Embryology, Development, and
Classification of Disorders of the
Craniovertebral Junction
The term craniovertebral junction (CVJ) refers to the occipital bone that surrounds the foramen magnum, the atlas, and the axis vertebrae. This bony enclosure encompasses the medulla oblongata, the cervicomedullary junction, and the upper cervical spinal cord. Bony abnormalities that affect this complex can result in neural compression along the entire circumference, vascular compromise, and abnormal cerebrospinal fluid (CSF) dynamics.1,2 The basis for understanding these problems comes from knowledge of the embryology, bony anatomy, and the biomechanics of this region. This chapter discusses the embryology and the normal and abnormal development of the CVJ and the traditional classification of disorders affecting the craniocervical area.3 Since 1977, the author has investigated 6000 patients with neurological symptoms and signs related to abnormalities of the craniocervical region.4 A surgical-physiological approach to treatment of the abnormalities is the basis for a practical classification of craniocervical region abnormalities.5
Embryology and Development of the Craniovertebral Junction
Congenital anomalies of the CVJ frequently occur with various combinations of neural abnormalities, suggesting an interrelationship, if not a common cause, of its origin and development.6 A review of the embryology, outlining the developmental sequence and timing of events in this region, follows here.
A definite notochord forms between the ectoderm and the endoderm during the third week of gestation. At the cephalic end of the embryo, the ectoderm immediately overlying the notochord begins to thicken and differentiates into neural ectoderm, which forms the neural plate. This plate begins to buckle along the middle to shape the neural groove, which becomes walled in by the neural folds on each side. While this is occurring, a collection of mesenchymal cells begins to coalesce in three regions. The most medial becomes a solid mass—the paraxial mesoderm—which is just lateral to the notochord and on either side of it. This precursor of bone, skeletal muscle, and skin begins to segment in the cranial and caudal directions.7–9 The parachordal mesodermal cells migrate to the midline and fuse around the notochord at the level of the jugular foramen.10
The bony cranial base is developed by the process of endochondral ossification in which a cartilaginous framework is first developed and subsequently resorbed with further deposition of bone, based on distorting forces such as brain and eye development.11–13 Suture growth also appears to play a fairly major role. The clivus is elongated by sutural growth of the spheno-occipital synchondrosis and by further sutural growth along the lateral portion of the base. Björk described two sutural growth sites, the petro-occipital and the sphenopetrosal junctions, which result in marked lowering of the occiput and foramen magnum.11 Thus, the combination of sutural growth and bony accretion is necessary for development in the cranial base.
The majority of the skull and facial bones—including the mandible, maxilla, premaxilla, zygoma, frontal, parietal, vomer, palatine, and nasal bones—develop by intramembranous ossification.14 This development of intramembranous ossification bypasses the intermediate cartilaginous stage characteristic of the development of the bony cranial base.
During the fourth week of gestation, 42 somites are formed: 4 occipital somites, 8 cervical, 12 thoracic, 5 lumbar, 5 sacral, and 8 to 10 coccygeal pairs. Each somite differentiates into an outer dermatome, an inner myotome, and a medial sclerotome. The sclerotomes are ventromedial in location and destined to form the vertebral bodies. These bilateral ventromedial cells migrate to the midline and surround the notochord. Each sclerotome develops a central cleft called the fissure of von Ebner, which divides a loose collection of cells cranially from a dense cellular area caudally. In the development of the normal spine, cells from the fissure of von Ebner migrate to and encase the notochord to become precursors of the intervertebral disk.12
The superior half of one sclerotome (caudal) unites with the lower half of its neighbor (cranial) and thus forms the earliest manifestation of a vertebral body. The first four sclerotomes do not follow this course and eventually fuse to form the occipital bone and the posterior portion of the foramen magnum.15,16 Vascularization of the occipital bone begins at this time, and differentiation of the ganglia and vascular tissue continues. The hypoglossal and first cervical arteries, although inconspicuous, are present and demarcate the caudal occipital segment.17
Simultaneous with this development, there is differentiation of the nervous system process. A tube-like appearance is seen by the end of the third week of gestation, and during the fourth week the neural fold that forms the side wall starts to fuse in the midline to form a tube. This occurs in the region of the future atlanto-occipital junction and corresponds to the fourth somite.18,19 The fusion of the neural tube proceeds simultaneously in a rostral and caudal dimension with both ends remaining open. During the fifth and sixth weeks, further differentiation of the various parts of the brain and spinal cord occurs.20 However, the roof of the fourth ventricle thins out in the midline to form the foramen of Magendie and, laterally, the foramen of Luschka. This occurs because an opening forms in the seventh week when a connection between the fourth ventricle and the subarachnoid space is established.
Membrane formation is usually over by 38 to 40 days of gestation. Cartilage formation then begins with chondrification in the basioccipital bone.13
The occipital sclerotomes correspond to the segmental nerves that group to form the hypoglossal nerve, with a path through the individual foramina in the bone.21 The first two occipital sclerotomes ultimately form the basiocciput ( Table 1.1 ). The third sclerotome is responsible for the exoccipital center as it forms the jugular tubercles. The key to understanding the CVJ embryology is the proatlas, which is the fourth occipital sclerotome ( Fig. 1.1 ).15,22–24 The hypocentrum of the fourth occipital sclerotome forms the anterior tubercle of the clivus. The centrum of the proatlas forms the apical cap of the dens as well as the apical ligament.16,17 The neural arch component of the proatlas divides into a rostral ventral component and a caudal dorsal portion. The ventral portion forms the U-shaped anterior margin of the foramen magnum as well as the occipital condyles and the midline third occipital condyle. The cruciate ligament and the alar ligaments are condensations of the lateral portion of the proatlas. The caudal division of the neural arch of the proatlas forms the lateral atlantal masses of C1, as well as the superior portion of the posterior arch of the atlas.
The atlas vertebra is formed by the first spinal sclerotome—a transitional vertebra modified from the remaining spinal vertebra. The centrum is separated to fuse with the axis body forming the odontoid process. At an early stage, a hypochordal bow is found in front of each vertebral segment but subsequently disappears, except for the part that forms the anterior arch of the atlas.7 The neural arch of the first spinal sclerotome forms the posterior and inferior portions of the atlas arch. The hypochordal bow of the proatlas itself may survive and join with the anterior arch of the atlas to form a variant, such that an abnormal articulation may exist between the clivus, the anterior arch of the atlas, and the apical segment of the odontoid process.25
During embryogenesis, the hypocentrum of the second spinal sclerotome disappears. The centrum forms the body of the axis vertebra, and the neural arches develop into the facets and the posterior arch of the axis. Thus, the body of the dens appears from the first sclerotome, whereas the terminal portion of the odontoid process arises from the proatlas.15 The most inferior portion of the axis body is formed by the second spinal sclerotome. At birth, the odontoid process is separated from the body of the axis vertebra by a cartilaginous band that represents the vestigial disk, referred to as a neural central synchondrosis. It lies below the level of the superior articular facets of the axis and does not represent the anatomical base of the dens.26 The neural central synchondrosis is present in most children younger than 3 to 4 years old but disappears by age 8. At birth, there should be a recognizable odontoid process that does not fuse to the base of the axis. The tip of the odontoid process is not ossified at birth; hence, it is not seen on the lateral radiograph. It is represented by a separate ossification center, which usually is seen at age 3 and fuses with the remainder of the dens by age 12.27


Expansion in the posterior fossa occurs as a result of a combination of endocranial resorption, sutural growth, and bony accretion.14 The growth of the basion elongates the basiocciput and lowers the frontal margin of the foramen magnum. There is a comparably matched resorptive drift downward and backward at the opisthion as a result of the cerebellar downward displacement with the rotation of the occipital and temporal lobes of the brain. Thus, the posterior fossa apart from the midline basiocciput relies fairly heavily on endocranial resorption. However, synchondrodial growth is also an important feature in posterior fossa expansion.11 The sagittal elongation in the spheno-occipital synchondrosis is active into the second decade of life. The fact that the basal angle of the cranial base appears to remain relatively constant throughout life is probably secondary to the mutual interaction between synchondrodial growth at the spheno-occipital synchondrosis and the constant surface remodeling that occurs. Growth in the skull base is interesting in that it coordinates with both brain development and development elsewhere in the body. It has been noted that there are pubertal spurts in the basal growth and that the rostrocaudal orientation parallels the growth in the brain hemispheres. However, despite this gradient of growth in the cranial base, the basicranial inclination of ~130 degrees is maintained throughout postnatal life. This angulation distinctly separates the expanding brain above and the facial complex and nasopharyngeal spaces below. The stability of the craniovertebral articulation with the forward inclination of the top-heavy cranial end of the fetus must then be maintained by the geometry of the articular surfaces of the CVJ, as well as by the ligamentous attachments and, more importantly, by the heavy development of the dorsal and lateral cervical musculature, which provides a clamping action on the craniocervical region.28,29
Implications
A wide variety of congenital anomalies of the craniocervical region exists, occurring singularly or as more than one anomaly in the same individual and involving osseous and neural structures.6,7,30 An insult to both may occur between the fourth and seventh weeks of intrauterine life and may result in a combination of anomalies. A careful look at the embryology and development of the craniocervical region makes it apparent that anomalies in this region would consist of failures of segmentation, failures of fusion of different components of each bone, or hypoplasia and ankylosis.4,22,31–39

Understanding the growth of the cranial base and CVJ forms the basis for understanding a variety of anomalous processes: abnormalities of atlas assimilation, occipitalization of the atlas, remnants of the occipital sclerotomes and fusion abnormalities, abnormal fusion between the occiput-C1 and C2, os odontoideum, ossiculum terminale, trauma to the craniovertebral complex in both children and adults, and secondary forms of invagination.2,39
It is important to differentiate between an occipital vertebra and atlas assimilation. An occipital vertebra occurs when the third occipital sclerotome fails to be incorporated into the rostral two occipital sclerotomes.39,40 In this situation, the occipital condyles attach to the vertebra. If the occipital vertebra contains a transverse process, it does not have a foramen for the vertebral artery. In atlas assimilation (sometimes called occipital-atlantal fusion), the transverse process of the atlas bears the bony foramen for the vertebral artery.41 Segmentation fails between the fourth occipital sclerotome and the first spinal sclerotome ( Fig. 1.2 ). Thirty-eight percent of individuals with symptomatic atlas assimilation and segmentation failures of C2 and C3 vertebrae were found to have a Chiari malformation.4,7 A paramesial invagination, reducible atlantoaxial dislocation, or basilar invagination may exist ( Figs. 1.3 and 1.4 ).


Os odontoideum was previously considered to be congenital and was described as a failure of fusion between the centrum component of C1 and that of C2.24 However, the radiographic abnormality always has a hypoplastic dens and the spheno-occipital synchondrosis is a definite visible entity. Thus, os odontoideum cannot be congenital.26 To be congenital, the defect would have to be below the superior slopes of the C2 facets.27 Os odontoideum most likely originates as odontoid trauma occurring between ages 1 and 4, with subsequent separation and distraction of the odontoid fragments. The superior segment is distracted upward by the apical and alar ligaments and receives its blood supply from the descending branch of the occipital artery, which courses along the apical ligament.26,27,42 Thus, there is a hypertrophied, rounded ossicle causing the hypoplastic dens as well as hypertrophy of the anterior arch of the atlas, leading to incompetence of the cruciate ligament and further abnormalities. Aplasia or hypoplasia of the dens is an uncommon feature but has been described ( Fig. 1.5A,B ).
Due to the high fulcrum of neck motion, spinal trauma in children younger than age 8 is mainly at the craniovertebral border,2 resulting in ligamentous injuries more than fractures. However, odontoid fractures in this age group are usually seen as avulsion injuries with separation of the neural central synchondrosis.
Anomalies and malformations of the caudal occipital sclerotomes are collectively called manifestations of an occipital vertebra and result in abnormal bony ridges and overgrowths of the ventral aspect of the foramen magnum.15,39 At times, segmentation abnormalities of the clivus are recognized and represent a failure of the proatlas to separate from the basiocciput of the clivus. Consequently, bone growth in the anterior aspect of the foramen magnum indents the ventral cervicomedullary junction ( Fig. 1.6 ).7 A third occipital condyle can present in the mid-line and is called median occipital condyle ( Fig. 1.7 ).43–45 Bilateral paramesial compression from hypertrophied occipital condyles represents the ventrorostral neural arch of the proatlas ( Fig. 1.8 ).15,46

It must be kept in mind that, during development, the occipital sclerotome is functionally a vertebra.21 In 1981, Marin-Padilla and Marin-Padilla demonstrated that the basichondrocranium of fetuses with hindbrain malformations, such as Chiari syndrome, is shorter than normal and elevated (lordotic) in relation to the axis of the vertebral column.47 The shortness of the basichondrocranium of these fetuses is attributed to underdevelopment of the occipital bone, especially noticeable in its basal component. The basic defect supposedly results in a short and small posterior fossa, inadequate to contain the developing nerve structures of the region. The authors theorize that the developing cerebellum is displaced downward to an anomalous position just above the foramen magnum, and the developing medulla is compressed and crowded into a small posterior fossa. This lordotic elevation of the basichondrocranium is responsible for the reduction of the pontine flexure and the increased angle of the cervical flexure of the hindbrain in these fetuses. The elongation of the odontoid process could be explained by the depression of the basiocciput, resulting in the basilar impression often seen in clinical Chiari malformations. These changes have been experimentally reproduced in pregnant hamsters by a single dose of vitamin A on the morning of the eighth day of gestation, thus inducing a typical Chiari I and Chiari II malformation as well as various types of axial skeletal-dysraphic disorders known to be associated with the human disease.48,49



The ongoing growth of the posterior fossa from birth to later adolescence provides an understanding of the continued need to observe children who have undergone dorsal occipitocervical stabilization or a ventral craniovertebral decompression.31 The downward growth of the brain, as well as the elongation of the posterior fossa and clivus, may re-create a ventral bony abnormality later in life despite a previous ventral decompression at the CVJ that might have been performed during the first two decades of life. Similarly, patients with known atlas assimilation and basilar invagination tend to become symptomatic toward the end of their first and second decade of life due to developmental changes in the size, structure, and configuration of the foramen magnum.4,50,51 Basilar impression is due to bone softening states, such as osteogenesis imperfecta, Paget disease, and hyperparathyroidism.4 The skull base infolds, and the spine ascends ( Fig. 1.9 ).

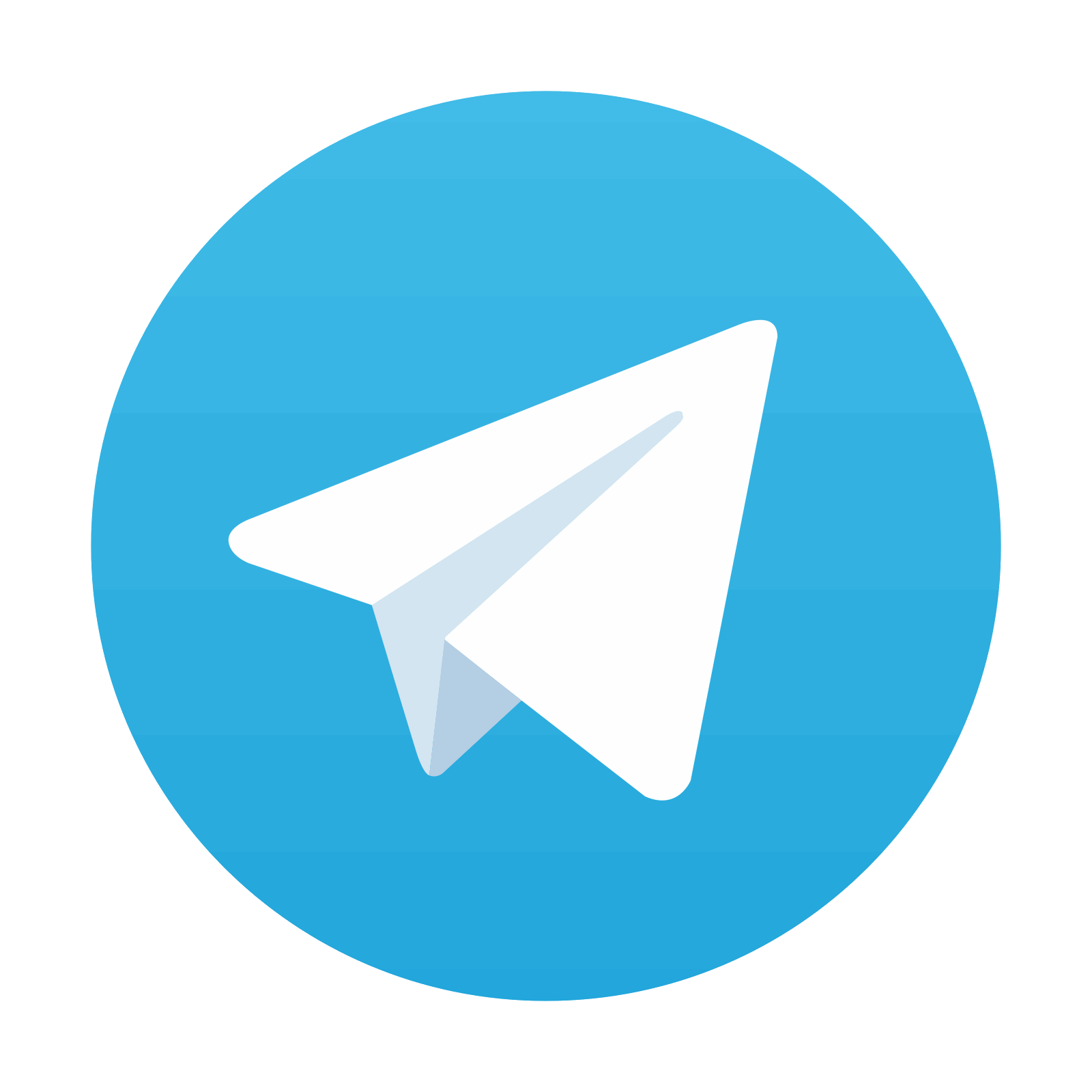
Stay updated, free articles. Join our Telegram channel

Full access? Get Clinical Tree
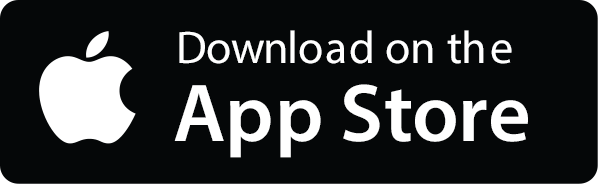
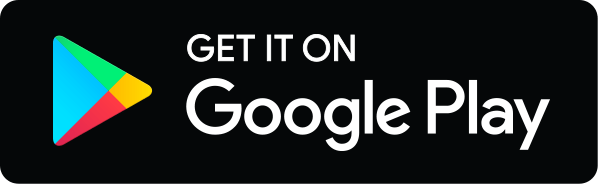
