html xmlns=”http://www.w3.org/1999/xhtml” xmlns:mml=”http://www.w3.org/1998/Math/MathML” xmlns:epub=”http://www.idpf.org/2007/ops”>
Chapter 21 Emerging targets and other stimulation-related procedures in the management of Parkinson’s disease
Introduction
Since the first report from Benabid et al. [1] about the stimulation of the ventral intermediate nucleus (VIM) of the thalamus as a successful treatment for tremor, deep-brain stimulation (DBS) has revolutionized the management of movement disorders, especially Parkinson’s disease (PD).
Along the years, the therapeutic value of DBS of other brain targets in PD has been firmly established, such as the subthalamic nucleus (STN) and the globus pallidus internus (GPi) [2]. Between the two nuclei, the STN has become the most widely used target for DBS in PD. The significant improvement in motor complications and quality of life in PD patients brought about by STN-DBS has prompted research on new brain targets that could become an option for patients who are not candidates for STN-DBS or GPi-DBS. Indeed, STN-DBS and GPi-DBS are effective treatments for levodopa (l-DOPA)-responsive signs and symptoms but not for manifestations of PD that are nonresponsive to dopaminergic treatment such as axial signs and cognitive decline, the main determinants of disability and quality of life in advanced stages of PD.
In this chapter, we will present the most recent targets investigated for DBS for the treatment of motor and/or nonmotor symptoms in PD. For each target, we will provide the fundamental anatomical and physiopathological data that support its consideration for the management of PD, and will describe the advances made in clinical studies to assess its therapeutic value. In addition, we will review the advances made in noninvasive brain stimulation and introduce novel stimulation paradigms for DBS.
The pedunculopontine nucleus
The pedunculopontine nucleus (PPN) is a structure bounded laterally by the medial lemniscus and medially by the superior cerebellar peduncle and its decussation [3]. Superiorly, it reaches the retrorubral field dorsally and the substantia nigra (SN) anteriorly. The most dorsal aspect of the PPN contacts the pontine reticular formation, and the cuneiform and subcuneiform nuclei from a dorsal–ventral axis [3]. The most inferior portion of the PPN is adjacent to the locus coeruleus [3]. The PPN is divided into two parts based on cell density: the pars dissipata, composed of cholinergic, glutamatergic and other neuronal subtypes; and the pars compacta, containing mainly cholinergic neurons [3]. The PPN is, together with the cuneiform and the subcuneiform nucleus, the major component of the mesencephalic locomotor region [4], in which stimulation has been able to elicit controlled locomotion in decerebrated animals [3]. The PPN is richly connected with several structures, receiving inputs from the cortex, the limbic system, the basal ganglia, the spinal cord and the brainstem, especially the ascending activating reticular system. The efferent pathways ascend toward the thalamus and the basal ganglia (mainly the STN and the SN), and descend toward the cerebellum and the spinal cord [3]. With such a rich and wide network of connections, the PPN has been proposed as a relay station for the modulation of gait and posture [3]. Most of the studies conducted to determine the connectivity of the PPN were done in rat and primate models, although an overlap has been founded in the few rare human studies [5]. In PD patients, there is a reduction of neurons (average 40%) within the pars compacta [6], which strengthens the rational for an involvement of the PPN in the pathophysiology of PD and for exploring its therapeutic potential by directly modulating PPN activity with DBS.
The first reports of bilateral PPN stimulation in PD patients had promising results [7, 8]. Since then, several studies with variable methodological quality have assessed the effects of PPN-DBS in PD but with inconsistent results [9–14] (Table 21.1). The only two randomized, double-blinded studies available did not show any significant improvement in the objective ratings using the Unified Parkinson’s Disease Rating Scale (UPDRS)-III 1 year after surgery. Both studies reported significant improvements in secondary outcomes when measured in the off-medication condition: the item “falls” of the UPDRS-II [11] and freezing of gait using a walking protocol that included environmental triggers [10]. The improvements associated with PPN stimulation were still present after an average postoperative follow-up of 4 years in one of these studies [11] (T. A. Mestre and E. Moro, unpublished data). Overall, these clinical studies suggest that low-frequency stimulation (below 70 Hz) is more effective, although different ranges of frequencies have been used [15]. Possible stimulation-related side effects included contralateral paresthesias due to current diffusion to the medial lemniscus, oscillopsia [16] likely due to current diffusion to fibers from the uncinate fasciculus of the cerebellum and the superior cerebellar peduncle [17], and myoclonus due to stimulation of the thalamic projections [10, 16]. Despite the small and inconsistent therapeutic effects so far observed, PPN stimulation has not been dismissed as a target for axial levodopa-resistant signs and symptoms in PD patients. Indeed, there are several issues that may have interfered with the different outcomes and require clarification. One fundamental aspect is that the optimal site for stimulation of the PPN region and surrounding areas has not been fully established. There is an ongoing discussion about the best site being in the actual PPN or in adjacent areas such as the subcuneiform nucleus [10]. In one study, a more posterior location of the electrodes was associated with greater improvement, which was interpreted as a possible involvement of the cuneiform and subcuneiform nuclei [10]. However, this remains far from consensual, with other authors indicating other nuclei such as the peripeduncular nucleus [18] and the existence of inconsistent clinical/anatomical correlations (T. A. Mestre and E. Moro, unpublished data). Moreover, targeting the PPN raises important technical issues. The electrode location seems to vary greatly among the studies [4, 10, 11, 18–22]. Such findings can be justified by the absence of a specific identifying neurophysiological activity and of any acute clinical benefit with a localizing value, after electrode placement and stimulation. A novel alternative MRI-based targeting has been proposed as a more accurate method compared with traditional atlas-based methodologies [23].
Literature about pedunculopontine nucleus (PPN) stimulation in Parkinson’s disease patients; case reports have been excluded
Study | Sample size (n) | Target | Study design | Study duration (months) | Stimulation details | Results |
---|---|---|---|---|---|---|
Stefani et al. [9] | 6 | Bilateral PPN + STN | Assessments pre-op, 2–3 months (off med), 6 months (on med); physician rater blinded to condition | 6 | Bipolar contacts, 25 Hz, 60 μs, 1.5–2.0 V | PPN only: improvement in total UPDRS-III (33–44%) and items 27–30 (off- and on-medication). Decline of PPN-DBS efficacy overtime |
Moro et al. [11] | 6 | Unilateral PPN | Double-blinded assessments pre-op, 3, 12 months | 12 | Mono/bipolar contacts, 66.7 Hz, 70 μs, 2.0 V (mean values) | Improvement in falls (item 13,UPDRS-II) off and on med, at 3 and 12 months. No significant improvement in UPDRS-III and items 27–30 |
Ferraye et al. [10] | 6 | Bilateral PPN + STN | Unblinded assessments pre-op, 12 months plus double-blinded crossover between months 4 and 6 | 12 | Bipolar contacts (5/6 patients), 15–25 Hz, 1.2–3.8 V, 60–90 μs | PPN only: improvement of freezing (item 14, UPDRS-II). Freezing episodes significantly improved (P = 0.046) only off med. No improvement in the double-blinded assessment |
Thevastan et al. [13] | 5 | Bilateral PPN | Unblinded assessments pre-op, 6 and 24 months | 24 | Monopolar contacts, 35 Hz, 60 μs, 3.5 V (mean values) | UPDRS-III (total, items 27–30) – no significant improvement. Gait and Falls Questionnaire – sustained improvement until 24 months |
Khan et al. [12, 14] | 7 | Bilateral PPN + cZi | Unblinded assessments pre-op, 12 months | 10–13 (n = 7) | Bi-/tripolar contacts, 60 Hz, 60 μs, 2.6 V (mean values) | PPN only: improvement in UPDRS-III (total, items 27–30, tremor, bradykinesia) both off and on med. PDQ-39 – n/a. Longer f/u at 12–60 months (n = 5) – no significant improvement in UPDRS-III (total, items 27–30) |
STN, subthalamic nucleus; cZi, caudal zona incerta; med, PD medications; op, operative; n/a, not applicable; f/u, follow-up; stim, stimulation; UPDRS, Unified Parkinson Disease Rating Scale.
Although there is some evidence suggesting that bilateral PPN stimulation might be more effective than unilateral stimulation [14], this remains to be fully established. In addition, the sample size of these studies has been small, and the potential poor sensitivity of the clinical rating scales used to measure gait-related outcomes such as freezing of gait deserves further appraisal, as the UPDRS-III does not incorporate environmental triggers for freezing of gait in its administration [10, 11].
The PPN has also been studied as part of multitarget DBS strategies. Combination of PPN and caudal zona incerta (cZi) stimulation was associated with a cumulative effect compared with the isolated stimulation of each target for the motor UPDRS axial subscore in the on-medication condition [12, 14]. A corresponding cumulative effect was observed in the regional cerebral blood flow using 15OH2O positron emission tomography (PET) [24]. Bilateral combined STN and PPN stimulation has been suggested to provide a potential synergistic effect, as measured by gait kinematics [25] during the off-medication condition. More importantly, bilateral combined STN and PPN stimulation had a cumulative effect for improvement in the UPDRS-III and its axial item scores 3 months after surgery, in both the off-medication and on-medication conditions, as well as for measures of activities of daily living [9]. Nevertheless, the antiparkinsonian effects related to PPN stimulation waned in a period of 6 months, while the effects related to STN stimulation were more sustained during the same period of time [9]. This observation has been interpreted as tolerance to DBS, and an intermittent stimulation with an overnight arrest of PPN stimulation was adopted in another study [10], although it was never compared with continuous PPN stimulation.
Modulation of sleep and alertness was observed in PD patients with PPN-DBS operated on for levodopa-resistant gait and balance impairment. Unilateral PPN-DBS increased the duration of rapid eye movement (REM) sleep using a wide range of frequencies (5–70 Hz) in stimulation [26], whereas the N1 stage sleep was induced with higher-frequency (80 Hz) PPN stimulation [27]. More importantly, low-frequency (20–30 Hz) PPN stimulation was associated with increased alertness [27]. The increase alertness could partially explain the improvement of freezing of gait and falls observed in PD patients with PPN stimulation. Another interesting finding related to PPN stimulation has been the potential improvement in cognition documented together with a corresponding activation of bilateral prefrontal and frontal cortical areas in 18F-fluorodeoxyglucose PET [28]. These nonmotor effects of PPN stimulation warrant further exploration for the treatment of sleep disorders and cognitive impairment, although the benefits would have to be clinically significant to outweigh the surgical risk.
The posterior subthalamic area
The posterior subthalamic area (PSA) is delimited anteriorly by the posterior border of the STN, posteriorly by the medial lemniscus, inferiorly by the dorsal SN, superiorly by the ventral thalamic nuclei, laterally by the posterior limb of the internal capsule, posteromedially by the red nucleus and posterolaterally by the ventrocaudal nucleus [29, 30]. The PSA is formed by the nucleus of the zona incerta (Zi) and the white fiber tracts of the prelemniscal radiations [31]. The Zi, or the “region of which nothing certain can be said,” as described by Forel in 1877, is a small diencephalic nucleus that covers the STN and lies between the bundles of the pallidothalamic tract [31]. The Zi is considered a connecting node for basal ganglia and thalamocortical and cerebellar thalamocortical circuits [30]. The pallidothalamic tract has its origin in the GPi and is composed of the ansa lenticularis and the fasciculus lenticularis (or Forel H2 field in classical nomenclature), which have different trajectories and progressively merge to form the Forel H1+H2 field and, finally, the fasciculus thalamicus before entering the ventral thalamus [29]. The prelemniscal radiations lie posterior to the STN and are composed of the mesencephalic reticular formation projections to the thalamus and the ascending cerebellothalamic tract. The cerebellothalamic tract, or brachium conjuntivum, connects the dentate, interposed and fastigial cerebellar nuclei with the thalamus (ventral division of the ventral lateral posterior nucleus) passing through the superior cerebellar pedunculus and its decussation, and the red nucleus anteriorly [29]. It is important to recognize that the Zi and the prelemniscal radiations are difficult to distinguish on MRI [30], even on a 3-tesla device [32].
The exploration of the therapeutic potential of the Zi in PD patients is not novel. In the 1960s, there were multiple clinical studies on the effect of subthalamotomy for different types of tremor, including PD tremor and essential tremor but also dystonia and other movement disorders [30, 33]. Most of the subthalamotomies corresponded to lesions in the PSA [30, 33] and the studies reported a consistent effect for tremor as well as for rigidity in PD [30, 34]. Indirect evidence of the potential therapeutic benefit of stimulating the Zi was provided by studies of clinical/anatomical correlation after STN-DBS, suggesting that the Zi was directly involved in the therapeutic effects observed with stimulation [35–37], together with dorsal–lateral STN and Forel fields, although this interpretation is not consensual [38]. In addition, evidence suggests a specific effect in the reduction of dyskinesias and of the levodopa-equivalent daily dose [39]. In the past decade, five studies have assessed in a systematic fashion the effect of PSA stimulation on tremor, rigidity and bradykinesia in a total of 51 PD patients [40–44] (Table 21.2). Although these studies report consistent positive efficacy results for PD cardinal signs, these should be interpreted with caution, due to their small sample size and the poor methodological quality: the studies were nonrandomized, open label and most of them were noncontrolled. Stimulation of the PSA has been considered a safe procedure. Worsening of depression, pyramidal effects, dysarthria and dizziness were rarely reported as chronic stimulation-induced effects, although, at times, with a suboptimal control of tremor [43, 44]. Irritability, psychomotor agitation, severe progressive insomnia [45] and persistent apathy [46] have also been reported in isolated case reports. In a case series of 19 PD patients with a mean long-term follow-up of 4 years, PSA stimulation (both cZi and prelemniscal radiations) was associated with an incidence of depression and speech impairment comparable with the known incidence in STN-DBS, although the patient group in these case series already had mild speech impairment and/or behavioral disease (depression and apathy) preoperatively [47]. Potential deleterious effects in speech were assessed for stimulation of the Zi in a direct comparison with STN-DBS: a slight worsening in voice intensity [48] and articulation rate [49] was associated with Zi stimulation, but there was no difference in the control of onset/offset of phonation [50] and no change in pitch variability, while STN-DBS improved the latter [51]. Swallowing functioning was not affected [52]. In addition, current spread to neighboring cerebellothalamic tract and pallidothalamic fibers has to be considered with the potential risk of dysarthria and postural instability [53, 54] and blocking of levodopa effects [55–57], respectively.
Literature about posterior subthalamic area stimulation in Parkinson’s disease patients
Study | Patients (n) | Target | Study design | Study duration (months) | Stimulation details | Tremor | Rigidity | Bradykinesia |
---|---|---|---|---|---|---|---|---|
Velasco et al. [40] | 10 | Uniteral Raprl | Unblinded assessments pre-op, 3, 6, 9 and 12 months | 12 | Bipolar contacts, 130Hz, 232 μs, 2.8 V (mean values) | Pre-op vs 12 months | ||
↓ | ↓ | = | ||||||
on vs off stim (off med) | ||||||||
↓ | = | = | ||||||
Kitagawa et al. [41] | 8 | Unilateral Zi/Raprl | Unblinded assessments pre-op, 6, 12, 18 and 24 months, on vs off stim (off med) | 24 | Monopolar contacts, 132.5 Hz, 86.3 μs, 2.3 V (mean values) | ↓ (78.3%) | ↓ (92.7%) | ↓ (65.7%) |
Plaha et al. [58] | 14 | Unilateral (n = 13)/bilateral (n = 1) cZi | Unblinded assessments pre-op, 6 months, on vs off stim (off med) (cZi vs STN vs dorsomedial to STN) | 6 (median value) | Mono/bipolar contacts, 150 Hz, 82 μs, 2.7 V (mean values) | ↓ (93%) | ↓ (76%)a | ↓ (65%) |
Carrilo-Ruiz et al. [43] | 5 | Bilateral Raprl | Unblinded assessments pre-op, 3, 6, 9 and 12 months, pre-op vs post-op | 12 (n = 4) | Bipolar contacts, 130 Hz, 90–330 μs, 2.5–4.0 V | ↓ (90%) | ↓ (94%) | ↓ (75%) |
Blomstedt et al. [44] | 14 | Unilateral (13)/staged bilateral (1) cZi | Unblinded assessments pre-op, 12 months, on vs off stim (off med) | 18 (mean value) | Mono(n = 12)/bipolar contacts, 160, 66 μs, 2.6 V (mean values) | ↓ (82.2%) | ↓ (34.3%) | ↓ (26.7%) |
Raprl, prelemniscal radiation; cZi, caudal zona incerta; STN, subthalamic nucleus, med, PD medications; op, operative; stim, stimulation. Statistically significant results are presented for comparison.
* Significantly different from STN or dorsomedial to the STN.
The substantia nigra pars reticulata
The substantia nigra pars reticulata (SNr) is the main output nucleus of the basal ganglia, along with the GPi. The SNr projects ascending fibers to the thalamus with its inhibitory efferents to cortical areas [59] and to the mesopontine tegmental area [60]. In animals, modulation of SNr activity modifies both locomotion and control of posture [60]. The effect of bilateral SNr-DBS on different parameters of gait was compared with STN stimulation in seven PD patients initially treated with STN-DBS but later found to have the most ventral contact of the electrodes located within the SNr [60]. In a head-to-head comparison for the same patient and lead, SNr stimulation alone improved only axial signs (reduction of 44%), measured by an axial score composed of the sum of items 27–30 of the UPDRS-III (rising from chair, posture, postural stability and gait). Stimulation of the STN improved axial and distal parkinsonian signs by 74 and by 77%, respectively, the latter defined as the sum of nonaxial items of the UPDRS-III. The effect of SNr stimulation on the biomechanics of gait was also assessed [60] and was associated with a significant improvement of braking capacity but no change in step length or peak velocity for both natural and fast gait conditions. Stimulation of the STN improved the three gait parameters. The changes in the three gait parameters correlated with the “axial” score. More recently, a double-blind, randomized, crossover study compared STN stimulation alone with interleaving stimulation of the STN and SNr in 12 PD patients [61]. This novel advanced programming modality allows the alternate stimulation of different contacts of the same lead with different amplitudes and pulse widths, at a common frequency, which was 125 Hz for this study. The contacts of electrodes were located in the STN area and in the caudal STN–SNr border zone. After 3 weeks of continuous stimulation, either combined STN–SNr interleaving stimulation or isolated STN stimulation did not improve the compounded score of axial items combining corresponding items of the UPDRS-II and -III [61]. The Freezing of Gait Assessment Course suggested a greater improvement of freezing of gait with combined STN–SNr interleaving stimulation than with isolated STN stimulation [61]. Four patients prematurely discontinued isolated STN stimulation due to gait impairment [61]. Combined STN–SNr interleaving stimulation was safe and well tolerated [61]. Two patients developed dyskinesias that remitted with the adjustment of stimulation. To further test the apparent effect of combined STN and SNr interleaving stimulation on freezing of gait, a larger phase 3 study is warranted.
The centromedian–parafascicular complex
The centromedian–parafascicular complex (CM-Pf), or central complex, is part of the caudal intralaminar nuclei of the thalamus [62]. The CM-Pf is composed of several subdivisions with highly organized efferent projections into sensorimotor, limbic and associative parallel circuits of the basal ganglia [62]. The CM-Pf receives afferents from the SNr, PPN and GPi, but has reduced synaptic interactions with dopaminergic axonal projections [62]. The CM-Pf has marked neuronal loss (40 –55%) in PD [62], further strengthening the rational to explore its therapeutic potential in PD. Lesions to the CM-Pf with therapeutic goals in parkinsonian patients were first reported in the 1960s [63]. The effects of CM-Pf stimulation were reported initially in anecdotal cases of patients with chronic pain syndrome submitted to thalamic DBS, in whom an improvement of concomitant hyperkinesias as varied as tremor, stump dyskinesia or athetosis was observed [64, 65]. The combined approach of CM-Pf stimulation with standard targets for DBS in PD (bilateral STN or GPi) has been formally reported in eight PD patients [66–68]: CM-Pf stimulation alone was associated with a significant reduction in UPDRS-III scores, although GPi stimulation alone provided a greater improvement than CM-Pf stimulation alone. Combined CM-Pf and GPi stimulation had a significant synergistic effect [66]. In addition, CM-Pf stimulation caused a significant improvement in freezing of gait, while GPi stimulation did not [66]. These results were maintained for 3 years post-surgery [68]. Combined stimulation of the CM-Pf and bilateral STN was assessed in two PD patients [67]. After 6 months of continuous stimulation, the blinded assessed UPDRS-III score improved more with bilateral STN stimulation alone than with CM-Pf stimulation alone, while resting tremor improved more with CM-Pf stimulation alone. In these studies, a high-frequency (185 Hz) stimulation was used [66]. An anecdotal report of improvement of limb tremor in two PD patients after CM-Pf stimulation alone has since been published [47]. Limiting stimulation-induced side effects can be persistent paresthesias, facial muscle contraction or dystonia [66]. In a single case report, CM-Pf stimulation was tried in combination with the contralateral ventralis oralis posterior nucleus of the thalamus and the cZi for refractory PD tremor [47]. Good tremor control was reported but at the expense of severe speech impairment that only improved with switching-off of stimulation in either side [47]. The potential reduction in treatment-induced dyskinesias in PD was suggested in a group of patients submitted to VIM-DBS, in which a more posterior and deeper position of the electrodes, corresponding to the CM-Pf complex, was associated with an improvement of tremor and better control of peak-dose dyskinesias than the accurately located VIM stimulation [69]. In contrast, studies using a combined approach of CM-Pf and GPi stimulation did not report a significant antidyskinesia effect with CM-Pf stimulation alone [66]. Further studies are necessary to support these preliminary observations.
The nucleus basalis of Meynert
The nucleus basalis of Meynert (NBM) is an important cholinergic nucleus located in the basal forebrain and extends from the olfactory tubercle anteriorly to the level of the uncus of the hippocampus posteriorly [70]. The NBM is divided into six sectors with ascending projections to the neocortex, mainly with connections with the medial temporal structures, amygdala, and frontoparietal and temporoparietal cortices. There is a considerable overlap between individual subsectors for some innervated neocortical areas, which potentially makes these areas more protected from the effects of neuronal loss within specific sectors of the NBM [70]. Although dementia represents a brain neurodegenerative process and DBS has not been shown to be neuroprotective for currently used targets, it is postulated that stimulation of these pathways might enhance cholinergic activity in the innervated cortical regions and improve cognitive function, including memory and attention [70]. The case of a single patient with PD and PD dementia (PDD) has been reported after chronic combined stimulation of the NBM and STN. The laterodorsal portion of the intermediate part (Ch4 intermedius sector) was targeted, which corresponds to the largest section of the NBM [71], in the expectation of achieving a more widespread neocortical projection of the stimulation effects. Low-frequency stimulation (20 Hz) was used in order to induce an excitatory effect [72]. At 24 weeks after implantation, NBM stimulation alone was associated with cognitive improvement compared with the preoperative assessment, STN stimulation alone and sham NBM stimulation [72]. Performance in the Auditory–Verbal Learning and Memory Test and the Trail Making and Clock Drawing tests improved, but there was no change for delayed recognition and recall [72]. Ideomotor and ideational apraxia improved up to 18 months after the onset of NBM stimulation [73]. Phase 1 and 2 trials are ongoing to assess the effect of NBM stimulation in PDD and dementia with Lewy bodies (ClinicalTrials.gov identifiers: NCT01701544 and NCT01340001). Other targets, such as the fornix, which have been assessed in Alzheimer’s disease, could potentially be assessed for PDD in the future [74, 75].
Motor cortex stimulation
Motor cortex stimulation (MCS) has been proposed as an alternative surgical procedure in PD patients with motor fluctuations and dyskinesias with a contraindication for either STN-DBS or GPi-DBS. Motor cortical activity has been shown to be dysfunctional in PD patients: the primary motor cortex (M1) has reduced intra-cortical inhibition [76] and an increased metabolism with disease progression [77]. Motor cortex stimulation in PD was first introduced by Canavero in 2000 [78], after a seminal observation by Woolsey et al. [79]. Since then, a few human studies were conducted targeting of the M1 area (Table 21.3). Most of these studies adopted an epidural placement of the electrodes, with the exception of one study that used a subdural implantation, with the rational of achieving a more precise placement of the electrodes and a more direct stimulation, without the interposition of cerebrospinal fluid [80]. Most of these studies were case series, with the exception of one study [80]. The results have been mixed, ranging from the observation of no therapeutic effect to a long-lasting improvement of parkinsonian disability measured by the UPDRS-III or its item scores of rigidity and axial signs. When reported, the improvement could be observed on both sides of the body, even for unilateral MCS. A reduction in treatment-induced dyskinesias has also been reported. Most of the clinical studies were small in sample size, as well as nonrandomized and open label. In addition, MCS lacked a standardized approach regarding stimulation parameters, duration of stimulation, laterality of electrodes and localization techniques. Overall, MCS appears to be a safe procedure, without changes in cognition or behavior [80]. Nevertheless, the risk for seizures and surgical complications such as venous brain infarction has to be considered [82]. Other potentially valuable cortical targets such as the supplementary motor area (SMA) and the dorsolateral prefrontal cortex (DLPFC) have not been explored.
Literature about motor cortex stimulation in Parkinson’s disease patients
Study | Patients (n) | Target | Study design | Study duration (months) | Stimulation details | Efficacy results |
---|---|---|---|---|---|---|
Bentivoglio et al. (2012) [80]; Giuda et al. (2012) [81] | 11 | Unilateral M1 | Unblinded assessments, pre-op, 1, 3, 6 and 12 months, pre-op. vs on stim (off and on med) | 12 (n = 9) | Epidural bipolar contacts, 80 Hz, 125 μs, 3.7 V (mean values) | ↓ UPDRS-III (13.2% at 12 months), significant reduction in rigidity and axial symptoms. On med – no significant change |
Moro et al. (2011) [82] | 5 | Unilateral M1 | Unblinded assessments, pre-op, 12 months. Double-blinded (3 months), randomized on vs off stim (off and on med) | 12 | Subdural mono/bipolar contacts, 108 Hz, 76 μs, 3.3 V (mean values) | UPDRS-III – nonsignificant changes (P > 0.05), 3 and 12 months |
Arle et al. (2008) [83] | 4 | Bilateral M1 (n = 3) | Unblinded assessments, pre-op, 1, 3, 6 and 12 months, pre-op vs on stim, off med and on stim vs on med and on stim | 12 (n = 2) | Epidural mono/bipolar contacts, 100–130 Hz, 210–240 μs, 3.2–3.5 V | UPDRS-III – nonsignificant changes at 1, 3, 6 and 12 months (P > 0.05) |
Cilia et al. (2007) [84] | 5 | Left M1 | Rater-blinded assessment, pre-op, 6 months. Pre-op vs on stim, and off vs on stim (off and on med) | 6 | Epidural mono/multiple bipolar contacts, 40–60 Hz, 180–210 μs, 3–4 V | UPDRS-II and -III and Item 39, medication dosage and dyskinesias – no significant mean changes |
Cioni et al. (2007) [85] | 7 | Unilateral M1 (n3); bilateral M1 (n = 4) | Unblinded assessments, pre-op, 1, 3, 6 and 12 months. Pre-op vs on stim, (off and on med) | 12 | Epidural bipolar contacts; 80 Hz, 120 μs, 3–4 V | UPDRS-III (off -med) – mean improvement of 30% at 3 months, 22% at 12 months (no statistical testing) |
Pagni et al. (2008) [86] | 41 | Unilateral M1 | Unblinded assessments, pre-op, 1, 3, 6 and 12, up to 36 months, on stim (on vs off med) | 3–36 | 25–80 Hz, 90–180 μs, 2.5–6 V | ↓ UPDRS-III (19.1% at 36 months); ↓ axial item score, UPDRS-III (only at 24 months). On med – no consistent significant change. Reduction in UPDRS-IV scores (up to 24 months), no change in LEDD |
Gutiérrez et al. (2009) [87] | 6 | Unilateral M1 | Unblinded assessments, pre-op, 1, 3, 6 and 12 months, off stim vs off med and on stim vs on med and on stim | 12 | Epidural bipolar contacts; 21.7 Hz, 421 μs, 3.6 V | UPDRS-III – no apparent change (only individual patient description) |
DeRose et al. (2012) [88] | 10 | Unilateral M1 | Unblinded assessments, pre-op, 1, 3, 6, 12, 18, 24 and 36 months. pre-op vs on stim (off vs on med) | 36 | Epidural bipolar contacts; 20–40 Hz, 120–240 μs, 2.5–4.0 V | ↓ UPDRS-III – sustained (13% at 36 months), greater improvement on axial signs (28% at 36 months). On med – no significant change. Reduction in LEDD (at 36 months), treatment-induced dyskinesias (18 months) |
M1, primary motor cortex; LEDD, levodopa-equivalent daily dose; med, PD medications; op, operative; stim, stimulation; UPDRS, Unified Parkinson’s Disease Rating Scale.
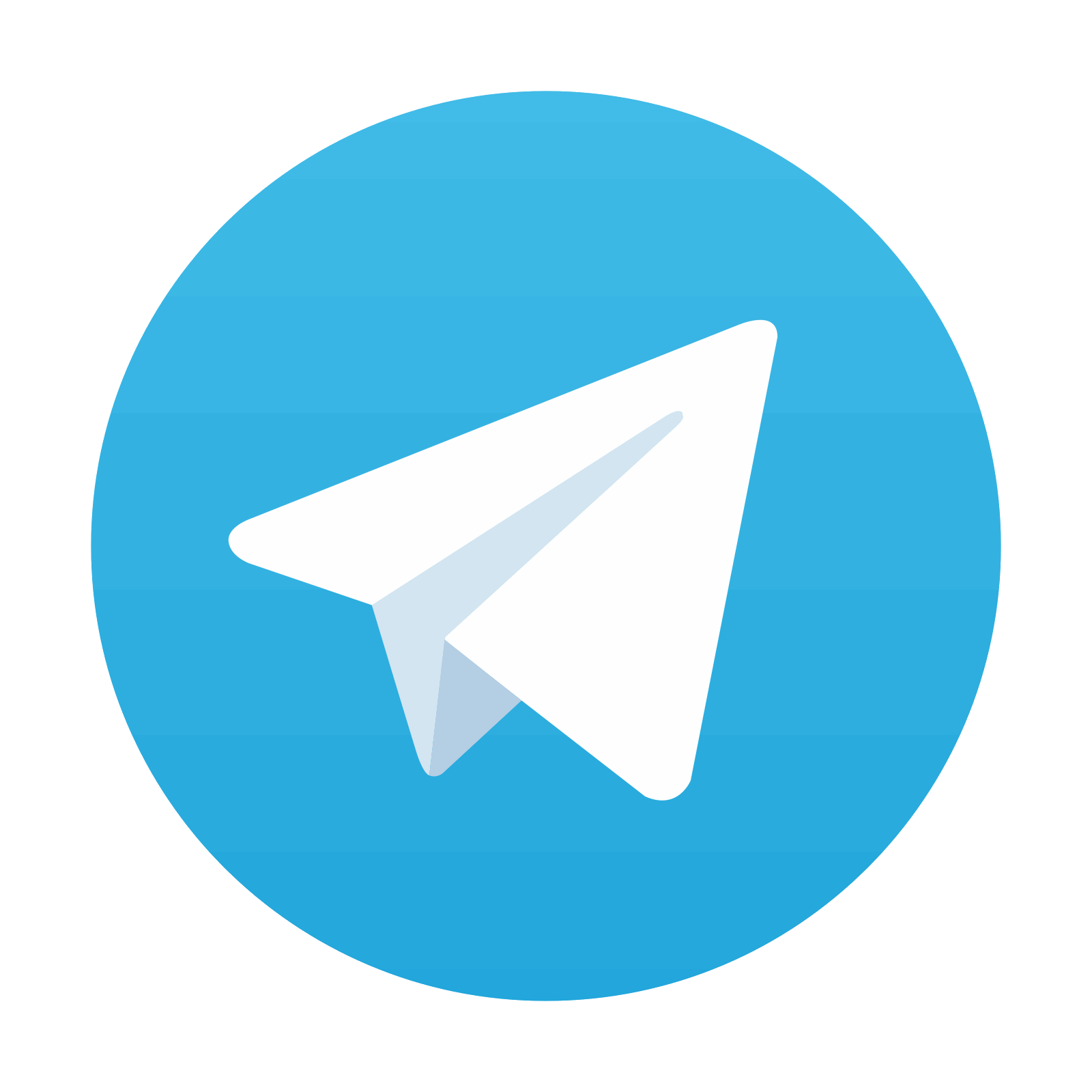
Stay updated, free articles. Join our Telegram channel

Full access? Get Clinical Tree
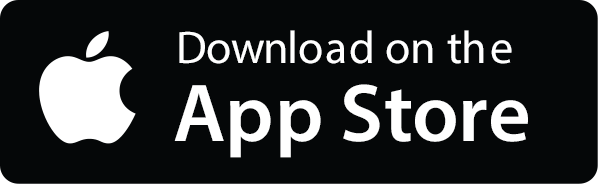
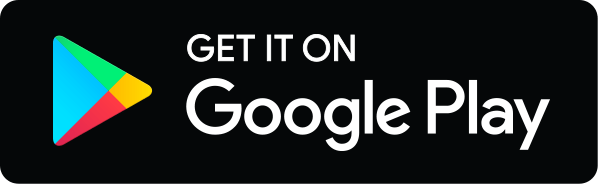