had to press these buttons in a set in the correct predetermined order. Ten sets were presented in a fixed order (hyperset). Participants had to learn the correct order by trial and error. When the participants pressed a button on the wrong order, the trial was aborted. The participants then had to restart the trial from the home key. The same hyperset was repeated until the participants completed it successfully for 10 trials in the current experiment
Note that due to its trial-and-error nature, the errors in the initial learning stage included both inevitable errors (errors made during the exploration of the correct sequence by trial and error in trial 1) and genuine errors (errors made in trials 2–10, after the correct sequence was identified in trial 1). Errors made in the later elaboration stage included only genuine errors, because the sequence had already been completed once. In the usual 2 × 10 task, the probability of inevitable errors is 0.5 when participants first encounter novel sets. In order to produce a “forced error” and a “forced success” group in our experiment [10], we made the probability of inevitable errors in the initial learning stage 1.0 for some participants (i.e., they always chose the button that was wrong for the first time; forced error condition) and zero for the other participants (i.e., they always chose the button that was correct; forced success condition). If the experience of unsuccessful trials has any beneficial effect on the elaboration of the learned sequence, the number of genuine errors in the elaboration stage should be lower in the forced error condition than the forced success condition. However, the results of the ratio of genuine error trials per successful trials showed the opposite pattern. The forced error condition led to worse performance in the elaboration stage than the forced success condition (Fig. 15.2a). We also evaluated performance speed by measuring the time that elapsed for each successful trial (time between the moment the home key was pressed to the moment the second button of the final (10th) set was pressed; Fig. 15.2b). While the performance speed in the last successful trial of the initial learning stage was worse in the forced success condition relative to the forced error condition, performance in the subsequent trials (i.e., the elaboration stage) was not different between the conditions. These results indicated that experiencing errors in the initial learning stage of the 2 × 10 task did not have any beneficial effects on the later elaboration stage in terms of accuracy or speed of performance. In other words, in the trial-and-error learning in the 2 × 10 task, “trial” is an important factor but experiencing “errors” is not. Although it may be premature to extend our findings in the visuomotor sequence learning task (i.e., 2 × 10 task) to other learning paradigms, the present observations support the importance of successful, rather than unsuccessful, experiences in learning.
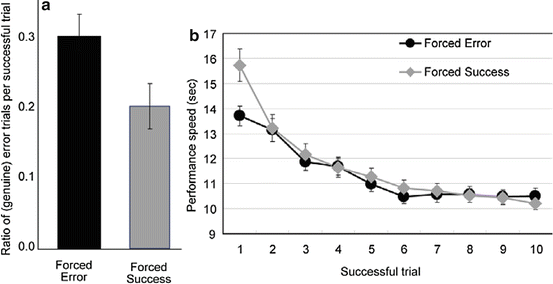
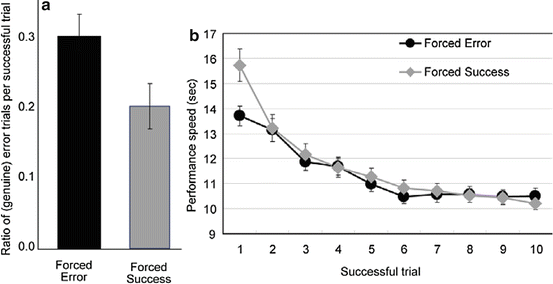
Fig. 15.2
Experiencing errors in the initial learning stage of the 2 × 10 task did not make the accuracy and speed of performance better in the later elaboration stage. Error bars indicate the standard error of the mean. N=22
15.3 Social Rewards Enhance Offline Improvements in Motor Skill
Positive evaluation by others (i.e., praise) has been known to enhance self-efficacy [11, 12], feelings of competence and autonomy [13], positive feelings [14], the association made between responses and their positive outcomes [15], and incentives for task engagement. Specifically, praise is thought to improve the level of subjective competence [16] by acting as an incentive to boost practice efforts [17]. Thus, praise equals enhanced motivation, which accelerates motor skill performance [16, 18, 19]. Indeed, because perseverance is needed for acquiring motor skills, repetitive practice of a given action is needed to master the motor skill, and successful experience and positive feedback become potential factors in enhanced motor learning.
Skills are improved not only online during practice but also by offline consolidation. Offline consolidation is a critical stage of successful learning, during which the newly acquired skill is reinforced. In learning a motor skill, consolidation is an opportunity for that learning process to evolve further despite the end of practice [20–22]. Indeed, consolidation is critical for both skill formation and long-term retention [23–25].
Surprisingly, few studies have investigated the effects of praise on skill consolidation. In a recent study [26], we examined whether praise influences skill consolidation directly or whether it acts to increase motivation for continued practice (an indirect effect). Participants were praised only after training was completed. Then they were allowed a 24 h retention period, after which participants were given a “surprise” retest of the trained sequence.
Initially, all participants were trained on a sequential finger-tapping task [21, 24, 25, 27–31]. They were told that they were monitored via webcam by evaluators in another room whose comments would be filmed following training. However, their performance was actually unmonitored, and without their knowledge, the movies’ contents were predetermined and prerecorded. Participants were divided into three groups with different praise-related characteristics (Fig. 15.3): (1) Self group participants watched a movie in which they were praised for their training performance; (2) Other group participants watched the same movie as the Self group, but were told that it was another participant’s performance evaluation; and (3) No-praise group participants saw no movie and received no other praise. At the end of day 1 of the experiment, participants were told that they would be performing a different task the next day (24 h later). However, all participants were instead given a “surprise” retest of the trained sequence in order to minimize the possibility of physical or mental practice prior to retesting. This also served to reduce the likelihood that Self group (or potentially Other group) participants would be more motivated to perform the next day’s tasks. We examined the effect of manipulating praise on retest performance by having participants perform (1) a novel sequence; (2) a randomly ordered tapping task; and (3) a working memory task, after the retest was finished. These additional tasks were included to differentiate between the effects of praise potentially related to an increase in motivation to perform well, as opposed to its effects on offline processing (consolidation). If praise enhanced general motivation, performance on the additional tasks on day 2 should be better only in the Self group, and not in the Other and No-praise groups.
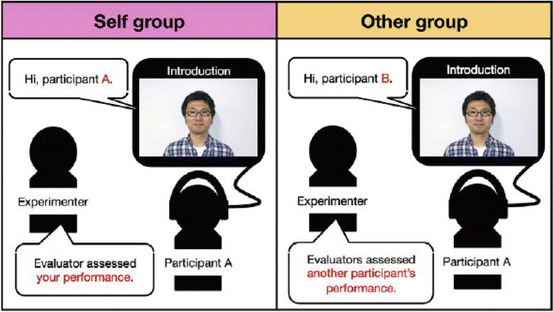
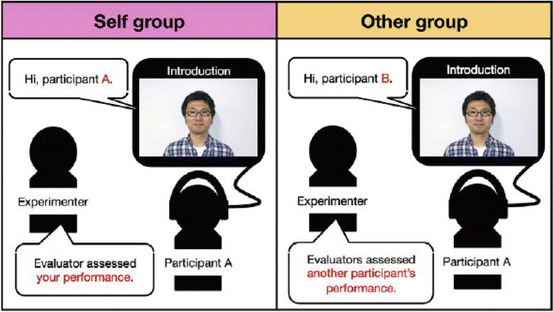
Fig. 15.3
Social reward improved consolidation (experimental paradigm). Self group participants were told that they were watching their own performance evaluation, while participants in the Other group were told they were watching another participant’s performance evaluation. Reproduced from Sugawara et al. [26] with permission
The results supported rather than refuted our hypotheses. Performance improved significantly in all groups between the end of training on day 1 and the retest on day 2, confirming consolidation of the trained sequence [24, 25]. However, the degree of offline improvement was significantly different, such that improvement was significantly greater in the Self group than in the Other group and the No-praise group (Fig. 15.4). Thus, praise appeared to have a positive influence on consolidation of the trained sequence. Additionally, Self group participants rated the movies as being significantly more pleasant than Other group participants, confirming the successful manipulation of praise. No significant group differences were noted in non-trained sequence, randomly ordered tapping task, or working memory task performance, suggesting that motivation was not generally affected by praise. These results instead demonstrate that praise enhanced skill consolidation directly.
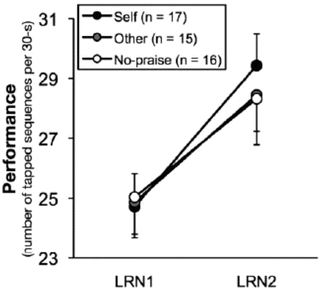
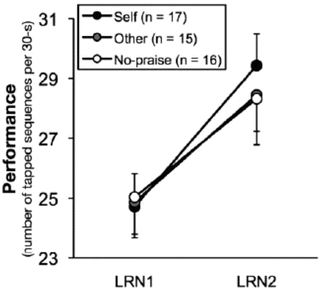
Fig. 15.4
Social reward improved consolidation (Results). Comparison of the mean performance on the last 3 training trials on day 1 (LRN1) and the first 3 retest trials on day 2 (LRN2). All groups improved on the trained sequence, confirming task consolidation had occurred. Notably, the offline performance enhancement in the Self group was significantly greater than in the Other and No-praise groups from LRN1 to LRN2. Error bars indicate the standard error of the mean. Reproduced from Sugawara et al. [26] with permission
Praise can be classified as a reward [32] and as such can induce feelings of happiness (hedonic component) and promote motivation [16, 18, 19], two key components of reward [33]. A recent human neuroimaging study demonstrated that praise activates the reward-related ventral striatum [32]. Corticostriatal circuits are critical for the automation of the motor sequence learning used in the present study [34–36] through dopaminergic reward circuits [37]. Thus, rewards could affect motor skill consolidation through alteration of reward-associated dopamine neurotransmission in the ventral striatum [38]. Taken together, these data imply that praise acts as a “social reward” that induces striatal dopamine transmission and enhances motor skill consolidation. Further understanding of the effects of social rewards on skill consolidation could produce techniques to improve motor skill learning in educational and rehabilitative contexts.
15.4 Transcranial Direct Current Stimulation and Behavioral Change in Clinical Fields
Because neural activity, which produces our mental function (and behavioral output), is dependent on electrical activity, tDCS could modulate activity and behavior. Indeed, tDCS can evoke transient changes in neural activity even with currents below 1 mA [39–45]. One mechanism for this has been suggested, where one study found an increase in cortical excitability following tDCS [43]. Subsequent extensive and methodical supporting studies have brought renewed attention to the neural effects of tDCS [41].
A potentially useful characteristic of tDCS, one that enables investigation of causal relationships between brain activity and behavior, is its ability to increase (anodal tDCS) or decrease (cathodal tDCS) the excitability of a stimulated cortical region depending on the polarity used for the stimulation. Hand motor cortex excitability, for example, is transiently increased by anodal tDCS and decreased by cathodal tDCS [41, 46, 47]. In addition, anodal tDCS of motor cortex enhanced the motor-evoked potentials (MEP) induced by transcranial magnetic stimulation (TMS), whereas cathodal tDCS suppressed them [41, 47]. Although the mechanisms by which tDCS affects neural activity in human motor cortex are still unknown, they appear to involve altering the membrane potential of groups of neurons, unlike the neuronal spike-inducing effect of TMS.
The tDCS device is relatively small and elicits no acoustic noise or muscle twitching, unlike other brain stimulation techniques, and is thus ideally suited for double-blind sham-controlled and/or clinical studies [40, 48, 49]. Furthermore, tDCS-induced changes in excitability have been associated with changes in the cognitive and motor task performance [42, 45, 50, 51], suggesting a high potential (even therapeutic) for utilizing tDCS over a wide range of applications.
Anodal tDCS has been shown to enhance motor functions such that application to the hand motor cortex was associated with a temporary improvement in the performance of complex hand motor tasks related to daily living (Jebsen–Taylor hand function test) [52]. We examined whether anodal tDCS applied to the leg motor cortex could facilitate the performance of leg motor tasks involving pinch force (of the great toe and digitus secundus) and reaction time [53]. Participants performed the tasks before, during, and after anodal, cathodal, or sham tDCS over the leg motor cortex. Maximal leg pinch force, but not reaction time, was boosted by anodal tDCS in a transient manner. Cathodal and sham stimulation had no effect on performance, nor did any interventions intended to affect hand pinch force or reaction time. This implies spatial specificity of tDCS. Other studies have also reported that tDCS influences motor learning [54, 55]. For example, anodal tDCS of the motor cortex, but not the premotor or frontal cortex, enhanced sequential motor learning [56]. In another study, anodal tDCS improved the early stage of learning in a visuomotor tracking task [57].
Several studies have investigated the therapeutic effects of tDCS on motor dysfunction following stroke. Maximal pinch force increased in stroke patients after anodal tDCS of the hand motor cortex, as did performance on the Jebsen–Taylor hand function test [52, 58, 59], and their reaction time for performing simple hand motor tasks was shortened [60, 61]. Our recent study of tDCS effects on leg motor function also supports the therapeutic potential of tDCS in neurorehabilitation [62]. In a double-blind, crossover, and sham-controlled experimental design, 8 patients with chronic subcortical stroke (4F/4M; mean age = 59.6 years) performed knee extensions using their hemiparetic leg before, during, and after anodal or sham tDCS of the lower limb motor cortex in the affected hemisphere. Grip force in the affected hand was also recorded. Patients receiving anodal tDCS demonstrated an increase of 13.2 % in the maximal knee extension force versus baseline and sham stimulation, which persisted for less than 30 min (Fig. 15.5). Maximal handgrip force did not change. Thus, anodal tDCS selectively but transiently enhanced knee extensor strength. Previous studies have reported a correlation between knee extension strength and lower limb function in patients with stroke [63], which along with our data suggests that cortical stimulation may promote neurorehabilitation of lower limb dysfunctions in stroke patients. To test this hypothesis, future studies will need to assess if there is an association between improvement of knee extension force by anodal tDCS and enhancement in lower limb functional recovery following stroke. Another recent study found that anodal tDCS enhanced lower limb functions after hemiparetic stroke, and most interestingly, the effect was especially strong regarding fine control of ankle movement [64]. At the same time, our study was the first to demonstrate that anodal tDCS enhanced force production of knee extension in subcortical stroke patients using a sham-controlled, double-blind, and crossover design. Combined, Madhavan’s [64] and our findings provide evidence supporting the use of tDCS therapeutically to enhance recovery of lower limb functions after chronic subcortical stroke. The possibility exists that repeated applications of tDCS combined with rehabilitation training may even improve mobility [65, 66], as has been found examining upper extremity motor training [67].
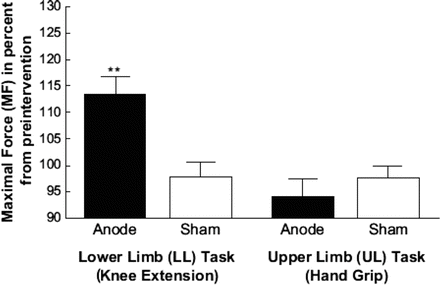
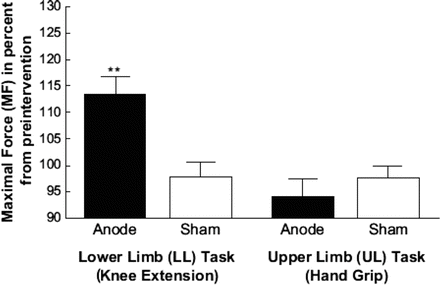
Fig. 15.5
Enhancement of motor function in stroke patients. Anodal transcranial direct current stimulation (tDCS) significantly affected the maximal force (MF) only in the lower limb (LL) task. There were no significant changes in the other three conditions. The black bars represent the results from the anodal condition, and the white bars represent the data from the sham condition. Data were normalized with respect to the baseline values prior to intervention. Data are presented as the mean maximal force (MF; n = 8) with error bars representing the standard error (SE). Reproduced with modification from Tanaka et al. [62] with permission
The effect of tDCS on Parkinsonian motor dysfunction [68] as well as in cases of focal dystonia has also been shown. Notably, Monti et al. reported that cathodal tDCS of Wernicke’s area, as opposed to anodal tDCS, enhanced the performance of aphasic patients in a picture-naming task [69]. In animal models, tDCS appears to influence motivation and reward systems [70] and thus has been suggested to alter preference and/or craving behaviors. Bilateral frontal cortical tDCS altered preference in patients with addictive disorders and reduce cravings for alcohol [71], smoking [72], and even for specific foods [73]. Indeed, the overall picture suggests that tDCS is potentially a therapeutic intervention for a wide range of neuronal dysfunctions, not to mention being a potentially useful neurorehabilitation tool.
15.5 Conclusion
In this chapter, the effects of positive feedback and successful experiences on behavioral change are discussed from the perspective of cognitive neuroscience. Three examples highlighted the effects of positive experiences on human performance: (a) in the trial-and-error learning in the 2 × 10 task, experiencing success enhances performance, whereas experiencing failure does not [10]; (b) social rewards directly enhance skill consolidation in humans and they might have a novel functional effect on the human motor memory system [26]; and (c) the effects of anodal tDCS on facilitating motor and cognitive functions may have great potential for cognitive and motor enhancement, supporting learning in healthy volunteers and speeding up rehabilitation in neurological patients [51, 62], for examples. Although it is still premature to conclude that the effects of positive feedback and successful experience can be applied to other forms of human cognition and performance, evidence has been accumulating that supports the unique effects of “happiness” in various contexts [1, 2]. Further research into the science of happiness is certainly warranted, which in turn will provide insight into our understanding of neuroplasticity and behavioral changes.
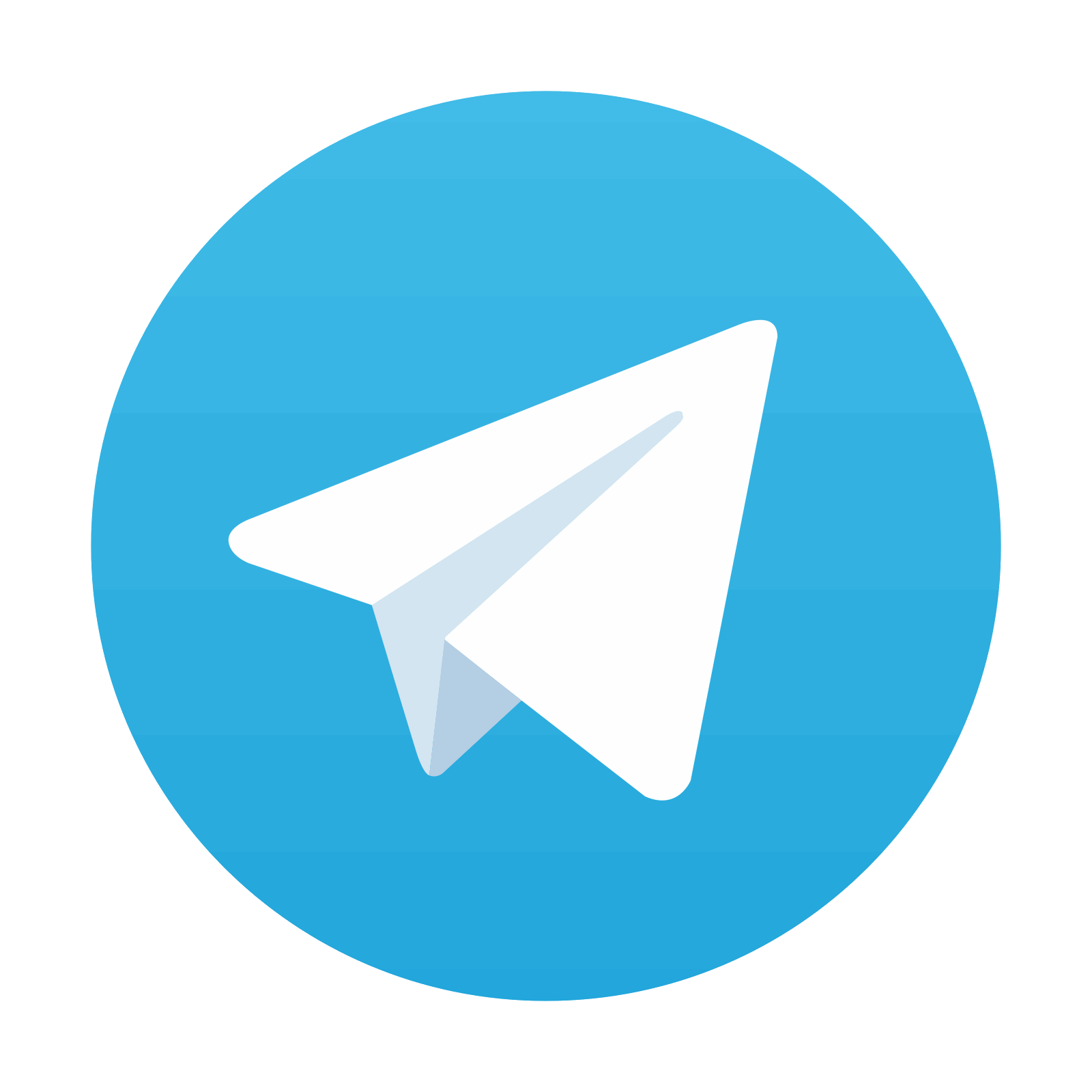
Stay updated, free articles. Join our Telegram channel

Full access? Get Clinical Tree
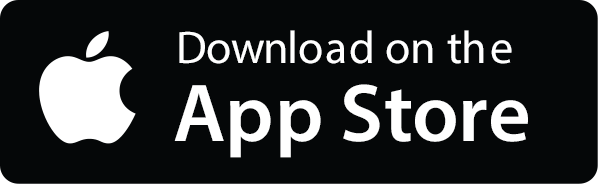
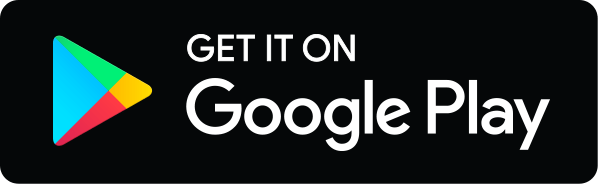