Years
Relationship sleep–GH
Relationship sleep–cortisol
Relationship sleep–glucose
1959–1969
During sleep, hourly glucose levels exhibit stable concentrations in nondiabetics [3]
1983–1989
Temporal association between sleep onset and inhibition of cortisol secretion (even if sleep is delayed or sleep–wake cycles are reversed or modified) [23]
Glucose levels increase until mid-sleep under constant glucose infusion [24]
1990–1994
– Close temporal and quantitative relationship between SWS or SWA and GH secretion (estimated by deconvolution). GH response to GHRH is enhanced during SWS and inhibited by awakenings [12, 13]
– Inhibitory effect of sleep is related to SWS [26]
1995–2000
– GHRH stimulates NREM sleep in intact and hypophysectomized rats, but stimulates REM sleep only in intact animals [18]
– Temporal coupling between EEG markers of alertness and cortisol secretion [33]
– Age-related changes in REM sleep and evening cortisol levels occur with identical chronology [15]
2004–2010
SWS and SWA are increased in GH deficiency of primary pituitary origin [20]
Until the second half of the twentieth century, no method was available to estimate hormone levels, and the existence of possible relationships between sleep and endocrine–metabolic function was never mentioned . At the end of the 1950s, a presentation by Yalow and Berson [1, 2] of a sensitive and specific radioimmunoassay, capable of measuring with a high degree of precision the insulin concentrations present in the circulation, opened a new and immense field for hormonal investigations. In 1959, it had also been shown that, despite prolonged fasting, glucose levels remain stable during sleep [3]. For years, however, no attempt was made to explore possible relationships between sleep and endocrine–metabolic function. In 1966, a first study [4] reported that, as judged from observations recorded in the diary, nocturnal GH peaks were regularly observed at times of likely deep sleep, leading the authors to hypothesize possible relations between GH secretion and sleep. A temporal association between slow-wave sleep (SWS) and elevated GH levels was evidenced in several studies [5–8], but other authors claimed that this relationship was fortuitous [9–11]. In the beginning of the 1990s, a new deconvolution procedure eventually allowed to determine with an extreme precision the amplitude and the temporal limits of GH secretory pulses and to unequivocally demonstrate the existence of a close temporal and quantitative relationship between SWS or slow-wave activity (SWA) and GH secretion [12, 13]. It was later shown that the age-related decrease in GH secretion during sleep occurs in parallel with the decline in SWS [14, 15].
For the past 20 years, the interrelations between GH and sleep have been extensively investigated and it is now well established that sleep is the major determinant of GH secretion in man. Conversely, GH-releasing hormone (GHRH) has been shown to stimulate SWS in animals [16–19] and in humans [16, 19]. Moreover, it was shown that SWS and SWA are increased in GH deficiency of primary pituitary origin [20]. Major steps are summarized in Table 50.1.
Meanwhile, the influence of the sleep–wake homeostasis on endocrine–metabolic regulation was progressively evidenced in many other fields. Two examples are illustrated in Table 50.1. In 1983, a first study showed that the 24-h rhythm of cortisol , though primarily driven by the circadian pacemaker [39], is also modulated by the sleep–wake cycle, since sleep onset is consistently followed by a drop in circulating levels of the hormone [23]. About 10 years later, it was shown that this decrease is related to SWS [26], and conversely that transient awakenings during the sleep period, as well as the final awakening at the end of a sleep period, trigger cortisol secretion [26–29]. A few years later, in 1998, it was shown that during the waking period, there is a temporal coupling between cerebral alertness and cortisol secretion [33]. In 2000, it was evidenced that the age-related increase in evening cortisol levels occurs as a mirror image to the decline in rapid eye movement (REM) sleep [15].
Sleep modulates glucose production as well. A number of studies confirmed the 1959 finding [3] reporting stable glucose levels during the sleep period in healthy subjects [40–45]. In the 1980s/1990s, constant glucose infusion [29, 24] or constant enteral nutrition [30] appeared to result in increased glucose levels during the first part of a nocturnal [24, 29, 30] or diurnal [29, 30] sleep period. A few years later, constant glucose infusion [36] and positron emission tomography [34, 35] studies showed temporal associations between reduced glucose utilization and a high amount of SWS during the first part of the sleep period, and between enhanced glucose utilization and high amounts of REM sleep during the second part of the sleep period [34–36]. Noteworthy, it was discovered that the brain is a glucose guzzler as its consumption is estimated as 30–50 % of the total body glucose utilization [37, 38].
Sleep Loss and Endocrine–Metabolic Function (Table 50.2)
Table 50.2
: Effects of sleep loss on glucose metabolism and appetite regulation
Studies | Sleep loss and glucose metabolism | Sleep loss and appetite regulation |
---|---|---|
Prospective population-based studies | – Difficulty in initiating and/or maintaining sleep [59, 60, 62], in falling asleep or using sleeping pills [63] predicts the risk of developing diabetes – Meta-analysis [66]: association between inadequate sleep and diabetes incidence | |
Laboratory studies Total sleep deprivation | ||
Laboratory studies Partial sleep restriction | – Decreased leptin levels [90–92], increased ghrelin levels [92], increased hunger and appetite [92] – Increased caloric intake [93–96], increased caloric intake for snacks [95] increased caloric intake from an ad libitum buffet [97, 98] – Muscle (rather than fat) loss under caloric restriction [99] | |
Laboratory studies Sleep quality alterations |
During the past decade, multiple epidemiological studies have documented a cross-sectional association between short sleep duration and increased risk for diabetes [46–54].
A number of prospective epidemiologic studies, more suitable at providing a direction of causality, have also examined the association between short sleep duration and diabetes prevalence [46, 55–65]. Many of them indicated a relationship between short sleep duration and diabetes prevalence [46, 55, 57, 58, 61, 64, 65], while others demonstrated a relationship between poor sleep quality and diabetes incidence [59, 60, 62, 63]. Only one study did not find any of these associations [56]. However, a meta-analysis [66] examining ten of these prospective studies reconciled the results to an association between short sleep duration and sleep disturbances, on the one hand, and the risk of developing diabetes, on the other hand. This association appeared to be stronger for men than for women [66].
The relationship between sleep loss and diabetes risk was also investigated in well-controlled laboratory studies. An alteration of glucose metabolism after total sleep deprivation had already been demonstrated in 1969 [78] and was confirmed later on by various groups [74–77]. Only one study [102] did not find any effect of one night of total sleep deprivation on glucose metabolism.
At the very end of the past century, exploration of bedtime curtailment, a phenomenon closer to everyday life, was initiated in laboratory studies. A pioneering study looking at the metabolic consequences of a semi-chronic sleep restriction was published in 1999 by Spiegel et al. [81], and rapidly became a “citation classic” (more than 1000 citations). Normal young men were submitted to 6 days of sleep restriction (4 h in bed), followed by 6 days of sleep extension (12 h in bed): sleep restriction (with partial preservation of SWS) led to alterations in glucose metabolism [81] , together with higher evening cortisol levels [81] and elevated GH concentrations during the waking period [103]. Ten years later, the impact of a semi-chronic sleep restriction on glucose metabolism was confirmed in middle-aged men and women submitted to 2 weeks of reduced sleep by 1.5 h daily compared to 2 weeks of extended sleep by 1.5 h daily [86], and in young men submitted to 1 week of 5-h bedtimes in a study that used a euglycemic–hyperinsulinemic clamp, the gold standard method to evaluate insulin sensitivity [82].
In the meantime, additional laboratory studies had examined the impact of partial sleep restriction on glucose metabolism . Most of them found that partial sleep restriction, for 1–7 days, resulted in increased insulin resistance [84, 85, 87–89]. Another study did not find any effect on glucose tolerance after 8 weeks of bedtime restriction in self-reported long sleepers [104] but the sleep-restricted group averaged more than 6.5 h of sleep at the end of the restriction period and slept only 16 min less than the control group [105]. Glucose tolerance remained stable in a study of a 4-day progressive sleep restriction from 7 to 4 h of sleep per night in women [93]. Recently, it was shown that individuals with a parental history of type 2 diabetes have a higher risk of developing diabetes if they are short sleepers [83].
Not only does sleep duration play a major role in the endocrine–metabolic function but sleep quality appears to be important as well. Using sound delivery to perturb sleep, a study has elegantly demonstrated that SWS suppression results in reduced glucose tolerance and increased diabetes risk, despite preservation of sleep duration and REM sleep [100]. Consistent with these results, sleep fragmentation markedly reducing SWS together with a small decrease in REM sleep without changing sleep duration also resulted in reduced glucose tolerance [101].
Sleep Loss and Appetite Regulation (Table 50.2)
Over the past decade, numerous cross-sectional studies have provided accumulating evidence for a robust association between short sleep duration and increased body mass index (BMI). Here, we cite the most important publications from 2000 to 2005 [106–112] but the number of references has grown tremendously. In 2008, a meta-analysis including more than 600,000 adults confirmed this relationship [113]. Although cross-sectional studies have mostly used self-reported sleep duration, the few that have measured sleep objectively via wrist activity monitoring [114, 115] and polysomnography [116, 117] found the same association between short sleep duration and the risk of obesity.
Most prospective studies [67–73], but not all [114, 118], including observations over 6 months to over 16 years suggested that sleep restriction predicts the incidence of obesity.
The mechanisms involved in the association between sleep duration and obesity were explored in well-controlled laboratory studies. Total sleep deprivation was found to result in decreased amplitude of diurnal variations of leptin (an anorexigenic hormone principally secreted by fat cells) [79] and in increased subjective hunger on the morning that followed a night of sleep loss [80]. In 2003–2004, studies consistently found that two [92], six [91], or seven [90] nights of partial sleep restriction (4 h in bed per night) resulted in a decrease in circulating levels of leptin [90–92] and a concomitant increase in circulating levels of ghrelin (an orexigenic hormone mainly secreted by gastric cells) [92], together with an increase in hunger and appetite [92]. Around the same time, two epidemiological studies [110, 119] extended these findings to long-term sleep loss, showing an upregulation of appetite with chronic sleep loss, controlling for BMI. Altogether, these findings suggest that sleep restriction is likely to result in increased food consumption. In fact, more recently, an increase in caloric intake was observed after four nights of progressively increasing partial sleep curtailment [93], after five nights of 4-h bedtimes [96], and after one night of 4-h bedtimes [94]. In addition, 14 days of sleep restriction (5.5 h in bed per night) versus 14 days of sleep extension (8.5 h in bed per night) with ad libitum access to food resulted in an increased intake of calories from snacks [95]. Three of these studies [93, 95, 96] obtained measurements of resting and/or total energy expenditure and showed no change in energy need with sleep loss , indicating that sleep restriction results in energy intake in excess. Preliminary data obtained in normal [98] and in obese subjects [97] indicate that caloric intake during an ad libitum buffet was increased after sleep restriction. In a crossover study comparing 5.5 h in bed per night versus 8.5 h for 2 weeks in overweight subjects, moderate caloric restriction resulted in similar total weight loss in both conditions, but sleep restriction was associated with increased hunger, decreased loss of fat mass, and increased loss of fat free mass [99]. Recently, a population-based study in 1088 pairs of twins showed that longer sleep duration was associated with decreased BMI and that shorter sleep duration could increase expression of genetic risks for high body weight [120].
Sleep Disorders
Obstructive sleep apnea (OSA) is one of the most common sleep disorders involving reduced total sleep time, sleep fragmentation, and low levels of SWS. Its incidence is rising rapidly, in relation with the epidemic of obesity. Noteworthy, the prevalence of OSA in morbidly obese subjects is estimated at 50–98 % [121]. It was shown in the 1990s that nocturnal release of the two pituitary hormones whose secretion is markedly stimulated by sleep (GH and prolactin) is decreased in OSA [122–124]. GH secretion during the first few hours of sleep [122, 123] and the frequency of prolactin pulses [124] are partially restored under treatment with continuous positive airway pressure (CPAP) . Years later, it was found that nocturnal luteinizing hormone (LH) and testosterone secretions were also decreased in OSA and partially corrected under CPAP treatment [125], and that adrenocorticotropic hormone (ACTH) and cortisol secretions were elevated in OSA and partially corrected by CPAP treatment [126, 127].
While obesity constitutes a major risk for OSA, OSA per se, independently of BMI, constitutes a risk for insulin resistance and therefore for diabetes: in 1993, a population-based study reported an association between snoring and abnormal glucose tolerance after adjustment for gender, BMI, physical activity, and alcohol and tobacco use [128], and a clinic-based study reported an association between the severity of OSA defined by polysomnography and insulin resistance [129]. In 1994, an improvement in insulin sensitivity was found in severely obese diabetic patients after 4 months of CPAP therapy [130]. Despite conflicting results (which can be due to differences in populations, selection of subjects, duration of therapy, variable compliance, etc.), there is now accumulating evidence suggesting that metabolic glucose abnormalities can be partially corrected under CPAP treatment, which is consistent with the concept of a causal link between OSA and altered glucose control (reviewed in [131, 132]).
Conclusions
Over the past 15 years, a large number of well-controlled studies have provided consistent evidence that partial sleep curtailment, a hallmark of modern society, increases the risk for obesity and diabetes. Given the morbidity and mortality associated with these diseases, the identification of novel potentially modifiable risk factors is particularly important. Future large field and interventional studies, incorporating objective measures of sleep duration and quality, should explore the potential benefits of sleep extension in short sleepers as a simple tool to reverse the adverse effects of sleep loss on diabetes and obesity risks.
References
1.
Yalow RS, Berson SA. Assay of plasma insulin in human subjects by immunological methods. Nature. 1959; 184(Suppl 21):1648–9.PubMed
2.
Yalow RS, Berson SA. Immunoassay of endogenous plasma insulin in man. J Clin Invest. 1960;39:1157–75.PubMedCentralPubMed
3.
Robin ED, Travis DM, Julian DG, et al. Metabolic patterns during physiologic sleep. I. Blood glucose regulation during sleep in normal and diabetic subjects. J Clin Invest. 1959;38:2229–33.PubMedCentralPubMed
4.
Quabbe HJ, Schilling E, Helge H. Pattern of growth hormone secretion during a 24-hour fast in normal adults. J Clin Endocrinol Metab. 1966;26:1173–7.PubMed
5.
Honda Y, Takahashi K, Takahashi S, et al. Growth hormone secretion during nocturnal sleep in normal subjects. J Clin Endocrinol Metab. 1969;29:20–9.PubMed
6.
Sassin JF, Parker DC, Johnson LC, et al. Effects of slow wave sleep deprivation on human growth hormone release in sleep: preliminary study. Life Sci. 1969;8:1299–307.PubMed
7.
Sassin JF, Parker DC, Mace JW, et al. Human growth hormone release: relation to slow-wave sleep and sleep-walking cycles. Science. 1969;165:513–5.PubMed
8.
Takahashi Y, Kipnis DM, Daughaday WH. Growth hormone secretion during sleep. J Clin Invest. 1968;47:2079–90.PubMedCentralPubMed
9.
Born J, Muth S, Fehm HL. The significance of sleep onset and slow wave sleep for nocturnal release of growth hormone (GH) and cortisol. Psychoneuroendocrinology. 1988;13:233–43.PubMed
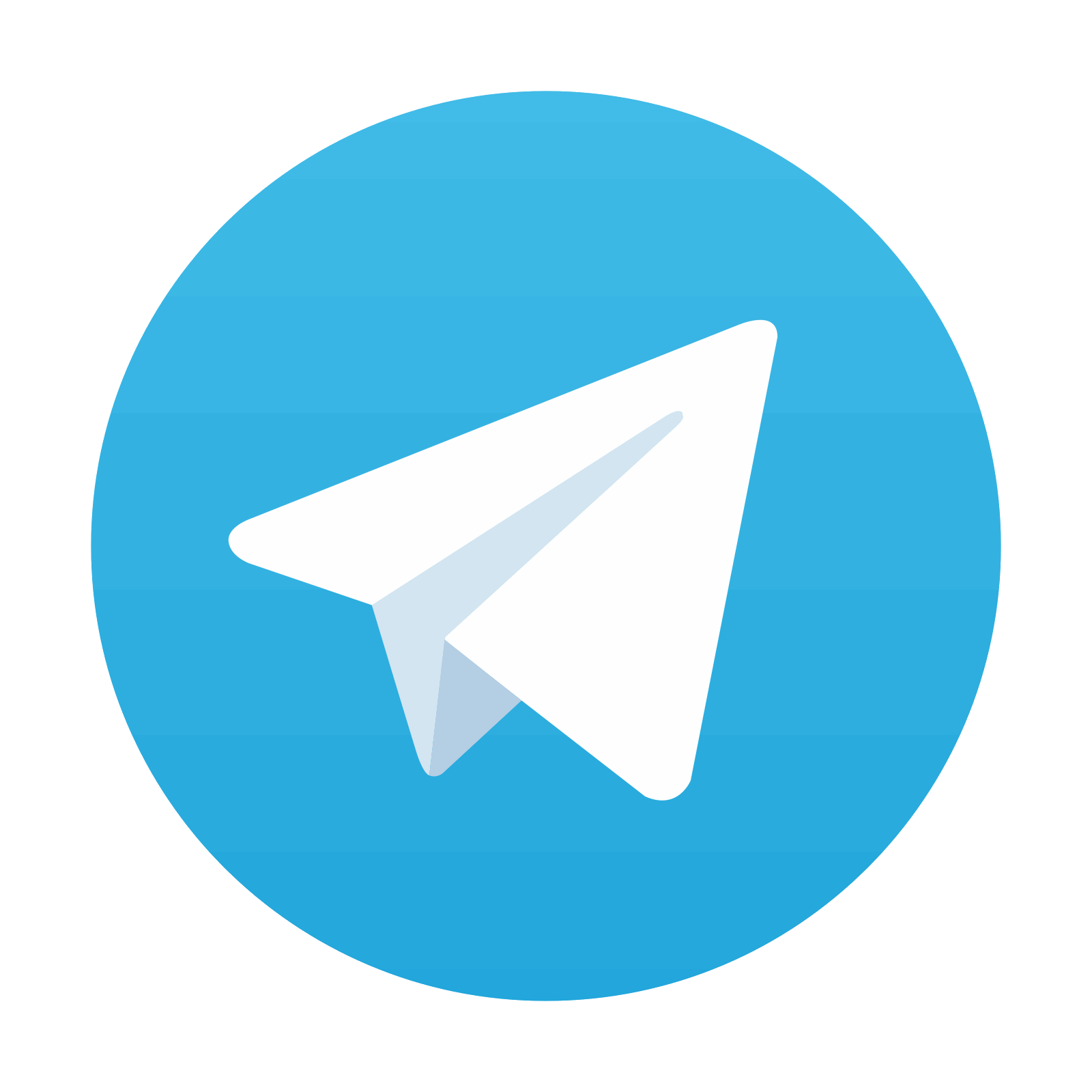
Stay updated, free articles. Join our Telegram channel

Full access? Get Clinical Tree
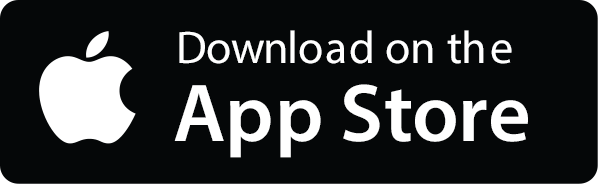
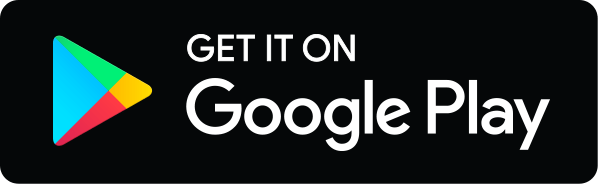