, Jean Paul G. Vonsattel2, Helmut Heinsen3, 4 and Horst-Werner Korf5
(1)
Dr. Senckenbergisches Chronomedizinisches Institut, Goethe University Frankfurt, Frankfurt, Germany
(2)
Medical Center Neurological Institute, Columbia University, New York, NY, USA
(3)
Division Psychiatic Clinic Morphological Brain Research Unit, Julius Maximilians University Würzburg, Würzburg, Germany
(4)
University of Sao Paulo Medical School, Sao Paulo, Brazil
(5)
Dr. Senckenbergisches Chronomedizinisches Institut, Goethe University, Frankfurt, Frankfurt, Germany
6.1 Types of Eye Movements that Guarantee Stabilization of the Images of Objects of the Visual World Onto the Central Foveal Region of the Retina
The stabilization of the images of objects of the visual world onto the central foveal region of the retina enables a clear and stable view of our environment which is achieved by seven different types of eye movements (Table 6.1) (Leigh and Kennard 2004; Leigh and Zee 2006; Rüb et al. 2008b, 2009). The principal aim of these different types of eye movements is either to stabilize gaze to hold images steadily on the central foveal region of the retina or to shift gaze and bring images of objects of the visual world to the retina’s fovea independent of head movements. The eye movements are generated by distinct and widely separated oculomotor circuits, which involve a variety of premotor oculomotor brainstem nuclei and converge at the level of the cranial nerve nuclei concerned with eye movements (i.e., oculomotor, trochlear, and abducens nuclei). The oculomotor functions are traditionally subdivided on the basis of how they aid vision: saccades, smooth pursuits, vergence, vestibulo-ocular reflex, optokinetic nystagmus, fixation, and gaze holding (Table 6.1) (Büttner and Büttner-Ennever 2006; Büttner-Ennever and Horn 1997; Leigh and Kennard 2004; Leigh and Zee 2006; Rüb et al. 2008b, 2009).
Table 6.1
Types of eye movements generated by the human oculomotor system and their dysfunctions in Huntington’s disease (HD)
Type of eye movement | Normal function-dysfunctions in Huntington’s disease (HD) |
---|---|
Saccades | Fast conjugate eye movements that bring new images of objects of interest onto the central foveal region of the retina; saccades can be voluntary or present as fast phases of vestibulo-ocular reflex and optokinetic nystagmus |
Saccades in HD | Initiation deficits and slowing and restricted range of vertical and horizontal saccades; loss of vertical and horizontal saccades |
Smooth pursuits | Conjugate eye movements that track and hold the image of a small moving visual target on the fovea |
Smooth pursuits in HD | Velocity reduction and interruption by inappropriate saccades or square wave jerks |
Vergence | Disconjunctive eye movements toward (i.e., convergence) or away from each other (i.e., divergence) which direct the fovea of both eyes at a single visual object simultaneously and guarantee nearing of visual objects and stereoscopic vision |
Vergence in HD | Inability to converge |
Vestibulo-ocular reflex | Conjugate eye movements with a slow tracking phase and a rapid resetting phase which hold images of the seen world steady on the fovea during brief head rotations or translations |
Vestibulo-ocular reflex in HD | Reduced gain of the slow component |
Optokinetic nystagmus | Combined conjugate slow tracking pursuit movements and quick repositioning saccades which track and keep the images of large moving visual targets on the fovea |
Optokinetic nystagmus in HD | Reduced gain of the slow or rapid component; loss of optokinetic nystagmus |
Fixation | Keeps the image of stationary objects of the visual world on the fovea by minimizing ocular drifts and suppresses saccades that turn the fovea away from the object of interest |
Fixation in HD | Steady fixation impaired by unwanted saccades |
Gaze holding | Stabilizes images of objects of the visual world subsequent to gaze shifting and permits stable eye position between eye movements |
Gaze holding in HD | Vertical and horizontal gaze holding consistently intact (even in the late clinical HD stages) |
Saccades represent conjugate eye movements that bring new images of objects of interest onto the central foveal region of the retina and can be voluntary or present as fast phases of the vestibulo–ocular reflex and the optokinetic nystagmus (Table 6.1). In contrast, smooth pursuits are comparatively slow conjugate eye movements that track and hold the image of a small moving visual target on the fovea (Table 6.1). Vergence is accomplished by disconjunctive eye movements toward (i.e., convergence) or away from each other (i.e., divergence). These eye movements direct the fovea of both eyes to a single visual object simultaneously and thus guarantee nearing of visual objects and stereoscopic vision (Table 6.1). The vestibulo–ocular reflex involves conjugate eye movements with a slow tracking phase and a rapid resetting phase which hold images of the seen world steady on the fovea during brief head rotations or translations (Table 6.1). The optokinetic nystagmus comprises a combination of conjugate slow tracking pursuit movements and quick repositioning saccades that track and keep the images of large moving visual targets on the fovea (Table 6.1). During fixation the image of stationary objects of the visual world is kept on the fovea by minimizing ocular drifts and suppression of unwanted saccades that turn the fovea away from the object of interest (Table 6.1). Gaze holding guarantees stabilization of images of objects of the visual world subsequent to gaze shifting and permits stable eye position between eye movements (Table 6.1) (Büttner and Büttner-Ennever 2006; Büttner-Ennever and Horn 1997; Leigh and Kennard 2004; Leigh and Zee 2006; Rüb et al. 2008b, 2009).
6.2 Oculomotor Dysfunctions in Huntington’s Disease (HD)
Oculomotor dysfunctions are among the common and most impressive clinical features of HD and are widely used to establish the clinical diagnosis. In the advanced clinical stages affected HD patients may show a broad range of eye movement abnormalities, including impairments of saccades, smooth pursuits, vergence, vestibulo-ocular reaction, optokinetic nystagmus, and fixation (Table 6.1) (Beenen et al. 1986; Blekher et al. 2004, 2006; Bollen et al. 1986; Kennard and Lueck 1989; Lasker and Zee 1997; Leigh and Zee 2006; Leigh et al. 1983; Margolis and Ross 2003; Oepen and Ostertag 1981; Oepen et al. 1981, 1985; Rüb et al. 2009; Walker 2007a, b).
Impairments of saccades represent the most prominent and frequent symptoms in HD patients and manifest as problems with initiating, with slowing, or with a restricted range of horizontal and/or vertical saccades. Initiation deficits of vertical and horizontal saccades are very frequent disease signs of HD patients and may already be present in preclinical HD gene carriers and in the early clinical phase of HD. Owing to these initiation deficits, affected patients often can start saccades only with an associated head thrust or blink. In addition, the vertical and horizontal saccades of HD patients can also be slowed, hypo-, or hypermetric, restricted in range, or completely lost. In the majority of HD patients, slowed and range-limited saccades occur more frequently in the vertical than in the horizontal plane. As with deficits of saccadic initiation, slowed saccades may already be present in presymptomatic HD gene carriers and may emerge early during the course of HD (Table 6.1) (Avanzini et al. 1979; Beenen et al. 1986; Blekher et al. 2004, 2006; Bollen et al. 1986; Collewijn et al. 1988; Golding et al. 2006; Kirkwood et al. 2000; Lasker and Zee 1997; Lasker et al. 1987, 1988; Leigh et al. 1983; Oepen and Ostertag 1981; Oepen et al. 1981, 1985; Peltsch et al. 2008; Rüb et al. 2009; Winograd-Gurvich et al. 2003). Deficits of vertical and horizontal smooth pursuits emerge comparatively late during the course of HD and pertain predominantly to velocity reduction and an interruption by inappropriate saccades or square wave jerks (Table 6.1) (Avanzini et al. 1979; Beenen et al. 1986; Collewijn et al. 1988; Kennard and Lueck 1989; Lasker and Zee 1997; Leigh and Zee 2006; Leigh et al. 1983; Rüb et al. 2009; Starr 1967; Winograd-Gurvich et al. 2003). Some HD patients may also show an inability to converge, a reduced gain of the slow component of the vestibulo–ocular reaction, as well as impairments of the optokinetic nystagmus (i.e., reduced gain of the slow or rapid component, loss of the optokinetic nystagmus) (Table 6.1) (Beenen et al. 1986; Blekher et al. 2004, 2006; Fielding et al. 2004; Golding et al. 2006; Kennard and Lueck 1989; Kirkwood et al. 2000; Lasker and Zee 1997; Leigh and Zee 2006; Leigh et al. 1983; Leopold et al. 1982; Oepen et al. 1981, 1985; Rüb et al. 2009; Starr 1967). Impaired steady fixation is also a frequent, early, and characteristic oculomotor dysfunction of HD patients, whereby affected patients are unable to suppress unwanted saccades during fixation and therefore cannot maintain steady fixation (Table 6.1) (Blekher et al. 2004, 2006; Collewijn et al. 1988; Kennard and Lueck 1989; Lasker and Zee 1997; Leigh and Zee 2006; Leigh et al. 1983; Peltsch et al. 2008; Rüb et al. 2009). In contrast to the six other types of eye movements, vertical and horizontal gaze holding is consistently intact in HD even in its late clinical stages (Table 6.1) (Rüb et al. 2009).
The occurrence of the oculomotor symptoms also pointed to an involvement of the premotor oculomotor brainstem network in HD and underscored the necessity of detailed brainstem studies (Bruyn et al. 1979; Koeppen 1989; Kremer et al. 1992; Lasker and Zee 1997; Leigh et al. 1983, 1985; Rüb et al. 2009; Vonsattel 2008; Vonsattel and DiFiglia 1998; Vonsattel et al. 1985). However no systematic analysis of this complex functional system could yet be performed in the brains of HD patients. Thus, the exact contribution of the nuclei of this network to the pathogenesis of oculomotor symptoms in HD remained unclear for many decades rendering our knowledge regarding the spectrum of morphological brain alterations underlying the various oculomotor impairments of HD patient fragmentary (Rüb et al. 2009).
6.3 The Human Premotor Oculomotor Brainstem Network
The human premotor oculomotor brainstem network (1) involves neural circuits subserving the generation of saccades, smooth pursuits, vergence, the vestibulo-ocular reflex, optokinetic nystagmus, fixation, as well as gaze holding, (2) generates and modifies different physiological features of slow and rapid eye movements (i.e., initiation, velocity, accuracy), and (3) steers the three oculomotor cranial nerve nuclei (i.e., oculomotor, trochlear, and abducens nuclei) by means of direct or indirect projections (Büttner and Büttner-Ennever 2006; Büttner-Ennever and Horn 1997; Rüb et al. 2008a, 2009).
Except that subserving vergence, much has been learned in the last two decades regarding the functional neuroanatomy of the neural circuits crucial for the generation of saccades, smooth pursuits, the vestibulo-ocular reflex, and optokinetic nystagmus, as well as gaze holding. The oculomotor circuits are widely separated structurally, generate different types of eye movements relatively independently, involve various premotor oculomotor brainstem nuclei, and converge only at the level of the motoneurons of the oculomotor cranial nerve nuclei (Büttner and Büttner-Ennever 2006; Büttner-Ennever and Horn 1997; Rüb et al. 2008b,2009). The combined application of cytoarchitectonical criteria, histochemical and immunocytochemical techniques, as well as advanced tracing methods enabled the identification of a number of important premotor oculomotor nuclei in the brainstem of nonhuman primates and humans. Prominent examples for these nuclei are the rostral interstitial nucleus of the medial longitudinal fascicle (riMLF), the area of excitatory burst neurons for horizontal saccades (EBN), the raphe interpositus nucleus (RIP) of the paramedian pontine reticular formation, as well as the dorsal paragigantocellular reticular nucleus (DPGI) (Figs. 6.1, 6.2, and 6.3) (Büttner and Büttner-Ennever 2006; Büttner-Ennever and Horn 2004; Büttner-Ennever et al. 1982, 1988; Horn 2006; Horn and Büttner-Ennever 1998; Horn et al. 1994, 1995; Rüb et al. 2008b, 2009).
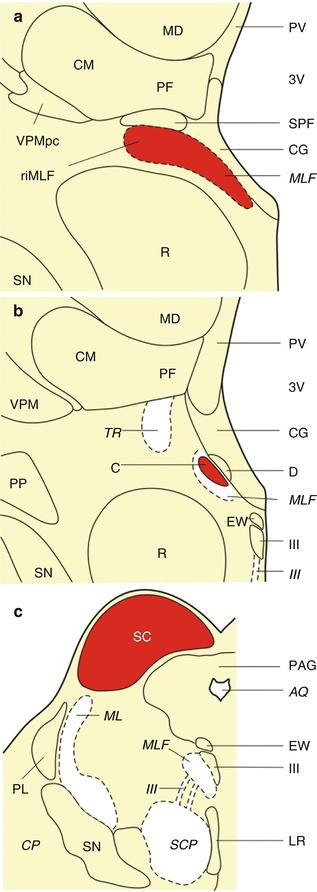
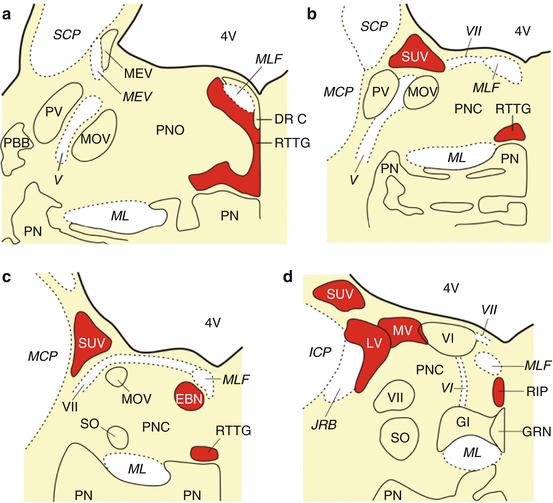
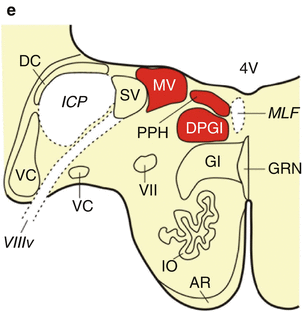
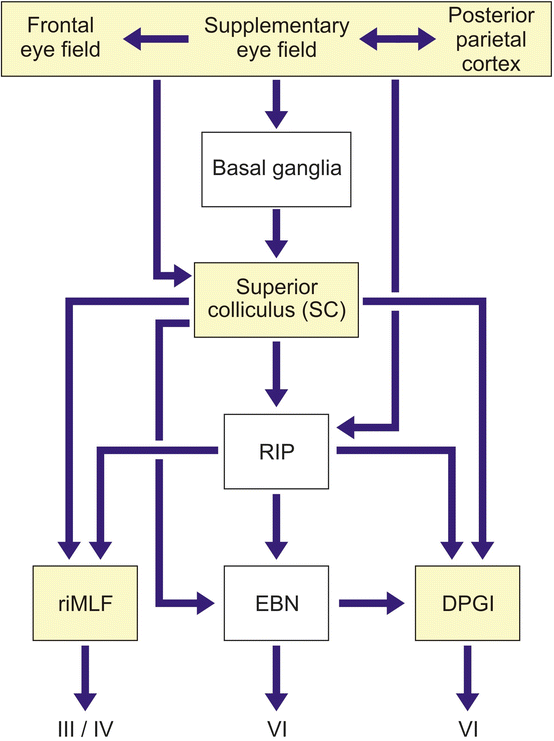
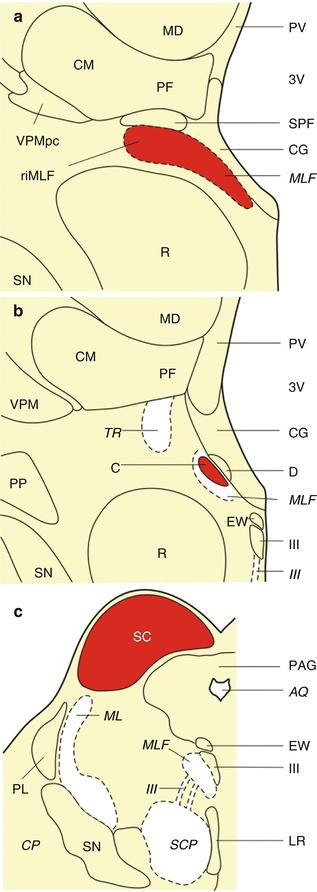
Fig. 6.1
The premotor oculomotor nuclei of the human midbrain. (a) Frontal section cut perpendicularly to the intercommissural axis of Forel showing the rostral midbrain with the medial longitudinal fascicle (MLF) and the rostral interstitial nucleus of the medial longitudinal fascicle (riMLF). (b) Frontal section cut perpendicularly to the intercommissural axis of Forel showing the rostral midbrain with the MLF and the interstitial nucleus of Cajal (C). (c) Schematized horizontal section through the caudal midbrain with the superior colliculus (SC) (Modified according to Rüb et al. (2008b), (Figure 1, page 170); with kind permission from Springer Science and Business Media). Abbreviations: AQ aqueduct, C interstitial nucleus of Cajal, CG central gray, CM centromedian nucleus of the thalamus, CP cerebral peduncle, D nucleus of Darkschewitsch, EW Edinger-Westphal nucleus, LR linear raphe nucleus, MD mediodorsal nucleus of the thalamus, ML medial lemniscus, MLF medial longitudinal fascicle, PAG periaqueductal gray, PF parafascicular nucleus of the thalamus, PL paralemniscal nucleus, PP peripeduncular nucleus, PV paraventricular nucleus of the thalamus, R red nucleus, riMLF rostral interstitial nucleus of the medial longitudinal fascicle, SC superior colliculus, SCP superior cerebellar peduncle, SN substantia nigra, SPF subparafascicular nucleus of the thalamus, TR tractus retroflexus (habenulo-interpeduncular tract), VPM ventral posterior medial nucleus of the thalamus, VPMpc ventral posterior medial nucleus of the thalamus, parvocellular part, III oculomotor nucleus, III oculomotor nerve, 3V third ventricle
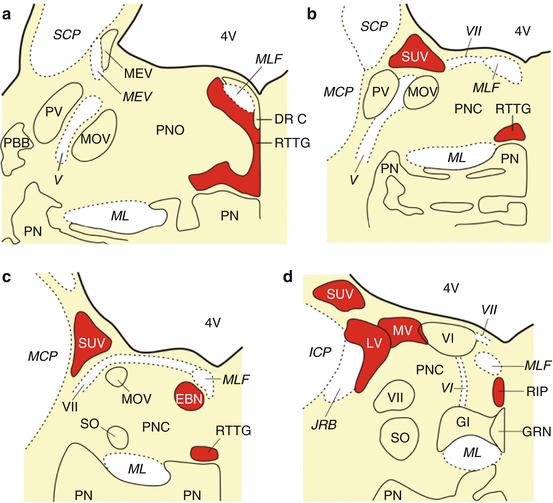
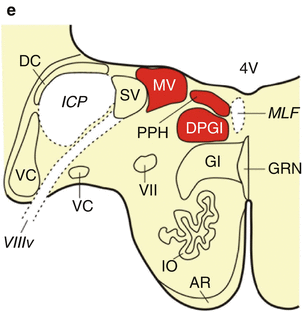
Fig. 6.2
The premotor oculomotor nuclei of the human pons and medulla oblongata. (a) Schematized horizontal section through the mid-level of the pons with the reticulotegmental nucleus of the pons (RTTG; nucleus Bechterew) and the medial longitudinal fascicle (MLF). (b) Schematized horizontal section through the mid-level of the pons, superior vestibular nucleus (SUV), caudal portion of the RTTG, and MLF. (c) Horizontal section through the caudal pons showing the area of the excitatory burst neurons for horizontal saccades (EBN), the caudal nucleus of the pontine reticular formation (PNC), the SUV and the most caudal portion of the RTTG. (d) The caudal pons with the superior (SUV), lateral (LV), and medial vestibular nuclei (MV), the abducens nucleus (VI) and nerve (VI), the raphe interpositus nucleus (RIP), and the MLF. (e) Horizontal section through the pontomedullary junction with the prepositus hypoglossal nucleus (PPH), dorsal paragigantocellular reticular nucleus (DPGI), MV, and the MLF (Modified according to Rüb et al. (2008b), (Figure 2, page 172); with kind permission from Springer Science and Business Media). Abbreviations: AR arcuate nucleus, DC dorsal cochlear nucleus, DPGI dorsal paragigantocellular reticular nucleus, DR C dorsal raphe nucleus, caudal part, EBN area of the excitatory burst neurons for horizontal saccades, GI gigantocellular reticular nucleus, GRN great raphe nucleus, ICP inferior cerebellar peduncle, IO inferior olive, JRB juxtarestiform body, LV lateral vestibular nucleus, MCP medial cerebellar peduncle, MEV mesencephalic trigeminal nucleus, MEV mesencephalic trigeminal tract, ML medial lemniscus, MLF medial longitudinal fascicle, MOV motor trigeminal nucleus, MV medial vestibular nucleus, PBB pontobulbar body, PN pontine nuclei, PNC pontine reticular formation, caudal nucleus, PNO pontine reticular formation, oral nucleus, PPH prepositus hypoglossal nucleus, PV principal trigeminal nucleus, RIP raphe interpositus nucleus, RTTG reticulotegmental nucleus of the pons (nucleus Bechterew), SCP superior cerebellar peduncle, SO superior olive, SUV superior vestibular nucleus, SV spinal vestibular nucleus, VC ventral cochlear nucleus, 4V fourth ventricle, V trigeminal nerve, VI abducens nucleus, VI abducens nerve, VII facial nucleus, VII facial nerve, VIIIv vestibulocochlear nerve, vestibular portion
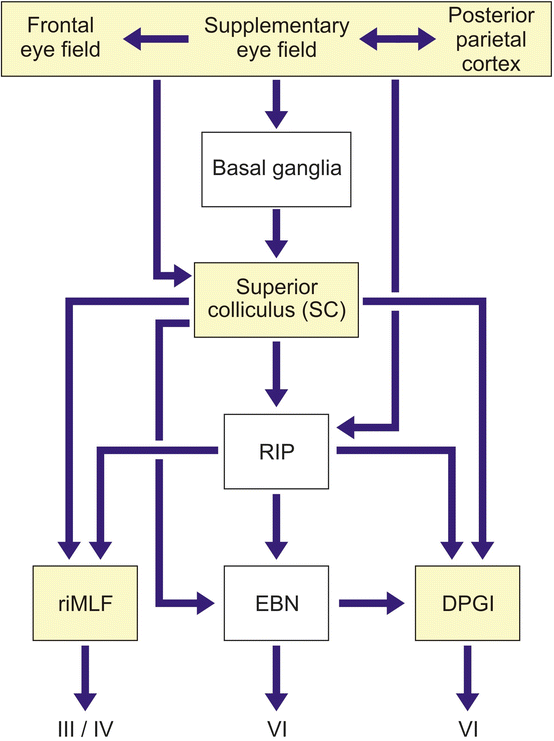
Fig. 6.3
Simplified block diagram of the neural network that generates horizontal and vertical saccades. Saccades are generated by two types of premotor brainstem nerve cells: (1) burst neurons, which deliver high-frequency bursts of activity to the motoneurons of involved external eye muscles before saccades and during saccades, and (2) pause neurons, which exert a tonic inhibition on brainstem burst neurons. The ongoing activity of these pause neurons is inhibited before and during saccades in a specific direction (directional pause neurons) or in all directions (omnipause neurons). In humans, the excitatory burst neurons for horizontal saccades are located in a subnucleus of the caudal portion of the pontine reticular formation (i.e., area of the excitatory burst neurons for horizontal saccades, EBN) and the excitatory burst neurons for vertical saccades in the midbrain rostral interstitial nucleus of the medial longitudinal fascicle (riMLF). The inhibitory burst neurons for horizontal saccades reside in the dorsal paragigantocellular reticular nucleus (DPGI). These inhibitory DPGI nerve cells are activated by the burst neurons of the EBN and inhibit the contralateral abducens nucleus (VI) during horizontal saccades. Located in the pontomedullary raphe interpositus nucleus (RIP), the saccadic omnipause neurons fire with a high ongoing rate before and during saccades. The pause of the ongoing firing of these omnipause neurons in the RIP allows the burst neurons in the EBN and riMLF to activate the external eye muscle motoneurons in the oculomotor cranial nerve nuclei (i.e., oculomotor, trochlear, and abducens nuclei, III, IV, VI). The omnipause neurons in the RIP send inhibitory projections to the premotor burst neurons in the EBN and riMLF and excitatory efferents to the inhibitory burst neurons of the DPGI. While the EBN, riMLF, and DPGI receive excitatory projections from the superior colliculus (SC), the efferent projections from the SC to the saccadic omnipause neurons in the RIP inhibit their tonic firing activity before and during saccades in all directions. Projections from the frontal and supplementary eye fields supply the omnipause neurons in the RIP with cortical information. The subcortical sites that until now have been shown to sustain neuronal loss in Huntington’s disease (HD) are depicted in white (Modified according to Rüb et al. (2003a), (Figure 1, page 117); with kind permission from Elsevier). Abbreviations: DPGI dorsal paragigantocellular reticular nucleus, EBN area of the excitatory burst neurons for horizontal saccades, riMLF rostral interstitial nucleus of the medical longitudinal fascicle, RIP raphe interpositus nucleus, III oculomotor nucleus, IV trochlear nucleus, VI abducens nucleus
The human premotor oculomotor brainstem nuclei are located in the midbrain and pontine tegmentum. They are intimately associated with the medial longitudinal fascicle (MLF) and represent comparatively small gray components with distinctive cytoarchitectonical features (Figs. 6.1, 6.2, and 6.3). Like other human motor or premotor brainstem nuclei, their nerve cells typically contain only small amounts of cytoplasmic lipofuscin granules. These characteristic cyto- and pigmentoarchitectonical features enable the straightforward and reliable identification of the human premotor oculomotor brainstem nuclei in unconventional thick serial tissue sections stained for neuronal Nissl material and lipofuscin pigment (pigment-Nissl staining according to Braak) (Figs. 6.4 and 6.5). This technical approach is therefore well suited to complement and to replace more labor-intensive and expensive histochemical or immunocytochemical staining methods in the field of the anatomy and pathoanatomy of the human oculomotor system (Braak et al. 2003b; Rüb et al. 2001, 2003a, 2004a, b, 2008a, b, 2009, 2013b; Scherzed et al. 2012). Owing to their optical superposition, unconventional thick serial brain tissue sections display considerably more nerve cells than the conventional thin sections routinely used by neuropathologists and therefore facilitate the identification of discrete, even minute neuroanatomical components such as the premotor oculomotor brainstem nuclei. The combined staining for neuronal Nissl material (with Darrow red) and lipofuscin pigment (with aldehyde-fuchsin) takes advantage of pigmentoarchitectonical information to facilitate detailed anatomical analyses as well as the evaluation of the exact topographic localization of neurodegeneration. Aldehyde-fuchsin stains neuronal cytoplasmic lipofuscin granules prominently, which are structurally stable and can be identified even after delayed fixation. Since their extraneuronal presence indicates the previous position of lost neurons, the presence of these granules in the brain neuropil can serve as a marker for the demise of lipofuscin-containing nerve cells (Braak et al. 2003b; Rüb et al. 2001, 2003a, 2004a, b, 2008a, b, 2009, 2013b; Scherzed et al. 2012).
