Chapter 18 Functional electrical stimulation
INTRODUCTION
Strengthening through electrical stimulation is sometimes called therapeutic electrical stimulation (TES). For added confusion, the abbreviation ‘TES’ has also been used for threshold electrical stimulation, in which extended periods (e.g. night-time) of very low-level intensity stimulation have been used to improve motor skills. The results from such stimulation are mixed (Dali et al 2002) and this technique will not be discussed further. The terms ‘neuromuscular electrical stimulation’ (NMES) and «functional neuromuscular stimulation’ (FNS) are also used in the literature to cover direct and indirect functional applications of electrical stimulation.
In most reported applications of FES, electrical impulses are applied to innervated muscles such that impulses result in nerve depolarization and then subsequent muscle contraction. The basic theory behind this has already been reviewed in Chapters 13–15, but typical stimulation parameters for FES are pulse widths of 100–1000μs and frequencies of 10–100Hz. The amplitude varies with the application and the impedance characteristics of the patient; however, for surface stimulation, values of up to 120mA (assuming a 1kω load) are not uncommon. In some cases, electrical impulses have been used to recruit denervated muscle by direct depolarization of muscle fibres. However, the electrical impulses have a pulse width that is usually 100–1000 times greater than those used for innervated muscle stimulation, so necessitating the development of a different range of stimulation equipment. Nonetheless, work in this area has the potential to restore function, and some success has been reported, e.g. maintenance of standing posture following spinal cord injury (SCI) (Kern et al 2005). Although this is a very relevant and interesting area of work, for the remainder of this chapter, innervated muscle will be assumed.
Skin-surface electrodes are often used in pairs, such that one (called the active or negative) electrode is usually placed over the motor point and the other (indifferent) electrode is placed at the proximal or distal end to complete the circuit and minimize recruitment of other muscles (Fig. 18.1). In some cases, rather than direct stimulation of the motor nerve, stimulation may be used to target a reflex action. The classic example is that of stimulation of the common peroneal nerve withdrawal reflex, which, if successful, can lead to a combination of hip and knee flexion, and ankle dorsiflexion (Fig. 18.2). It should not be forgotten that surface stimulation has a sensory component that, in some patients, can lead to disuse and make stimulation of less superficial muscles problematic. In addition, in some cases, particularly with finger/hand stimulation, it can be difficult to achieve or maintain the necessary specificity for clinical use. Percutaneous and implanted systems can address some of these problems, but the benefits have to be balanced against cost and clinical convenience.
In the following sections, a number of common applications of FES for upper and lower limb applications will be discussed and practical examples presented, however, it is a dynamic field and the review is indicative only. The reader is also encouraged to review other areas, for example restoration of bladder and bowel function, phrenic nerve stimulation, the use of stimulation to encourage fibre type conversion, and the general area of neuromodulation. Further recommended reading and information sources are given at the end of the chapter. For specific guidance on surface electrode positions the work by Baker et al (2000) and the information available from Odstock Medical Ltd (Salisbury District Hospital, Salisbury, UK) will be of particular interest.
LOWER LIMB STIMULATION
For some time, there has been considerable interest in the application of FES following SCI. Much of the earlier work used surface systems, and benefits in muscle bulk and blood flow through regular use of surface electrical stimulation have been reported (Taylor et al 1993). The use of surface systems to provide standing and walking has also been investigated (Fig. 18.3). The type of work reported ranges from stimulation-only-based systems (Ewins et al 1988) to hybrid approaches in which FES and mechanical (orthotic) systems are combined, for example Davis et al (1999) combined stimulation with a specially designed ankle–foot orthosis (AFO), and Sykes et al (1996) evaluated the benefits of combining FES with a reciprocating gait orthosis (RGO). The benefits of stimulation only systems are the lack of encumbrance of external mechanical aids and therefore reliance on and use of the subject’s own musculature. The downside includes fatigue, resulting from synchronous recruitment of motor units (with a potentially greater proportion of type II muscle fibre following SCI), which can lead to short standing durations, and the issues surrounding control of a complex biomechanical system with a limited number of stimulation channels.
Routine clinical take-up of surface systems (standalone and otherwise) has been limited because of a combination of factors, including system complexity, cosmesis, time to attach/remove components and relative functional gain provided when compared to, for example, advances in wheelchair technology. Some of these problems have been addressed through the development of percutaneous and implanted stimulators, but further work in, for example, implantable sensor technology and system modelling, is required before routine daily use becomes practicable. This has led to a refocusing of interest in the use of FES for SCI, certainly in the UK, with growing emphasis on areas such as FES to assist fitness through its application in cycling (Fig. 18.4) and rowing (Hunt et al 2006, Wheeler et al 2002). In such systems, stimulation of large muscles groups, e.g. quadriceps and hamstring muscles, have the potential to provide overall performance and health benefits, doing so as part of regular recreational activities, which should encourage transfer of the technology to more routine use.
One of the earliest published examples of FES was as a gait assist for patients who present with a flaccid foot drop (Liberson et al 1961). In the simplest case, the FES system consists of a single-channel device stimulating the peroneal nerve to induce ankle dorisflexion and hip and knee flexion during the swing phase of the gait cycle. A sensible starting point for the electrode positions would be as shown in Figure 18.2; however, a number of electrode positions may have to be evaluated before an optimal response is found. The system employs a sensor that controls the timing of the stimulation. This is often a footswitch placed under the heel. In most cases, stimulation starts once the heel of the affected leg rises off the ground and ends when the heel makes contact with the ground during the swing to stance transition. The stimulator is usually worn either in a pocket or attached to a belt. Figure 18.5 shows a modern version of the stimulation equipment. Other physical sensors, e.g. tilt sensors, goniometers and electromyographic signals, have been investigated with varying levels of success, although the tilt sensor based WalkAide system (Innovative Neurotronics, Maryland, USA) is now marketed in the USA.
The most commonly cited patient groups for dropped-foot stimulation are stroke and multiple sclerosis. For example, Taylor et al (1999a) reported a significant increase in walking speed and decreased energy consumption in a stroke patient group, and reported similar orthotic benefits in patients with multiple sclerosis. They also found smaller but still significant ‘carry-over’ benefits when the stimulation was not used. Kottink et al (2004) concluded that their review suggested a positive orthotic effect of FES on walking speed following stroke.
The authors’ experience is that a very wide range of responses is shown to stimulation in stroke and MS patient groups, from quite dramatic improvements (Fig. 18.6) to no visible change. Despite a lack of observable improvement, some patients in the latter group report the FES to be helpful in assisting walking. This could be as a result of the sensory cue delivered by stimulation. Some preliminary work in patients with Parkinson’s disease (Mann et al 2004) supports this observation.
When reviewing the evidence for the application of FES in improving motor control, the Royal College of Physicians’ (2004) National Clinical Guidelines for Stroke concludes that ‘FES should not be used on a routine basis and that individual patients should be considered for FES as an orthosis in certain circumstances, such as improving arm movement, ankle dorsiflexion and gait performance.’ The guidelines go onto say that ‘when considering the use of FES as an orthosis, local teams may wish to specify which patients are considered suitable; and how its benefit is to be judged for any patient trying it.’ The need for further research in this patient group was also identified in the review by Pomeroy et al (2006), who found that questions such as the most effective type of stimulation, dose and time after stroke require further investigation.
Surface FES has also been investigated to assist in the gait of children with cerebral palsy (CP). One example is for those children who present with an equinus gait. There are two apparently opposing approaches, with some studies reporting positive results from stimulating the anterior tibial muscles (Durham et al 2004, Hazlewood et al 1994, Pape et al 1993), whereas others support stimulation of the calf or triceps surae muscle group. The rationale for electrical stimulation to the anterior tibial muscle is to produce active dorsiflexion at the ankle during the swing phase of gait, which at the same time reciprocally inhibits the antagonist, triceps surae (Hazlewood et al 1994). By contrast, stimulating the triceps surae is thought to favourably modify altered muscle activation patterns with a reduction in spasticity (Rose 1998). In a study of four children (Carmick 1993, 1995), gait was improved through the application of electrical stimulation to triceps surae. In this study there was good evidence of a carry-over effect with improvements remaining when stimulation was not used in two of the four children. In a further study (Comeaux et al 1997), electrical stimulation of the gastrocnemius alone and then the gastrocnemius and tibialis anterior resulted in a trend towards increased dorsiflexion at initial toe contact. It made no difference whether gastrocnemius alone was stimulated or gastrocnemius and tibialis anterior alternately; both produced significant and similar results. This improvement was small but followed only short periods of limited use. Whereas both approaches have shown some encouraging results, they fail to produce conclusive evidence as to which is the treatment method of choice, or to provide prescription guidelines for appropriate patient selection. With this uncertainty, and as indicated by the work of Postans and Granat (2005), use of formal assessment techniques such as gait analysis might lead to more effective use of FES by allowing the clinical team to develop a greater understanding of the underlying reasons for the presenting problem.
The use of electrical stimulation for the treatment of congenital talipes equinovarus (CTEV) was suggested by Kirsch and Pape (1992). Their treatment involved two children aged 7 and 11 and a 44-year-old patient who suffered from persistent residual problems of CTEV. The results showed an increase in the active range of motion of the ankle and foot, an increased muscle bulk of the thigh and calf, and an improvement in the number of steps per 6 m and the distance travelled in a 6-minute walk. They suggested that the outcome of CTEV may improve by including treatment of the muscle atrophy with electrical stimulation and that younger children might demonstrate a greater benefit. Studies into the effect of stimulation on children with CTEV are being conducted at Queen Mary’s Hospital, Roehampton, using surface electrodes following treatment by the Ponseti method. Figure 18.7 shows typical electrode positions used in this work to improve muscle strength of the weaker dorsiflexors and evertors. The preliminary results showed an improvement in foot position in both standing and walking and emphasized the need for further research in this area.
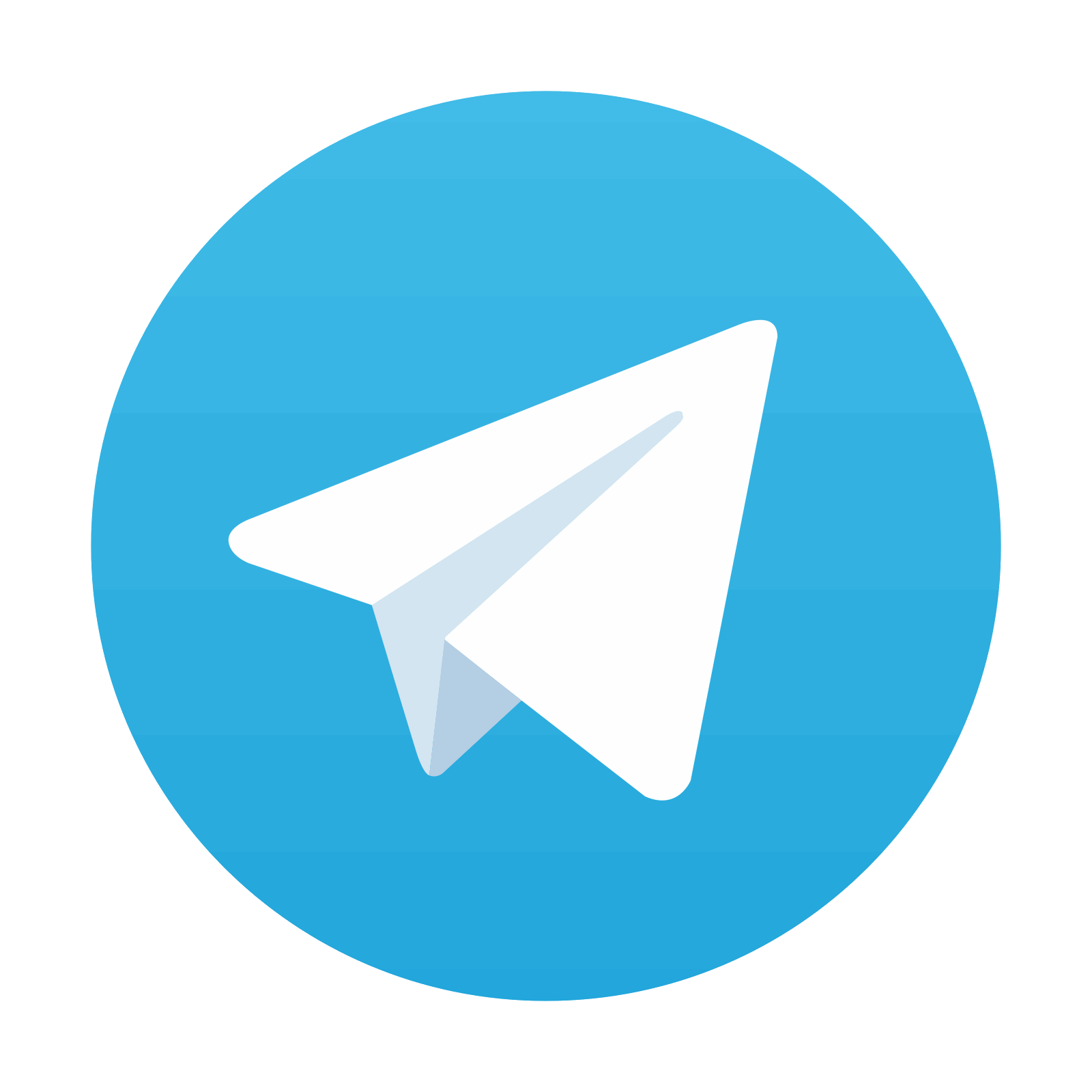
Stay updated, free articles. Join our Telegram channel

Full access? Get Clinical Tree
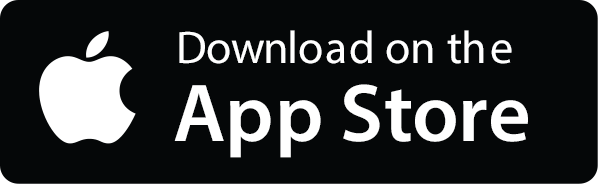
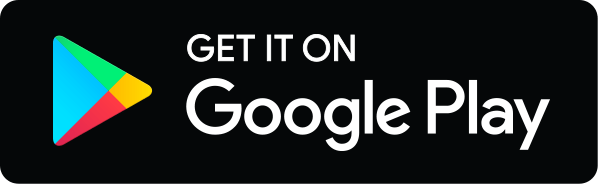