CHAPTER 62 Emerging Surgical Techniques for the Treatment of Meningiomas
INTRAOPERATIVE IMAGING
Image-guided neurosurgery using a navigation system in the traditional operating room is important in the management of many meningiomas because it can localize the tumor and critical structures around it. Intraoperative imaging is a useful further development because it compensates for brain shift, allows accurate navigation, and gives verification of what has been done surgically before the patient leaves the operating room.1 Current intraoperative imaging modalities offering real-time imaging are computed tomography (CT), magnetic resonance imaging (MRI), and ultrasound. Other modalities may help define the tumor by cellular techniques—these include photodynamic imaging, radio immune imaging, and infrared imaging with ala or other material. These extend the capacity of the surgeon significantly but are not discussed here.
Brain shift may result from fluid shifts, reduction in tumor volume as the tumor is resected, changes in arterial pCO2, and cerebrospinal fluid (CSF) loss after opening of the dura.2–5 It makes guidance from preoperative images potentially and variably inaccurate. Intraoperative brain surface deformations greater than 10 mm have been documented within 1 hour of opening the dura. The error induced by this type of shift may be even larger in the presence of hydrocephalus or preexisting loss of parenchymal volume. Intra-operative imaging was in part created to overcome the effect of brain shifting.
CT in Intraoperative Imaging
CT has good tissue resolution but has traditionally been limited to uniplanar imaging and has the disadvantage of radiation of the patient and surgical team. As post-processing becomes more sophisticated and as radiation doses lessen, these may become less important. Lunsford and colleagues have extensive experience with CT in guiding tumor resection.6
Intraoperative Ultrasonography
Ultrasound is less expensive and less bulky than CT or MRI. It guides operative neurosurgery by differences in physical properties of the tumor and may show vasculature, which is quite helpful in larger meningiomas. Images may be difficult to interpret because echogenic structures may not reliably discern normal from abnormal tissue, and air bubbles or blood products in the surgical field may cause misinterpretation of ultrasound images.7–9 It is a potentially important modality, however, for working within the surgical field once it is exposed, and its importance for assessing residual tumor and vascularity in skull-base meningiomas has not been evaluated. As new three-dimensional systems become available, this may be the most widely available and useful technique for intraoperative imaging.7
Intraoperative MRI
Intraoperative MRI allows multiplanar imaging of changes during surgery, accurate navigation, immediate assessment of such complications as hemorrhage, and verification of the planned resection. The first system was the GE Signa 0.5 tesla “double doughnut” installed at Brigham & Women?’s Hospital (BWH) in 1994.1 The first brain tumor extirpation guided by intraoperative magnetic resonance was in 1996 at BWH. Multiplanar imaging, tissue discrimination, and absence of ionizing radiation have all contributed to the development of MRI as a tool for guiding interventional procedures, and there are more than 50 installations worldwide at the time of writing of this chapter.8–18 Imaging sequences such as MR spectroscopy, functional MR, diffusion-weighted imaging, perfusion weighted imaging, MR angiography, and MR venography, are all possible with high-field systems and can significantly influence the decision-making process of the neurosurgeon.
Factors contributing to high MR image quality for diagnostic purposes include the system field strength, field homogeneity, and stability of the static and gradient magnetic fields. The optimal design of a magnet with regard to field homogeneity would be a complete sphere, without opening. However, access to the patient is a prerequisite for surgery and monitoring. Design of intraoperative MR systems have advanced through compromises between opposing forces of field strength and surgical convenience and several systems have emerged from the close collaboration among surgeons, engineers, and industry. It is beyond the scope of this chapter to analyze the different intraoperative MR systems currently in use, and the reader is referred to descriptions in the recent literature. There are roughly two axes of interest; field strength, and movement of the patient. One classification is based on the magnet’s field strength; magnets can be described as low-field (0.12–0.15T), mid-field (0.3–0.5T), and high-field (1.5–3T). At present, low-field and mid-field systems have the advantage of providing greater patient access. High-field (1.5–3T) systems have restricted access to the patient during scanning, but offer higher resolution imaging. Examples of low-field systems are the GE Signa, the Hitachi 0.3 tesla system, and the Polestar 1.5 tesla system. These provide very comfortable patient access but imaging is not high resolution. In contrast, the high-field systems—Siemens Brain Suite, IMRIS system, and the Philips systems have more involved patient access.11–17
A second parameter is movement of the patient. Low-field systems tend to be more convenient—the GE system allowed surgery in the MR scanner; the Polestar is like an MR fluoroscope. Other systems require patient movement: for the Siemens system, into and out of the scanner by a rotating table, for the Philips movement of patient on a table. The IMRIS system brings the MR to the patient.11
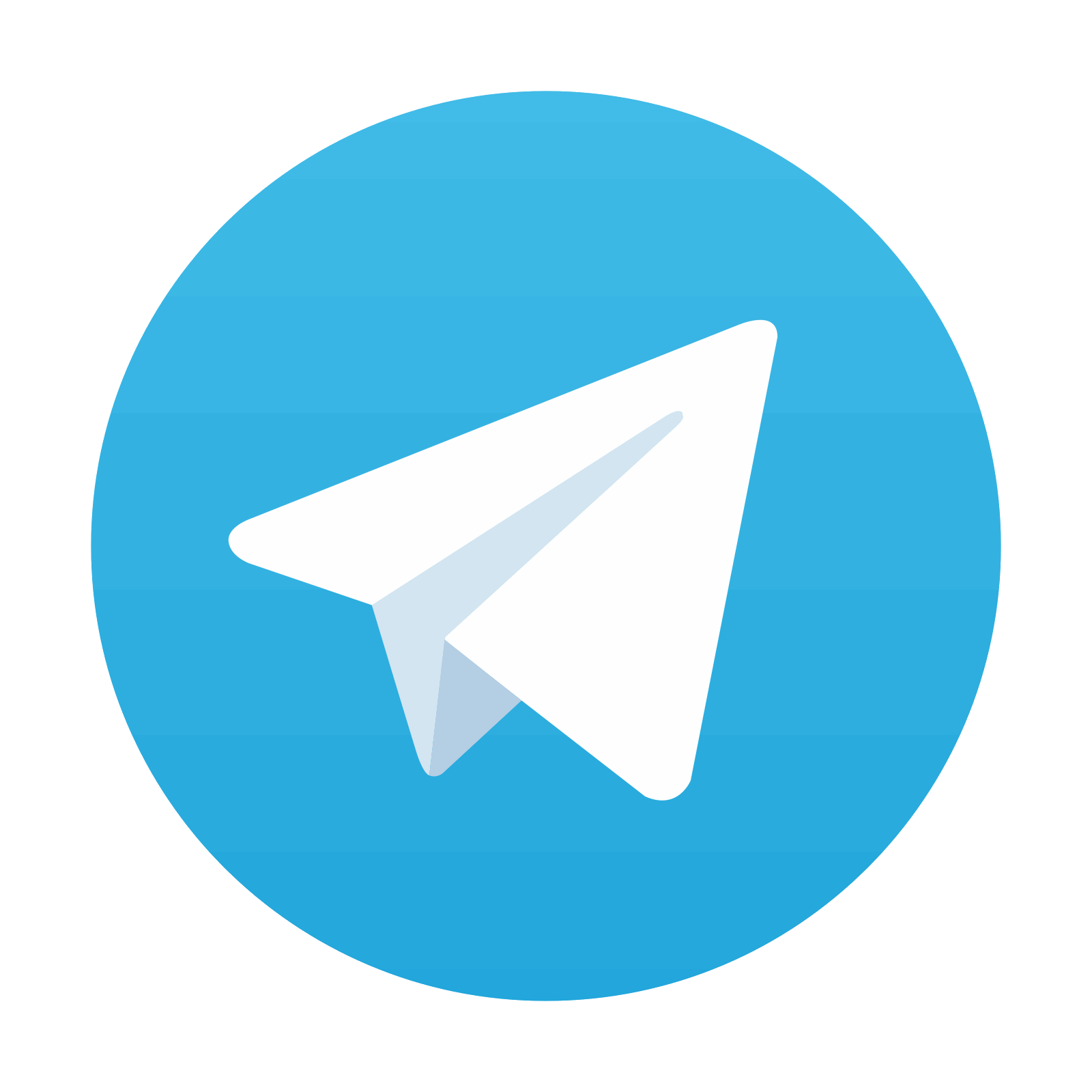
Stay updated, free articles. Join our Telegram channel

Full access? Get Clinical Tree
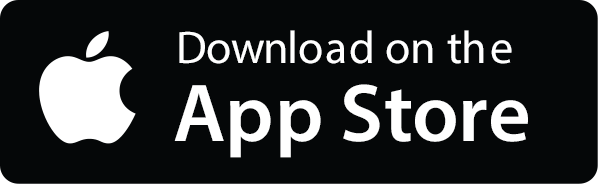
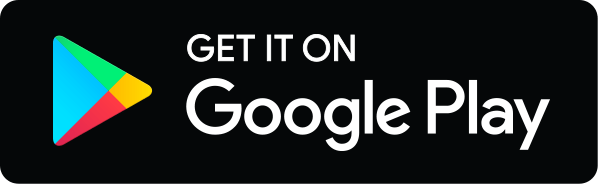