Introduction
Nearly a third of patients with epilepsy fail to respond to two or more appropriately selected and well-tolerated antiseizure medications and are diagnosed with drug-resistant epilepsy . Medication resistance in epilepsy is a persistent concern despite the continuously expanding options of antiseizure medication. Approximately 50%–75% of children with medication resistance will benefit from undergoing epilepsy surgery, with resultant seizure remission and a significant improvement in their quality of life .
To attain complete seizure freedom following epilepsy surgery, a complete resection of the epileptogenic zone (EZ) is mandatory. EZ is defined as the minimum area of cortex that is necessary to initiate seizures and upon removal of which, complete seizure freedom can be expected .
Accurate identification of the EZ remains a challenge and despite significant advances in available presurgical evaluation modalities and techniques, the overall postsurgical seizure freedom rates still linger around 50% .
It has been discussed that the concept of one EZ limited to a single brain structure, might be too simplistic and multiple interconnected EZs exist in some patients . This matches with the EZ rather consisting of a network of hyperexcitable, interconnected regions likely extending far beyond one brain region. The epileptogenicity of this brain network is determined by the level of excitability and interconnectivity . This concept is in line with the observation that surgical success is decreasing over time and relapses can be observed after a prolonged period of seizure freedom. New seizure might then be generated from residual, previously dormant, epileptic cortical tissue farther downstream from the originally resected epileptogenic lesion .
This proposes a challenge when it comes to defining the extent of a planned surgical resection. The Pediatric Epilepsy Surgery Task Force of the International League Against Epilepsy (ILAE), concludes that an ictal scalp electroencephalography (EEG) with video and a 3D volumetric magnet resonance imaging (MRI) are mandatory steps in a pre-surgical evaluation . The complexity of surgical cases often mandates more diagnostic tests like functional imaging. In patients in whom noninvasive tests are inconclusive, intracranial EEG is used to improve surgical precision. Trends in epilepsy surgery over time suggest that the percentage of intracranial EEG especially stereotactically implanted depth electrodes has risen over the past 10 years, which can be attributed to an increase in complex often nonlesional patients . At the same time new analysis methods and electrode types have been developed which caused a surge in investigating new potential EEG biomarkers. In this chapter we will contrast traditional and novel biomarkers that are used in intracranial EEG.
Traditional biomarkers of epileptogenicity
Interictal spikes
Interictal spikes, also known as the interictal epileptiform discharge (IED), for a long time have been the most important interictal biomarker for epileptic tissue. The neuronal correlate of interictal spikes is the paroxysmal depolarization shift, which is a large amplitude depolarization potential originating in a pyramidal cell/neuron; the principal generator of almost all electrographic activity of the brain .
In scalp EEG IEDs are often described as sharply contoured events with a negative peak. In contrast IEDs in intracranial EEG can have both positive and negative peaks and occur in a large variability of shapes and patterns. The latter can be predictive of a type of epileptic lesion such as rhythmic spike runs and continuous spiking over focal cortical dysplasia (FCD). It is also important to note that some brain regions generate physiological spikes, that can be misleading to the unexperienced reader. This is for example the case in high-amplitude spikes occurring over the hippocampus .
It is well established that epileptic spikes are sensitive to identifying epileptic areas but not very specific. Per definition the area generating spikes, labeled the irritative zone is only partially overlapping with the EZ . It is well established that patients can be seizure-free even if not all spike-generating brain regions are removed . Thus, the persistence of IEDs after successful epilepsy surgery does not necessarily depict failure of treatment or persistence of seizures nor does their rate of occurrence correlate with the anticipated seizure frequency in the matter of a relapse . Additionally, it can be hard to separate between spike origin and propagation in clinical practice. Often spikes are seen over wide-spread areas as a propagation phenomenon. This is often seen when spikes and seizure onset originate from a deep source and bottom of the sulcus and can lead to mis-localization of epileptic areas .
Interictal electrographic patterns
Certain other, non-IED, electrographic patterns have been identified interictally as well as pre- or periictally which could be considered useful as biomarkers of epileptogenicity of a cortical region of interest. Interictal patterns that were commonly observed in intracranial EEG include low voltage fast activity, persistent focal electro-decrement, or the presence of delta brushes . However, the quality and the quantity of available evidence to guide the utilization of these biomarkers remain sparse, which in itself is a limitation.
Seizure onset zone and ictal onset patterns
The current gold standard for delineating margins for epilepsy surgery is the recording of spontaneous seizures and identification of the seizure onset zone (SOZ). The identification of the SOZ however is not without pitfalls. First, false localization due to fast propagation is common especially when deeper foci are involved. Additionally intracranial electrodes can only record activity from their direct vicinity. As a result, electrode placement influences the ability to identify SOZ areas correctly. Overall studies show varying success of surgery after complete removal of the SOZ .
Special emphasis has been placed on trying to identify ictal onset patterns that are specific to the SOZ and confirm that the first ictal activity observed in a patient is not the result of propagation phenomena. In one study, seven seizure-onset patterns were identified across 53 sampled seizures, namely, low-voltage fast activity (43%); low-frequency high-amplitude periodic spikes (21%); sharp activity at ≤13 Hz (15%); spike-and-wave activity (9%); burst of high-amplitude poly-spikes (6%); burst suppression (4%) and delta brush (4%) . Each pattern occurred across several pathologies, except for periodic spikes, which were only observed in association with mesial temporal sclerosis and the delta brush pattern which occurred exclusively in FCD . While these onset patterns are a key indicator of SOZ areas, no evidence systematically analyses that observation of any of these SOZ patterns in the region of the surgical resection correlates with postsurgical seizure outcome. Moreover, the utility of SOZ has lately been challenged by observations from long-term intracranial recording devices. These recordings suggest that SOZ areas in individual patients may change over time especially in patients with multifocal epileptic activity. In patients with bi-temporal epilepsy, for example, the predominant seizure onset may alternate between mesio-temporal structures over time .
Novel biomarkers of epileptogenicity
As detailed above, epileptologists still struggle to predict surgical seizure outcomes in individual patients and no perfect gold standard exists to ensure best possible prediction. While we know that some clinical indicators such as nonlesional epilepsy, incomplete lesion resection during surgery or widespread interictal activity prior to surgery are negative prognosticators for outcome, precision to predict good outcome is limited.
Recent developments in presurgical diagnostics increasingly focus on multimodal analysis with the aim of allowing surgical interventions for more candidates and improving surgical outcomes. This chapter focuses on the use of stereo-EEG and associated biomarkers. For many of these new approaches the same limitations apply as discussed for classical biomarkers. Namely, the limited spatial coverage time constraints when recording SEEG as well as the lack of evidence from clinical trials to prove the efficacy of a novel diagnostic approach.
We identified two general approaches in the development of new biomarkers. First, those that expand the conventional frequency spectrum of the cortical electrographic activity such as high-frequency oscillations (HFOs) and infra-slow activity (ISA). Second, those that use advanced computational analysis to assess the epileptic network such as the epileptogenicity index (EI).
Exploring the frequency spectrum
Cerebral neuronal circuitry is capable of producing a wide range of electrical oscillations spanning over five orders of magnitude in frequency . By convention, the focus of electrophysiologists has always been narrowed down upon a limited bandwidth of electrographic frequencies. Over the past couple of decades, a growing interest was observed in exploring a more wider spectrum of electrographic frequencies that the human brain is capable of producing in health and in disease. This includes expanding the frequency analysis below 1 Hz on the lower end and above 30 Hz on the higher end of the spectrum. Many of these analyses have become possible due to advanced amplifier technology allowing sampling rates suitable for these frequencies and signal-to-noise ratios that do not interfere with analysis of very small spectral changes. Research studies have focused on both ictal as well as interictal changes. Interictal analyses have the advantage that they do not require recorded seizures during the diagnostic test and are easier to apply. Often interictal noise and artifact levels facilitate analysis as well. On the other hand, there is a worry that interictal biomarkers are less specific to epileptogenic areas as has been described for epileptic spikes.
Another challenge that newly developed biomarkers face is the necessity to meet rigorous validation in clinical trials that allow them to be implemented in clinical care. For many biomarkers we have larger datasets that look at retrospective group evidence that a marker is linked to the SOZ and even that removal of the marker correlates with postsurgical seizure outcome. This level of evidence however is very different from being able to prospectively predict seizure outcome in an individual patient or tailoring a resection according to biomarker distribution.
High-frequency oscillations
HFOs are defined as spontaneous EEG or magneto-encephalographic (MEG) events in the frequency range between 80 and 500 Hz, consisting of at least four oscillations that clearly stand out from the background activity . Frequencies between 80 and 250 Hz are further subdivided as ripples while the frequencies that are between 250 and 500 Hz are identified as fast ripples and very-fast ripples would even exceed 500 Hz in frequency . HFOs are thought to be generated via multiple mechanisms . Physiological HFO that ubiquitously occur in cortical regions under natural circumstances reflect summated excitatory postsynaptic potentials. Pathologic HFO in the frequency range of either ripples or fast ripples reflect summated action potentials of synchronously bursting neurons or principal cell action potentials .
When it comes to the clinical utility, HFOs are probably some of the best studied biomarkers. First studies explore the relationship between HFOs and the SOZ. A strong argument for the link between fast ripples and epileptogenicity is the observation from the kainate model of epilepsy that fast ripples were found in the hippocampus of rats that developed chronic spontaneous seizures but were not found in rats that did not develop epilepsy . In patients with epilepsy this link naturally cannot be established because all patients investigated with intracranial electrodes have chronic seizures. In addition, intracranial EEG methods are not perfect when it comes to sampling only epileptogenic tissue and avoiding healthy brain tissue. For these reasons, only correlative evidence exists to establish a link between HFOs and the presumed epileptic tissue in patients. When it comes to proving HFOs are biomarkers of epileptic tissue, this link is maybe the weakest but also easiest to test. Most previous studies were retrospective group analyses, and current results show that in groups of patients the average rate of HFOs on electrodes recording from the SOZ is significantly higher than outside of it . However, the highest HFO rate does not occur in the SOZ for every patient. And, while these studies support the general finding of increased HFO rate in the SOZ, the results were not completely identical. Some studies found HFO superior to interictal EEG spikes while others did not . Moreover, some studies suggest a stronger link between ripples (80–250 Hz), rather than fast ripples, and the SOZ . Many factors contribute to this variability including different recording methods, different patient groups, and different epilepsy etiologies. Yet, despite this variability, the fact that HFOs are associated with the SOZ in groups of patients with epilepsy is reassuring rather than discouraging.
Studies clearly indicate HFOs exist beyond the SOZ , which again is unsurprising since it is generally accepted that the volume of brain tissue responsible for generating seizures extends beyond the SOZ and HFOs are present in normal brain tissue . To prove that HFOs are relevant to seizure generation in patients, one must establish a link between the removal of HFO-generating tissue and seizure outcome . Studies on seizure outcomes were designed in a very similar way to the SOZ studies . In general, these studies tested the hypothesis that patients would be seizure-free if most contacts with HFOs were removed, while patients would continue to have seizures if contacts with HFOs remained ( Fig. 3.1A ). Most seizure outcome studies were performed retrospectively using group analysis, but the first studies had single patient data, and showed in some surgical patients that the majority of HFOs were removed yet seizures continued . It was proposed that these findings do not discount HFOs as reliable markers of epileptic tissue; intracranial electrodes are always limited in their spatial coverage, and some HFOs may exist beyond the sampled brain areas . The fact that studies from different centers using different analysis methods essentially replicated earlier results was reassuring . However, metaanalysis of several larger studies showed the link between the removal of HFOs, and seizure freedom is weak , and none of the studies at this time would satisfy the criteria for a high-quality trial as, for example, measured by Cochrane .

Generally, prospectively designed studies have less potential for bias (e.g., investigator bias, loss to follow-up) and confounding effects (e.g., type of epilepsy or seizure type, location of SOZ) than retrospective studies. In the context of HFOs and epilepsy surgery, prospective studies can be performed using a group or individual patient approach. Most prospective HFO studies conducted thus far were observational, meaning that HFOs were not used to tailor a resection. Two studies performed at the group level show it is possible to use HFOs to predict 1-year seizure outcomes . This information, however, is less relevant to a patient who would want an individual prediction of his or her surgical outcome. In this regard, the results of HFO studies are not convincing. Two complementary scenarios were observed: (1) either all sites with HFO were removed and the patient continued to have seizures or (2) brain regions with frequent HFOs remained and the patient was seizure-free. The results from two prospective studies show that 6 of 37 (16%) patients did not become seizure-free despite removing most of the HFO-generating tissue. By contrast, 14 of 35 (40%) patients became seizure-free after incomplete removal of HFO . These results challenge the reliability of HFOs as a biomarker for epilepsy. We provide below some explanations for these results (Sections 2.3 and 2.4) and hope for future studies to improve HFO analysis and enhance clinical utility (Sections 2.5, 3, and 4).
Most recently a prospective randomized controlled trial was completed using intraoperative ECOG to identify HFO and tailor the surgical resection according to the HFO distribution . This trial was a noninferiority trial aiming to prove that HFO performs similarly to using interictal spikes in regard to identifying epileptic areas. This trial could not show that HFO are as good as epileptic spikes to tailor the resection when looking at all patients. Pathologies associated with poor prognosis were found to be a confounder in the HFO-tailored resection group. After correcting for confounders, HFO were noninferior to epileptic spikes in patients with neocortical epilepsy onset. Even if this trial did not show the expected superiority of HFO compared to classical EEG markers, it is a milestone in neurophysiology research and evaluations of presurgical diagnostics as it is at this point the only randomized controlled evidence in the field.
The discussed results challenge the reliability of HFOs as a biomarker for epilepsy. In the following we provide some possible explanations for these results, which are not unique to HFO and should be considered whenever establishing a biomarker for epileptic tissue.
A first challenge to consider is the fact that HFOs are found not only in epileptic tissue; oscillations in the same frequency band are generated during physiological activity . Ripples in the mesio-temporal structures are clearly linked to memory consolidation , and fast ripple-frequency oscillations have been linked to sensory function in the central and occipital brain areas . Thus, some of the remaining HFOs in seizure-free patients might not be pathological at all ( Fig. 3.1D ). No methods exist to differentiate normal from pathological HFOs within the same brain regions. Compounding this issue is evidence for normal and pathological HFOs in the same tissue , and recent data from the normal brain stereo-EEG brain atlas suggesting that normal HFOs are much more abundant than previously thought . Attempts to identify normal HFOs by establishing a link with other physiological events, such as sleep slow waves or spindles, are promising but have not been tested in a prospective surgical setting . An alternative approach is to use only HFOs with interictal spikes to identify pathological HFOs. This limits the HFO analysis to brain regions with interictal spikes however, in some types of epilepsy, this could be an advantage rather than a limitation.
The second challenge is finding the right threshold when interpreting HFO data ( Fig. 3.1B ). In most patients, there are some brain areas with very frequent HFOs, many areas with moderate HFOs, and fewer areas with little or no HFO activity. This leaves epileptologists with the same challenge associated with interpreting interictal spikes to delineate the irritative zone: how to threshold the events. How much HFO activity is required to identify pathological brain regions, which areas with HFOs can be ignored, or should any area with HFOs be ignored? In retrospective studies, some groups addressed this challenge by using thresholding methods like the “upper fence” , which help to individually determine the area of highest HFO activity. It is important to note that these threshold measures are not based on any mechanistic understanding but are instead motivated by statistical notions. In addition, HFO rates change—for example, with sleep stage, medications, lesion type, or extended recordings (see Section 4)—which may cause any type of thresholding to fail.
Thresholding is only one of many methodological problems when analyzing HFOs in a prospective fashion. Different automatic detection approaches, electrode types, or recording methods may differ in reliability when analyzing HFOs . A multicenter trial led by some of the authors aimed to address these concerns. However, neither thresholding, different recording methods, different detector types, nor different statistical approaches generated a reliable prediction of seizure outcomes using HFOs .
Last but not least HFO like other epileptic activity might not be isolated localized events, but also be connected via networks ( Fig. 3.1C ). New thinking has emerged from a study using pre- and postoperative acute electrocorticography that might help to explain results from prior HFO studies . The authors showed that the distribution of HFOs is variable before and after a surgical resection. This observation suggests that HFOs are organized in networks, and the removal of a core generator could leave remote sites silent. This concept cannot be tested in the same way in chronic stereo-EEG recordings as for ethical reasons patients are not recorded with intracranial EEG after surgery. As illustrated in Fig. 3.2 the network concept is very similar to what we know from interictal spikes, that is, some areas with spikes might be due to propagated activity. Currently no measure has been established to differentiate between the core and the propagating sites of HFOs. Van’t Klooster and coworkers suggest that the combined analysis of pre- and post-operative HFO distributions helps to provide a better predictor for outcome on an individual basis . Meanwhile more studies suggest that HFOs can form networks of variable sizes and should not be interpreted as standalone events . These results are consistent with the notion of complex epileptic networks, and fundamentally differ from the existing approach to HFO analysis. Historically HFOs were considered to have very focal generators, local impact, and no propagation. These concepts should be reconsidered in light of recent results, and future HFO analysis should focus on interactions between HFOs in separate brain regions as well as their variable impact on seizure generation.

In summary, HFOs are promising biomarkers of epileptic activity, but recent studies which focus on higher-level evidence data, like prospective studies and randomized trials have dampened the enthusiasm for HFO being a simple ubiquitously used new biomarker. Rather we have learned that a deeper understanding and better methodology is necessary prior to widespread application.
Ictal infra-slow activity
ISA has been traditionally invisible in EEG, as a lower filter setting was set to eliminate activity below 0.5 Hz. More recently lower frequency bands in the subdelta range (<0.5 Hz) have started to gain popularity and electrophysiologist’s interest . In contrast to the work in HFO, ISA has been mostly analyzed in the preictal and ictal periods. This is mostly related to the fact that ISA is a very slow and long-term change that occurs regularly over different brain regions and then is nonspecific to epilepsy. To use the method specific to epileptic activity analysis around seizures is necessary to identify a time point around which epilepsy specific changes in ISA might occur. Very few studies looked at interictal very low activity and its clinical relevance is currently unclear. Underlying mechanisms are likely different from ISA .
In feline models with experimental tonic-clonic seizures, depolarization of the pyramidal neurons caused a negative direct current (DC) shift when recorded using conventional DC amplifiers . This gradually declined and changed into a positive shift during the postictal period. Further studies in feline models showed that these DC shifts had a horizontal dipole with a maximum amplitude at the focus and a field extending to a perimeter of 10 mm . In human epilepsy, ictal DC shift was first described by Ikeda et al. with the use of subdural electrodes in three patients with focal refractory epilepsy .
A slow-rising negative potential (DC shift) was observed in a localized area, 1–10 seconds prior to the observed conventional ictal discharges . The terms slow DC shift (slow DC shift), ISA as well as ictal baseline shifts (IBSs) have been used interchangeably to describe this electrographic phenomenon which is in the range of subdelta (<0.5 Hz) frequency range.
The term “DC shift” stems from the use of a DC amplifier based on the fact that in the earlier studies slow baseline shifts could not be appreciated on conventional EEG recordings obtained with alternative current (AC) amplifiers. This was because of the short time constant used in low-frequency filters. However, it was demonstrated that with the use of AC amplifiers coupled with a low frequency filter harnessing a longer time constant, ictal DC shifts could be recorded in humans, in intracranial as well as on scalp EEG recordings . Therefore, either ISA or IBS are better-suited terms in describing this particular electroencephalographic phenomenon.
The mechanism by which the ISA originated has been widely debated. Experimental feline epilepsy models as well as nonhuman primate (Baboons) models have demonstrated a rise in extracellular potassium associated with the ictal DC shifts secondary to the failure of glial mechanisms which buffer this system .
As mentioned above, ISAs are ubiquitous throughout the brain and not specific to epileptic areas. Ictal ISAs however were found to be either preceding, co-occurring with or following the conventional EEG ictal onset, and had a duration ranging between 1.9 and 2.9 seconds .
Extensive intracranial coverage shows that ictal ISA can be spatially distributed over a wide surface area . However, its true spatial extent is generally smaller than the area defined by conventional frequency activity in both neocortical and temporal lobe epilepsy patients .
It is therefore a challenge of the method to identify spatially restricted ictal ISA that are linked to epilepsy and delineate the SOZ. Currently no prospective trials exist that assess the sensitivity or specificity of ISA to improve outcome prognostication. Evidence from smaller studies suggests that ISA can improve the precision for identifying epileptic areas. Most of these studies as retrospective and focus on identifying the SOZ . In a prospective study of six patients, full resection of areas with ictal ISA and partial resection of the SOZ defined by conventional EEG frequencies, led to Engel class I outcome in 2/6 and Engel class II in 4/6 . 5 out of 6 patients with eventual class I outcomes had the DC contacts that were resected in a separate study .
Overall, the evidence for ISA in the presurgical setting is lower than for other biomarkers such as HFO. On reason is the methodology used for most studies. DC coupled amplifiers have been used historically in the past to record slow EEG baseline shifts. However, most researchers studying slower EEG fluctuations nowadays use AC amplifiers instead, which are more stable compared to DC amplifiers . Pure, unaltered DC recordings can only be achieved using nonpolarizing silver/silver chloride electrodes which are potentially toxic to human brain parenchyma . For a successful recording, apart from using an AC amplifier with a longer time constant, using platinum electrodes spread over a larger surface area (e.g., subdural as opposed to depth electrodes) and using a higher input impedance of the amplifier (>50 MΩ) can overcome some of the technical difficulties . Recent studies suggest that different time constants for ISA exist which would allow use of regular amplifiers in some circumstances .
Overlap between spikes, high-frequency oscillation and infra-slow activity
Interictal epileptic discharges, HFO and ISA are usually not analyzed with the same EEG setting and require different time resolution, filter settings and amplification. As a result, understanding the interactions between these different biomarkers and their relation to epileptic tissue is not trivial.
Early on it was discovered that spikes and HFO can occur both together and independent of each other . Some studies referred to the high-frequency content of epileptic spikes , while others identified HFO riding on epileptic events as a separate biomarker entity. There has been some suggestion that spikes with HFO are more specific to the seizure outside and surgical outcome than spikes or HFO alone . HFO coupled to spikes have also been used to differentiate between physiological and epileptic HFO . One study looking at simultaneous micro-macro electrode recordings identified a specific multiunit activity firing pattern that separated spikes with and without HFO occurrence ( Fig. 3.2A–C ). Overall, it can be concluded that analyzing the co-occurrence of spikes with HFO can provide additional information and overcome the individual limitations of each biomarker. While HFOs are specific but not sensitive enough for epileptic tissue, spikes are more sensitive but not as specific ( Fig. 3.2D ).
In a similar way that ISA had been compared to classical seizure onset patterns, studies have focused on looking at ictal HFO changes and ISA. ISA and HFO were observed to co-occur over the EZ during seizures . Ikeda et al. discussed two novel concepts of “ictal active DC shifts” and the co-occurrence of slow oscillations and HFO labeled as “Ictal Red Slow” activity as a possibly promising EEG biomarkers with a higher sensitivity of localizing the EZ . In summary studies that compare ISA and ictal HFO suggest three things; first both events are seen over the same brain regions, second both events identify smaller and more spatially restricted areas than interictal spikes, third, both events are closer linked to the seizure onset than classical seizure onset patterns .
Biomarkers looking at network changes
Recent methodological advances reveal the importance of network changes in understanding epileptic areas and predicting postsurgical outcomes. Increased connectivity between seizure onset areas and other brain areas can suggest a larger epileptic network and be a negative predictor for surgical outcomes. Many of these studies focus on fMRI, scalp EEG or MEG but some methods are specifically developed for intracranial EEG. This chapter focused on one example that has gained wider acceptance, the EI.
Epileptogenicity index
The EI was introduced as a new quantitative measure of characterizing the epileptogenic potential of the involved anatomical brain structures and is a measure of both epileptogenicity and connectivity. It is based on the assumption that there is an increase in rapid discharges and activity during the transition of an interictal pattern into an ictal pattern on intracranial recordings . Often there is an increase in beta/gamma activity accompanied by a voltage attenuation. EI takes into account, both the spectral and temporal properties of the fast brain activity as well as the occurrence and interaction of this activity between brain regions covered by electrodes and involved in seizure generation. It characterizes the ability of a brain structure to generate fast oscillations during a seizure and the time delay in producing these in comparison with the time of seizure onset (t0). EI functions semiautomatically, ranking each of the intracranial electrodes based on the tonicity of the observed rapid discharges at ictal onset and the time delay in involving the particular electrode. The quantification process gives a value between 0 and 1. The developers of EI suggest that any electrode area with an EI of more than 0.3 can be identified as epileptogenic.
EI has been designed to allow a more automatized identification of brain areas that are involved in seizure generation. Its use can avoid bias in visual review. Studies that compared retrospectively EI values with visually identified SOZ areas showed a high correlation between both regions. In a group of 17 patients with mesial temporal lobe epilepsy EI values were computed in mesial and lateral structures of the temporal lobe. Structures which were involved early in the ictal process and produced rapid discharges at seizure onset demonstrated statistically high EI values . The highest EI values were computed from signals recorded in mesial structures in the majority of patients. Similar findings were observed in a mixed group of patients with temporal and neocortical epilepsy suggesting that widespread EI increases were linked to poorer seizure outcomes after surgery .
A particular limitation of the EI derives directly from the fact that it highly depends on changes in high-frequency activity. Ei will not be increased when ictal patterns do not involve fast activity, but rather present with either rhythmic spikes or slow waves as seen with a quarter to one-third of intracranial ictal patterns . Thus, some EI might fail to identify some seizure-onset areas.
The connectivity EI (cEI) was proposed as a continuous development from EI to overcome the inability of EI to evaluate slower electrographic onset patterns .
CEI measures changes in functional connectivity between SEEG signal trends shorty prior to and around the seizure onset . It combines a directed connectivity measure based on the nonlinear correlation coefficient ( h ²) and graph theory measures (out-degrees) with the EI. Like with EI there is a strong correlation between visually identified SOZ areas and high cEI measures. Data from 51 patients showed that cEI has a better yield in marking SOZ in seizures with slow onset patterns. The removal of EI areas has also been linked to better seizure outcomes.
In contrast to the individual biomarkers discussed in the beginning of this chapter EI and cEI do not only aim for an improved identification of epileptic tissue but also focus on automatizing the identification of epileptic areas. This reflects a constant movement from visual analysis which is largely reviewer dependent to more objective methods like automated assessment. A recent publication might be a peek into future applications and introduce the concept of virtual epileptic patient brain modeling. This method simulates seizure data using a virtual brain and combines information from stereoelectroencephalography, anatomical MRI data, connectivity measures and a computational neuronal model. In the publication the ability to identify epileptic areas was similar to that of cEI and resection of identified areas correlated with postsurgical seizure outcome .
Summary
Despite the widely accepted notion that complete resection of EZ leads to best surgical outcomes and seizure freedom, there is currently no single universally accepted biomarker to precisely delineate the EZ in medically refractory epilepsy, either invasively or noninvasively.
Over time, several different biomarkers have been suggested with a wide heterogeneity in the quality of available evidence. This chapter focuses on methods applied during the phase 2 evaluation of a presurgical investigation using intracranial recordings.
There is a significant lack of class 1 evidence to guide surgery. What has been conventionally used is a combination of complementary traditional and novel biomarkers in conjunction, to derive an accurate electro-clinco-anatomical correlation.
The most conventional biomarker of the EZ is the interictal spike or the IED which we now know can be quite misleading. Nevertheless, it continues to be an essential component of the modern day presurgical work up in both invasive and noninvasive modalities.
Beyond this, there are only a handful of options available as novel biomarkers. None has been able to reach the gold standard status thus far defined by the qualities including but not limited to universal accessibility, affordability, reliability, and precision with minimal adverse effects on the patient. The biggest challenge in utilizing novel biomarkers is that each requires a high level of computational knowledge to be used in routine clinical practice.
Overall, a better understanding of the epileptogenic network versus the epileptogenic focus is necessary to improve postsurgical outcomes. Presumed focal epilepsies may not necessarily remain focal and their causative pathology may not be static necessitating a dynamic approach in both investigative as well as treatment measures. Therefore, multiple complementary investigative modalities such as scalp EEG plus intracranial EEG, fMRI plus intracranial EEG and MEG plus intracranial EEG may require to be combined in order to detect the epileptogenic network and tailor a successful epilepsy surgery.
In terms of novel biomarkers, the level of available evidence is highest for HFO followed by ISA, both of which expand the frequency spectrum and can be utilized to identify the epileptogenic focus as well as potentially analyze the epileptogenic network. New and upcoming biomarkers that analyze the epileptogenic network itself, such as EI and cortico-cortical evoked potentials; a novel low-frequency stimulation method used for brain mapping, are promising, but require more research evidence.
It is likely that some of these novel biomarkers will be incorporated into routine presurgical evaluation guidelines given that it is most likely that the gold standard biomarker of epileptogenicity will remain not just one but a combination of multiple complementary investigative modalities.
References
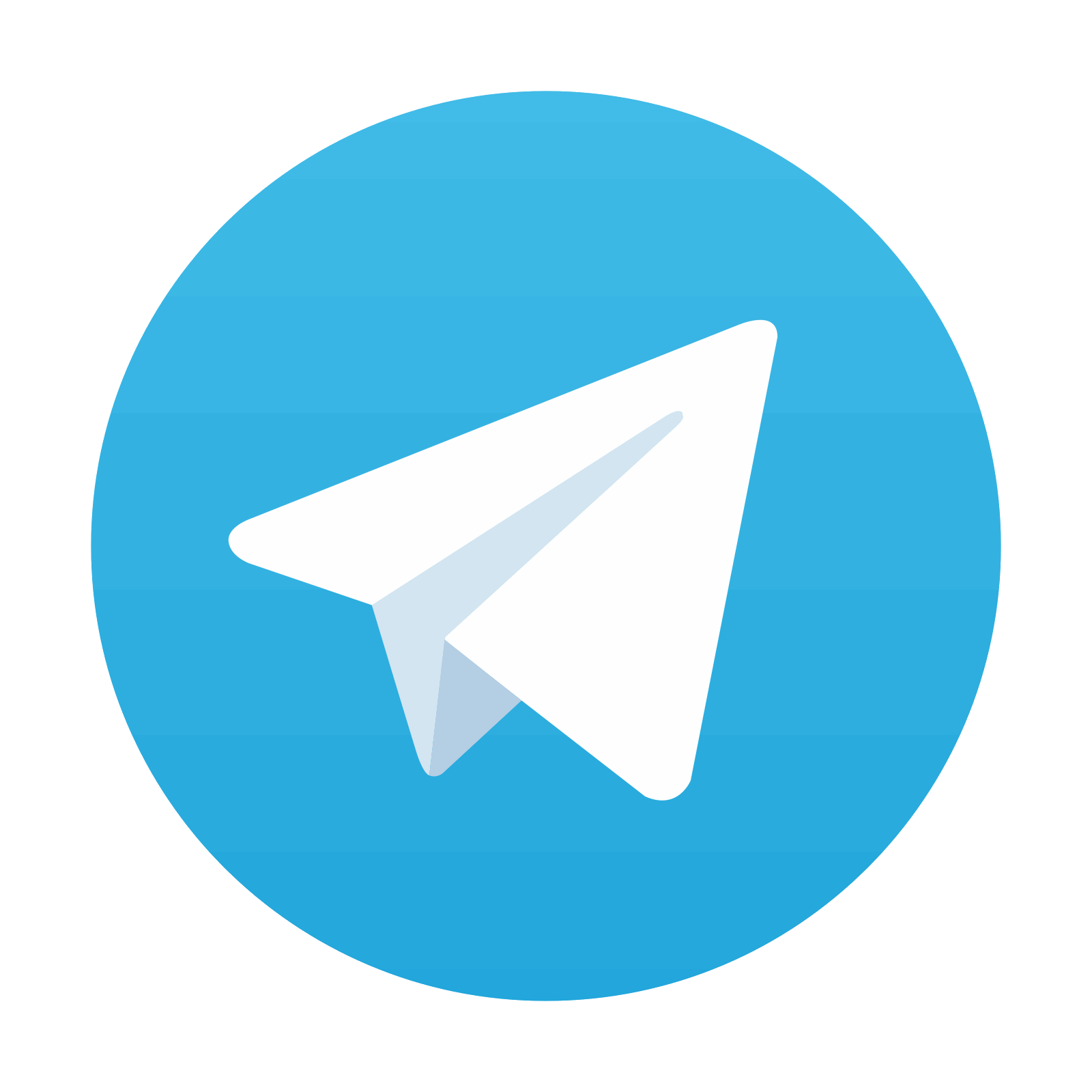
Stay updated, free articles. Join our Telegram channel

Full access? Get Clinical Tree
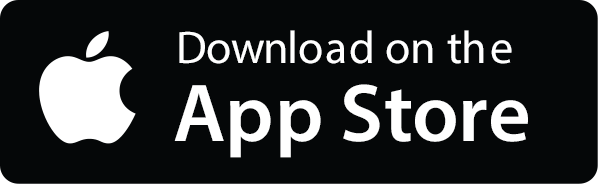
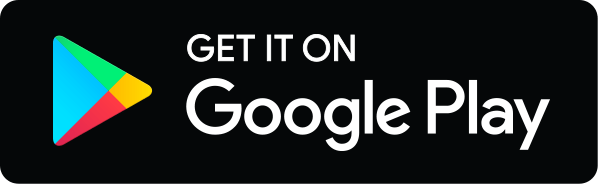
