(1)
Neurological Department, Municipal Hospital Dessau, Dessau-Rosslau, Germany
5.1 Introduction
Electrodiagnostic examination (EDX) consists of two major parts: nerve conduction studies (NCS), including long latency reflex testing (F-waves), and needle electromyography (EMG). In addition, somatosensory evoked potentials and magnetic stimulation motor evoked potentials are needed sometimes, but the two latter techniques are outside the scope of this chapter. NCS and EMG are usually performed together. In contrast to nerve imaging such as magnetic resonance imaging (MRI) and high-resolution ultrasound (HRUS) (see Chap. 6), EDX provides functional information about the electrical properties of the peripheral nerves. EDX should only be carried out by specially trained staff, such as by a certified physician, or under his or her direct supervision [1].
What does EDX do? It extends the clinical examination. It can differentiate between disorders of the peripheral nerves, the neuromuscular junction, and the muscle itself. In a peripheral nerve disease in particular, EDX provides useful data regarding the localization of focal involvement (e.g., anterior horn cell, nerve root, nerve plexus, peripheral nerve), the type (e.g., demyelinating or axonal), the severity (incomplete versus complete), the duration (subacute or chronic), and the prognosis (reinnervation or no reinnervation) [2, 3].
What is EDX not able to do? It cannot replace a careful anamnesis survey and clinical examination by a neurologist experienced in the field of peripheral nerve disorders [4, 5]. Furthermore, EDX is unable to depict the underlying pathological features of a peripheral nerve disease. For instance, a carpal tunnel syndrome is usually caused by compression of the median nerve owing to narrowness within the carpal tunnel, but it may also occur because of compression by a mass lesion such as a ganglion cyst, an intraneural hematoma, or a peripheral nerve tumor. In all these cases, EDX provides the same result. Only imaging techniques (see Chap. 6) are able to demonstrate the underlying pathological features in those cases [6].
5.2 Types of Nerve Fibers Assessable with EDX
According to the classification of Erlanger and Gasser, there are three different types of nerve fibers: large myelinated A-alpha fibers, small myelinated A-delta fibers, and unmyelinated C fibers [7]. A very important fact is that conventional EDX can only assess the largest, fast-conducting, and well-myelinated A-alpha fibers of the motor, sensory, and “mixed” peripheral nerves, including efferent motor fibers and afferent sensory fibers mediating the sense of touch, position, and vibration. Slow conducting A-delta and C fibers mediating pain, cold, and warm sensations are only measureable with special techniques, such as heart rate variability test or sympathetic skin response test, which are discussed elsewhere [2, 3]. This fact explains why some painful conditions, such as small fiber neuropathy, cannot be examined using conventional EDX [4, 8].
5.3 Motor Nerve Conduction Studies
5.3.1 Technique and Parameters
In a motor NCS, the peripheral nerve is stimulated with a transcutaneous depolarizing square wave electrical pulse (stimulation electrode) of very short duration. The stimulus generates a muscle contraction in the target muscle, and its intensity must be supramaximal in order to excite all A-alpha fibers. Furthermore, a ground electrode is also necessary, providing a zero-voltage reference point. With two self-adhesive surface recording electrodes that are placed on the muscle belly (pick-up electrode) and on the tendon of the target muscle (reference electrode), the compound muscle action potential (CMAP) arising from the activated muscle fibers is recorded. The stimulation can be carried out proximally to the recording electrodes at different sites of the nerve course (e.g., wrist, forearm, elbow, upper-arm, and Erb’s point) [9].
In the case of deeply situated peripheral nerves, such as the lumbar plexus, stimulation is also possible with needle electrodes or, alternatively, by means of transcutaneous magnetic stimulation (TMS). This also applies to deeper lying muscles, where the CMAP can also be recorded with needle electrodes. However, these special techniques are discussed elsewhere [2, 3].
Neurophysiological convention specifies that negative voltage results in an upward deflection of the CMAP. Taking this fact into account, the routine technique described above allows a couple of parameters derived from the CMAP, that are used in the diagnosis of peripheral nerve diseases to be measured and calculated (Fig. 5.1a) [9–12]:
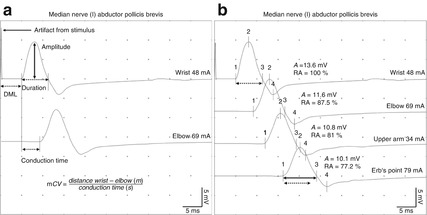
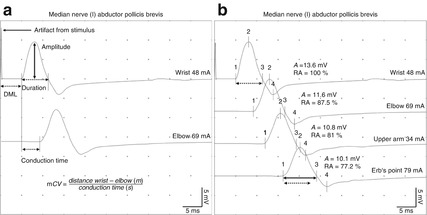
Fig. 5.1
(a) Important parameters derived from motor nerve conduction studies and calculation of the motor nerve conduction velocity. (b) Physiological “temporal dispersion.” Note the amplitude decay (RA, relative amplitude) and the prolonged duration of the compound muscle action potential (CMAP) recorded after proximal stimulation compared with the CMAP obtained after distal stimulation
Distal motor latency (DML) is the time between stimulus onset and onset of the negative peak of the CMAP. It reflects the time required for conduction through the distal axons (fastest A-alpha fibers), the neuromuscular transmission time, and the time needed to generate a muscle action potential. It is measured in milliseconds.
CMAP Amplitude is measured from the baseline to the negative peak of the CMAP. It is determined by the number of activated muscle fibers. It is measured in millivolts.
Duration is defined as the time from the onset of the CMAP to the return to baseline at the end of the CMAP. It reflects the range of conduction velocities within the nerve. It is measured in milliseconds.
Conduction time is measured in milliseconds on the basis of the difference between the motor latencies after proximal and distal stimulation.
Motor nerve conduction velocity is calculated for every nerve segment after distal and proximal stimulation by determining the quotient of the distance between the two stimulation points over the conduction time. It is measured in meters per second. The minimum distance between the two stimulation points should be at least 10 cm to avoid inaccuracy [9–12].
Note that the larger the distance between the stimulation and recording electrodes, the longer the duration of the CMAP owing to an increased range of conduction velocities. This phenomenon is additionally characterized by an increasing decay of the amplitude of the CMAP, also called temporal dispersion (Fig. 5.1b) [13, 14].
Age-matched normal values for NCS, including motor nerve conduction velocity, distal motor latency, and the CMAP amplitude of every single peripheral nerve are either derived from studies in the laboratories or obtained from related literature [2, 3, 9, 15].
There are many physiological factors affecting nerve conduction. Only a few are addressed here. Generally, nerves of the upper extremity conduct faster than those of the lower extremity. Moreover, the nerve conduction velocity in proximal nerve segments is faster than in the distal parts of a peripheral nerve [16]. The most important factors influencing NCS in the clinical routine are age and skin temperature. In infants and patients of advanced age, nerve conduction velocities are diminished. Furthermore, a decrease in skin temperature also decreases the conduction velocities [17–19]. Therefore, standardization and documentation of the skin temperature during electrodiagnostic testing is mandatory. In the case of low skin/room temperatures, a hot water bath or ceiling-mounted radiant heaters can be effective in raising the skin temperature of the limbs.
5.3.2 Demyelinating Disorders
Demyelinating disorders of the peripheral nerve result in impairment of conduction owing to the focal or generalized pathological features of the myelin sheath [11]. As a consequence, EDX demonstrates a slowing of the motor nerve conduction velocities and/or a prolongation of distal motor latencies. Depending on the type of nerve involvement, different patterns of motor nerve conduction velocity deterioration can be observed [2, 3, 9–12, 15]:
Focal: affecting only one nerve segment (e.g., in the early stages of entrapment in ulnar neuropathy at the elbow). An example is shown in Fig. 5.3a.
Multifocal: affecting certain nerve segments in one or more different peripheral nerves (e.g., multifocal motor neuropathy, acute and chronic inflammatory demyelinating neuropathy).
Generalized: affecting all nerve segments in different nerves (e.g., hereditary sensorimotor neuropathy type 1).
If only the distal nerve segment is affected, prolongation of the distal motor latency will result, whereas nerve conduction velocity and CMAP amplitude are normal. This applies in particular to the early stages of carpal tunnel syndrome, tarsal tunnel syndrome, and Guyon’s canal syndrome. In contrast to focal entrapments, a generalized demyelinating neuropathy leads to prolongation of the distal motor latencies in addition to a slowing of the nerve conduction velocities (Fig. 5.2). Distal motor latency is used for evaluation because the nerve conduction velocity of distal nerve segments cannot be calculated. DML can therefore be explained by the fact that the exact distance between the stimulation electrode and the endplate region of the supplied muscle is unknown. Moreover, distal motor latency contains the indeterminable time needed for neuromuscular transmission and the generation of muscle contraction (CMAP) [2, 3, 9–12, 15].
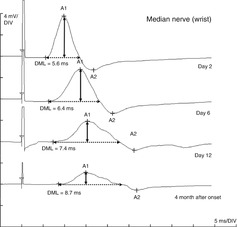
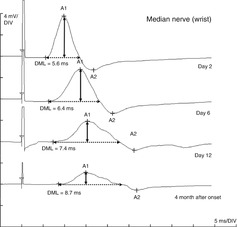
Fig. 5.2
Distal CMAP and distal motor latency (DML) of the median nerve in a patient with acute inflammatory demyelinating neuropathy 2, 6, 12 days, and 4 months after onset of the clinical symptoms. Note the prolonged DML due to the distal nerve segment being affected and pathological “temporal dispersion”
Apart from prolonged distal motor latencies and lowered conduction velocities, there are two other conditions that typically occur in demyelinating nerve disorders, referred to as “abnormal temporal dispersion” and “conduction block” [13, 14]. As mentioned above, in a healthy peripheral nerve the duration of the CMAP after proximal stimulation normally increases and the CMAP amplitude decreases compared with the CMAP recorded after distal stimulation. This feature is termed “temporal dispersion.” In the case of a demyelinating disorder, temporal dispersion is further increased because of the lowered conduction velocity and increasing heterogeneity of the motor nerve conduction velocities of different A-alpha fibers generating the CMAP. Consequently, besides the prolonged duration of CMAP and the decay of its amplitude, it appears to be split at and above the site of the lesion, whereas the distal CMAP remains normal (Fig. 5.3d) [9–12]. In scientific studies, different consensus criteria regarding the “abnormal temporal dispersion” have been published. According to the American Academy of Neurology, temporal dispersion is abnormal “if the CMAP obtained after proximal stimulation shows more than a 20 % decay of the amplitude and more than a 15 % increase of the duration compared with the CMAP obtained after distal stimulation” [13]. In contrast, the criteria of the American Association of Neuromuscular and Electrodiagnostic Medicine assume an abnormal temporal dispersion “if the duration of the proximal CMAP is prolonged by more than 30 % compared with the duration of the distal CMAP.” It is assumed that segmental demyelination over longer distances is responsible for this phenomenon [14].
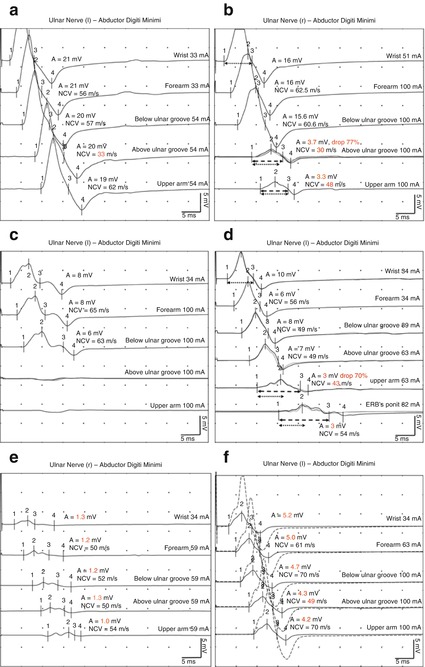
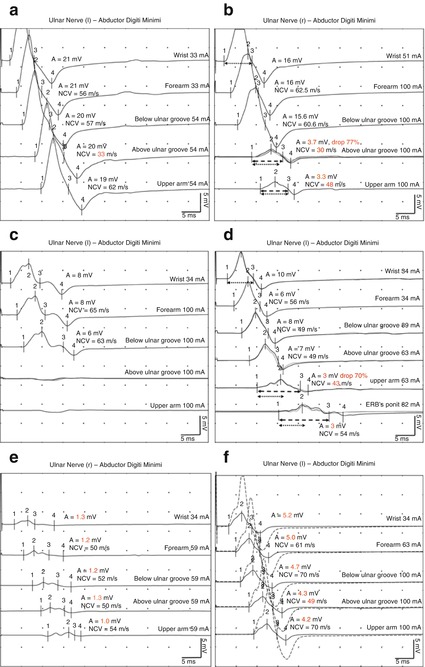
Fig. 5.3
Different types of demyelinating lesions on the example of ulnar neuropathy at the elbow obtained with fractionated motor nerve conduction studies. (a) Segmental slowing of the motor nerve conduction velocity. (b) Incomplete conduction block. (c) Complete conduction block. (d) Abnormal “temporal dispersion.” For details please refer to the text. (e) Pure axonal loss. The site of the lesion is not detectable with use of a motor nerve conduction study. (f) Both axonal loss and focal demyelination. Dotted line: CMAP of the ulnar nerve of the healthy side
On the other hand, a short segmental demyelination is attributed to a “conduction block.” If the conduction block is complete, a normal CMAP can be recorded after distal stimulation, whereas stimulation proximal to the lesion will generate no CMAP (Fig. 5.3c). An incomplete conduction block is assumed if the CMAP amplitude (or area under the curve) after proximal stimulation is reduced only when with the CMAP after distal stimulation [9]. Depending on the affected nerve, there are again different criteria defining an incomplete conduction block. According to the American Association of Neuromuscular and Electrodiagnostic Medicine, a “drop of more than 50 % in proximal CMAP compared with CMAP obtained after distal stimulation in the median and ulnar nerves and more than 60 % in the tibial and peroneal nerves is considered as pathological, while CMAP duration increases by no more than 30 %” [14]. In contrast, the criteria of the American Academy of Neurology require a “20 % drop of the (proximal) CMAP area or amplitude compared after distal and proximal stimulation” [13].
Unfortunately, the diagnosis process for demyelinating disorders of the peripheral nerves can become very challenging. Primary axonal loss is sometimes accompanied by secondary demyelination (Fig. 5.3f). This explains why slowed conduction velocities and prolonged distal motor latencies can occur in an axonal disorder [12]. Moreover, during the first few days after severe axonal nerve damage this condition will present a complete or incomplete conduction block because Wallerian degeneration has nor been completed yet and the segment distal to the damage is still conducting. This phenomenon has frequently been referred to as a “pseudo-conduction-block” [20]. Therefore, different criteria have been suggested, considering motor conduction velocities, distal motor latencies, and F-wave latencies. For instance, Van den Bergh and Pieret assume inter alia a motor conduction velocity slowing of <70 % of the lower limit of normal and a prolonged distal motor latency of >150 % of the upper limit of normal to be characteristic of a demyelinating condition [21].
5.3.3 Axonal Disorders
Axonal loss leads to a reduction of the activated muscle fibers and is thus paramount to a reduction of CMAP amplitude. In a fractionated motor nerve conduction study this applies to all generated CMAPS – distally and proximally. As a consequence, and in contrast to focal demyelinating disorders, a localization of the lesion with motor NCS is impossible (Fig. 5.3e) [10–12]. This important lack of diagnostic power can be solved by needle electromyography and nerve imaging (see Sect. 5.6 and Chap. 6) [6, 22]. Furthermore, at an early stage (days 0–14) after onset of an axonal injury, this presentation can be misdiagnosed as a conduction block (see Sect. 5.3.2) [20]. If axonal loss also includes the fastest conducting A-alpha fibers, motor nerve conduction velocities may also be reduced. However, the extent of conduction slowing is less than following nerve fiber demyelination, and it usually ranges above 70 % of the lower limits of normal [2, 3, 21].
5.4 Sensory Nerve Conduction Studies
5.4.1 Technique and Parameters
As in a motor NCS, the peripheral nerve is stimulated with a transcutaneous depolarizing square wave electrical pulse (stimulation electrode) of a very short duration. However, a lower stimulation intensity is required than in a motor NCS. In contrast to motor NCS, a self-adhesive recording electrode can be placed either distally (antidromic recording) or proximally (orthodromic recording) to the stimulus site because the evoked electrical activity propagates in both directions. Furthermore, a ground electrode is also necessary [9]. In clinical practice, antidromic recordings are preferred, mainly because the amplitude of the sensory nerve action potential (SNAP) is larger than that obtained by orthodromic recordings [10–12]. Sensory nerve conduction studies can also be performed with needle electrodes; however, these special techniques are discussed elsewhere [2, 3]. Compared with CMAPs, SNAPs are smaller and measured in microvolts. Therefore, an averaging of several SNAPs is required to improve the signal-to-noise ratio (SNR). Most important parameters derived from sensory NCS are as follows (Fig. 5.4) [10–12]:
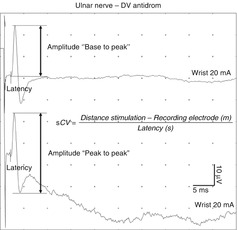
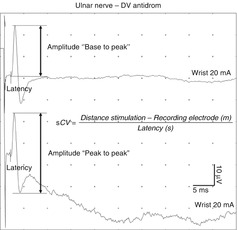
Fig. 5.4
Important parameters derived from sensory nerve conduction studies and calculation of the sensory nerve conduction velocity
SNAP amplitude reflects the number of the largest activated sensory nerve fibers (A-alpha). It is measured (base to peak or peak to peak) in microvolts.
SNAP conduction velocity is easily calculated using only a single site measurement because the distance between the recording and stimulating electrodes and the sensory latency is directly measurable. It is measured in meters per second.
As mentioned above, only the largest and fastest conducting sensory A-alpha fibers, which functionally supply senses of touch, position, and vibration senses, can be assessed using sensory NCS. Usually, small fiber neuropathies affecting C-fibers mediating pain do not show any abnormality in conventional sensory NCS [4, 8]. Quantitative sensory testing and autonomic testing or skin biopsy are necessary to provide the correct diagnosis in this situation. These special techniques are described elsewhere [2, 3].
5.4.2 Demyelination Disorders
As in motor NCS, the primary feature of demyelination is a reduction of sensory nerve conduction velocity [10–12]. Additionally, the duration of SNAP increases owing to an enlarged range of nerve conduction velocity. It leads to “temporal dispersion” and “phase cancellation” (Fig. 5.5). Consequently, the SNAP amplitude will also drop in a demyelinating disorder [10–12, 23].
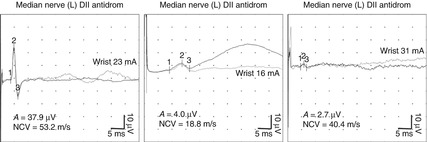
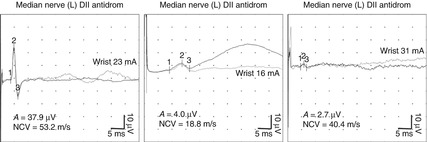
Fig. 5.5
Sensory nerve conduction studies of the median nerve. Left: physiological. Middle: demyelinating disorder with slowing of motor nerve conduction velocity and “temporal dispersion.” Right: pure axonal loss
5.4.3 Axonal Disorders
Axonal disorders will primarily reduce the amplitude of the SNAP generated after electrical stimulation, whereas its duration is normal. As in motor NCS, further axonal loss of the fastest conducting fibers will also lead to a modest slowing of the sensory nerve conduction velocities (Fig. 5.5) [10–12, 21, 23].
Sensory NCS are useful to determine whether the site of the nerve lesion is pre- or postganglionic. In the case of a preganglionic lesion the connection between the cell bodies of the dorsal root ganglion and the peripheral nerve remains intact. Consequently, a normal SNAP and conduction velocity can be obtained by sensory NCS, despite anesthesia in the dermatome supplied by that root. However, an absent or pathologically changed SNAP indicates a postganglionic lesion either of the nerve plexus or of a single peripheral nerve [24]. Apart from this electrophysiological dogma, in some recent studies it has been shown that – in a small percentage of subjects (2.4–12.1 %) with preganglionic lesions due to a L5 or S1 radiculopathy – the SNAP amplitude was abnormal. This exception may be due to dorsal root ganglion compression proximal to the spinal foramen or intra-spinally, and should be always kept in mind [25, 26].
5.5 Long Latency Reflexes (F-Waves)
5.5.1 Technique and Parameters
F-waves are a type of late motor response. Their name is derived from the foot, where they were first described. With a reversed stimulation electrode, F-waves can be recorded in the same setting as stated in Sect. 5.3.1 concerning motor nerve conduction studies [9]. If a nerve is electrically stimulated above its final segment the action potential propagates in both directions.
The orthodromic propagated action potential generates CMAP, also known as an M-response. On the other hand, the antidromic propagated action potential reaches the anterior horn cell body and depolarizes the axon hillock. As a result, a small portion of the alpha-motor neurons backfire and a second orthodromic action potential reaches the muscle. This causes a late muscle depolarization that involves only a small portion of the muscle fibers, referred to as an F-wave [27–31]. Recording F-waves will therefore allow the electrodiagnostic testing of long and proximal nerve segments (e.g., the lumbosacral plexus or nerve roots) which are inaccessible to conventional electrical nerve stimulation [29]. In contrast to the M-response, F-waves usually vary in latency and shape because different populations of motor neurons backfire with each stimulus. Therefore, normally, “10–20 consecutive stimulations will be needed and the most reliable measure of the F-wave is its minimum latency of 10–20 firings” [29, 31]. It strongly depends on the body height, which should be taken into account. Moreover, the “F-wave persistence” is commonly used and represents the number of F-waves obtained per number of stimulations [27–31]. Regarding other parameters (dispersion, amplitude–ratios) derived from F-wave studies please refer to specialist literature [29].
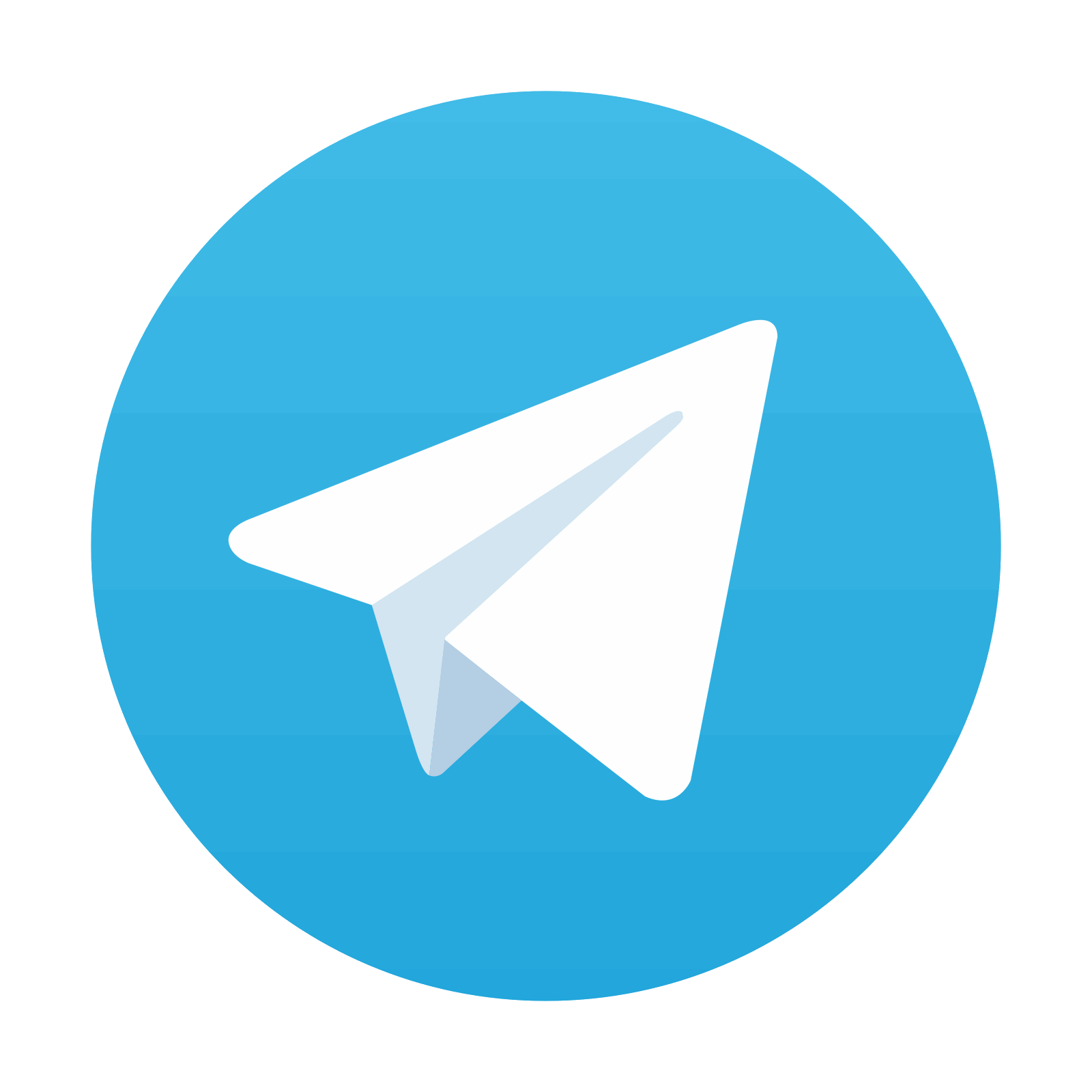
Stay updated, free articles. Join our Telegram channel

Full access? Get Clinical Tree
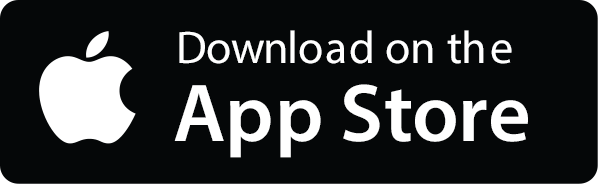
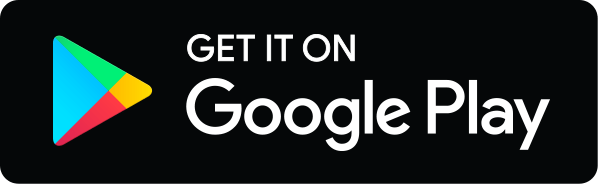