52 Endoscopic Endonasal Approaches for the Treatment of Vascular Lesions
Abstract
In this chapter, we present the technical nuances required for the treatment of vascular lesions through an expanded endonasal endoscopic approach (EEA). For this purpose, we briefly describe the background that has led skull base endoscopy to face modern surgical challenges such as resection of cavernous malformations, clipping of intracranial aneurysms, and removal of vascular tumors. We revise the most relevant anatomy from the bony and dural landmarks that safely guide an EEA, to the exposure of intradural neural and vascular structures involved in vascular pathology. We revise the preoperative evaluation, surgical technique, general and specific principles of reconstruction, and technological adjuncts available today for the treatment of these pathologies. Furthermore, a thorough literature review is presented in terms of worldwide experience in the treatment of brainstem cavernous malformations, anterior and posterior circulation intracranial aneurysms, and vascular tumors such as angiofibromas and hemangiomas. We present anatomical dissection photographs as well as clinical cases to further illustrate this chapter’s contents.
Keywords: endoscopic endonasal approach, intracranial aneurysm, cavernous malformation, vascular tumors, hybrid surgery
52.1 Introduction
Endoscopic endonasal approach (EEA) for skull base surgery started using the transsphenoidal corridor to reach pituitary tumors. Historically, the major issues for endonasal approaches were the illumination, visualization, and proper instrumentation. Gerard Guiot, a revolutionary neurosurgeon who learned from Norman Dott the transsphenoidal approach, improved his technique using intraoperative image intensification, fluoroscopy, and, for the first time, endoscopic visualization to reach the suprasellar region.1 After this initial experience, Apuzzo, Bushe, and Halves reported endoscopic-assisted microscopic resections for tumors with extrasellar extension.2 In 1992, Jankowski et al3 were the first to report a pure endoscopic transsphenoidal approach for pituitary tumors, which was later popularized and improved by Sethi and Pillay,4 Carrau et al,5 and Cappabianca et al.6 The technological improvements in frameless neuronavigation, high-quality endoscopic imaging, intraoperative imaging systems, angled endoscopes, and specialized instruments have allowed the neurosurgeon to reach into the sagittal plane from the frontal sinus to the odontoid process and into the coronal plane to the foramen ovale. Jho et al7 , 8 , 9 and Kassam et al10 , 11 , 12 , 13 have pushed further the concept of extended EEA and have described multiple anatomical corridors and developed several techniques to reach more complex skull base lesions beyond the sellar region.
The treatment of vascular lesions through the EEA is a complex challenge for the neurosurgeon that relies on the proper selection of the patient, a detailed preoperative analysis of the lesion, and an adequate equipment to safely perform the surgery. Since Frank and Pasquini developed the ethmoid-pterygoid-sphenoid endoscopic approach for the treatment of cavernous sinus lesions based on the transsphenoidal approach to a carotid-cavernous fistula described by Laws et al,14 , 15 the scope of EEA upsurged with the development of new techniques for the treatment of vascular lesions in the skull base. On the other hand, the improvements in skull base reconstruction using vascular pedicle flaps16 have supported the feasibility to reach intra-axial vascular lesions such as ventral brainstem cavernous malformations (BSCM).
In the following section, we will describe the vascular pathologies that according to our skull base team philosophy could be treated safely and effectively using the EEA.
52.2 Endoscopic Anatomy during Treatment of Vascular Lesions
Thorough anatomical knowledge is basic in order to treat vascular lesions through the EEA. The surgeon must be familiarized with all the structures located within the nasal corridor, the sphenoid sinus, the sellar, suprasellar, and parasellar regions, the clivus, and the cavernous sinus. Several anatomical publications have addressed the importance of recognizing such bony, dural, and surrounding neurovascular structures in order to safely guide the surgery during an EEA.10 , 11 , 17 , 18 , 19 For the purpose of this chapter, the most important anatomical landmarks used during treatment of vascular lesions would be separated into four categories: vascular, neural, bony, and dural anatomy.
52.2.1 Vascular Anatomy
The use of traditional internal carotid artery (ICA) classifications has been widely generalized.20 , 21 These are useful when assessing preoperative digital subtraction angiography (DSA), MRA, or CTA, or when dealing with vascular pathology such as intracranial aneurysms through lateral transcranial approaches. However, these classifications show some limitations when using the ventral corridor of the EEA, such as lack of clear divisions between segments of ICA or anatomical landmarks to guide its safe exposure. Labib et al’s endoscopic classification of the ICA has overcome these situations and has been helpful since its description.17 Roughly, it is divided into six segments named parapharyngeal (from common carotid artery bifurcation to carotid foramen), petrous (from carotid foramen to lacerum foramen), paraclival (from lacerum foramen to petrolingual ligament [superomedial petrous apex]), parasellar (from petrolingual ligament to proximal dural ring), paraclinoid (from proximal dural ring to distal dural ring), and intradural (from distal dural ring to ICA bifurcation). For the treatment of vascular pathologies such as aneurysms, knowledge of anatomy surrounding the paraclival, parasellar, paraclinoid, and intradural segments of the ICA are of utmost importance.
In terms of visualization of posterior circulation arteries, the V4 segment of the vertebral artery (VA) (exposed length: right side 1.7 ± 0.6 mm [range 0.8–2.8], left side 1.6 ± 0.6 mm [range 0.8–2.5]), proximal part of anterior medullary segment of the posterior inferior cerebellar artery (PICA) (exposed length: right side 0.5 ± 0.2 mm [range 0.2–1.4], left side 0.5 ± 0.2 mm [range 0.1–1.0]), vertebrobasilar junction, basilar trunk, and bifurcation (exposed length: 2.6 ± 0.3 mm [range 2.0–3.2]), and anterior pontine segment of the anterior inferior cerebellar artery (AICA) (exposed length: right side 1.1 ± 0.3 mm [range 0.6–1.7], left side 0.8 ± 0.3 mm [range 0.4–1.5]) can be exposed through a mid and lower transclival EEA.22
Furthermore, visualization of the basilar tip, proximal segments of the superior cerebellar artery (SCA), posterior cerebral artery (PCA), and posterior communicating artery (PComA) can be achieved once a pituitary gland (PG) transposition and an upper transclival approach is performed (see below).23 , 24
Early identification of perforating branches such as superior hypophyseal artery from intradural ICA, recurrent artery of Heubner arising from A1 and A2 segments of anterior cerebral artery (ACA), perforators from anterior communicating artery (AComA), short circumferential branches from basilar trunk, and thalamoperforator arteries from P1 segment of PCA should be identified early and preserved to prevent important postoperative morbidity.
52.2.2 Neural Anatomy
Optic nerves have been divided into five segments named intraocular, orbital, canalicular, preforaminal, and intracraneal.25 For the treatment of vascular lesions in the parasellar area, the last three are of most importance because of their relationship with the paraclinoid and intradural ICA. In order to decompress the nerve by 270 degrees to have full access to the ICA, drilling of the bone overlying the parasellar and paraclinoid ICA, the optic strut, the medial opticocarotid recess (OCR), and the tuberculum sellae is needed (see below). Once the optic nerve surrounded by its dural sleeve is exposed, a wide dural opening along its long axis should be extended on the upper part of its canalicular and preforaminal segments. On the contrary, when working on the inferior part of the optic nerve, the ophthalmic artery may be injured.26
The vidian nerve, formed by the junction of the greater superficial petrosal nerve (parasympathetic innervation) and the deep petrosal nerve (sympathetic innervation), travels through the vidian canal at the floor of the sphenoid sinus to reach the pterygopalatine ganglion in the pterygopalatine fossa. This nerve can be identified once the pterygopalatine fossa is unroofed at its posterior wall, or namely, at the base of the pterygoid process.27 A transpterygoid approach is performed, drilling the inferomedial aspect of the vidian nerve until reaching the inferior half of the foramen lacerum. Then, the nerve can be fully skeletonized and even transposed to prevent decreased lacrimation.28 The vidian nerve is located inferolaterally to the anterior genu of the petrous ICA; therefore, it helps to safely identify its depth and to prevent any injury.
52.2.3 Bony Anatomy
Preoperative analysis of a CT scan will help to identify the degree of sinus pneumatization, number and location of septations inside the sinus, or rare anatomical variations such as a sphenoethmoidal cell (Onodi), interclinoid osseous bridge, or bone dehiscences around the ICA, vidian or trigeminal nerve, etc.29 , 30 , 31
Once inside the sphenoid sinus cavity, careful inspection should be performed in order to foresee the location of hidden neurovascular structures regardless of sinus pneumatization. The most prominent bony landmark that should be first identified is the lateral OCR that corresponds to the optic strut, one of the anterior clinoid process attachments to the sphenoid body.19 , 26 This structure is interposed between the superolateral aspect of the parasellar ICA and the inferolateral aspect of the true canalicular portion of the optic nerve.26 Both lateral OCRs would help to define the midline in cases where many septations are present or in case of presellar pneumatization of the sinus (Fig. 52.1).29
The medial OCR10 , 19 is equivalent to the lateral tuberculum strut of the tuberculum sellae, and it resembles the MacCarty keyhole since its removal exposes the undersurface of the intracranial portion of the optic nerve superolaterally, the transition between paraclinoid and intradural segments of the ICA laterally, and the PG inferiorly. The tuberculum sellae is located between both medial OCRs and it is located superficial to the dura of the prechiasmatic sulcus, which will lead to the suprasellar cistern and the pituitary stalk once opened.
Middle clinoid process, although present in approximately one half of the population, helps to identify the roof of the cavernous sinus once it is removed, and hence locate the transition between parasellar ICA (cavernous segment of the ICA) and paraclinoid ICA.18 Below it, the anteroinferior venous space precedes the parasellar ICA, while above it, the paraclinoid ICA is only separated by a dural layer.
Posterior clinoid processes and dorsum sellae, part of the superior clivus from an endoscopic perspective, interpose along with the PG, between the nasal corridor and the interpeduncular cistern. Previous techniques have successfully achieved a pituitary transposition and posterior clinoidectomy, either through an extra-„, inter-„, or intradural approach32 to reach the cistern contents.
Clival recess, when visible, is located below the sellar region, between both paraclival ICAs and above the sphenoid floor.10 It leads to the mid-clivus dura and, therefore, to the pons, vertebrobasilar junction, basilar trunk, and AICA.
Despite the fact that it is not a true bony landmark, the fibrous tissue between the floor of the sphenoid sinus and pterygoid process, named as the pterygosphenoidal fissure,33 leads directly to the lacerum foramen which is the transition between the paraclival and the petrous ICA.
The inferior clivus is bordered superiorly by the sphenoid floor, laterally by the foramen lacerum and the petroclival synchondrosis, and inferiorly by the foramen magnum. It has an outer layer of cortical bone, an intermediate layer of cancellous bone, and an inner layer of cortical bone. The only landmark that can be seen on its outer surface, once the pharyngobasilar fascia is removed along with the rectus capitis anterior and longus capitis muscles, is the inferior clival line, just below the pharyngeal tubercle. It is located at the same level as the hypoglossal canal, which goes through the cancellous bone from a medial to lateral and posterior to anterior direction. Just deep to the inner cortical bone at the midline, venous bleeding from the basilar plexus can be controlled with hemostat packing. This window permits visualization of the VA, vertebrobasilar junction, premedullary cistern, PICA, and lower cranial nerves.34 , 35
52.2.4 Dural Anatomy
The dural folds and ligaments are used as landmarks when working through and around the cavernous sinus through a lateral corridor;36 37 38 the dural folds identified during an EEA provide further information about underlying structures. This is the case with the proximal dural ring which travels from the undersurface of the optic strut (lateral OCR) toward the middle clinoid process and defines the division between parasellar and paraclinoid ICA, as described earlier. This ring is more lax compared to the distal dural ring which is firmly adhered to ICA. Furthermore, the distal dural ring travels from the upper surface of the optic strut toward the medial OCR. Both rings encompass the paraclinoid segment of the ICA. A careful dural ring dissection away from the ICA is needed in case a C4–C5 segment, medial-pointing intracranial aneurysm is present, in order for the clip blades to completely obliterate the neck.
The other dural fold named the limbus sphenoidale,26 which corresponds to the medial projection of the falciform ligament, helps to define the limit between transtuberculum and transplanum approach, in case the anterior communicating complex needs to be exposed.
The anterior wall of the cavernous sinus must be safely opened when working with the paraclival, parasellar, and paraclinoid ICA. This requires removal of the middle clinoid, packing hemostats inside the cavernous sinus to avoid brisk venous bleeding, and identifying neural and dural structures inside it. Cavernous sinus ligaments were described recently in an attempt to further clarify the medial aspect of the cavernous sinus (medial wall) and its contents.39 Of utmost importance are the caroticoclinoid ligament which attaches the parasellar ICA (horizontal segment) to the anterior clinoid process and the medial wall, limiting its mobility; and the inferior parasellar ligament bounding the anterior cavernous sinus wall and parasellar ICA (vertical segment) with the medial wall. These ligaments should be identified and cut to prevent a vascular injury due to traction while performing a transcavernous approach, in case the exposition of the paraclival ICA for proximal control is needed.
In addition to the knowledge of anatomy, we firmly believe that laboratory training should be mandatory, and surgical experience should be obtained gradually from less to more complex cases. We strongly advocate the use of image guidance in every EEA to guarantee a safe procedure for the patient.
52.3 Intraoperative Fluorescence and Imaging Tools in EEA for Vascular Lesions
Intraoperative use of different imaging and fluorescence techniques for transcranial surgery has become a useful and frequent practice, since they have proven to reduce the incidence of postoperative complications, to enhance the extent of resection, and to keep vasculature and blood flow intact reducing the occurrence of ischemic events. Some of these techniques are now beginning to be adopted in the EEA, by adapting them to traditional endoscopic systems or by the design of new endoscopic systems with integrated imaging modalities.40 , 41 , 42 Also, some of these techniques can be used in combination with hybrid procedures (see below) to achieve the best results.
52.3.1 Endoscopic Indocyanine Green (endoICG)
Indocyanine green (ICG) is a near-infrared (NIR) contrast agent that is frequently used for intraoperative video angiography (ICG-VA) since it binds to plasma proteins and by remaining in the intravascular compartment it emits a bright white image of the vessels in comparison to the surrounding dark tissue.40 , 41 , 42 Its main advantage is the proper visualization and identification of both normal and abnormal vasculature.40 , 41 , 43
In the setting of EEA, this technique has been used in recent years since the development of endoscopic devices with ICG integrated systems, such as the Iridium system (Visionsense, Philadelphia, USA), the D-Light P system (Storz, Tuttlingen, Germany), or the Visera Elite II system (Olympus Co Ltd, Tokyo, Japan). Up to now, in most cases, the endoscopic ICG-VA is used for localizing the ICA in sellar and parasellar procedures41 , 44 , 45 or to verify the perfusion of the nasoseptal flap (NSF).46 In a recent publication,40 the authors described a “second-window ICG” technique for visualization of tumors during an EEA, which relies on the enhanced permeability and retention effect. Although there are no publications to date on the use of endoICG-VA for the treatment of vascular lesions through an EEA, there is a report regarding its use for the treatment of concurrent perimedullary and dural arteriovenous fistulas in the cervical spine.47 Perhaps this use may be applied for the treatment of vascular lesions through an EEA, such as medial ophthalmic segment aneurysm, to corroborate the absence of residual aneurysm and patency of parent and emerging vessels.
52.3.2 Sodium Fluorescein
Sodium fluorescein is a fluorophore used in neurosurgery mainly for cranial approaches to resect high-grade gliomas, metastasis, or vascular lesions, such as aneurysms or arteriovenous malformations (each having a distinct application procedure and dosage). This fluorophore can be seen either by direct visualization (high doses) or under microscopic filters (low doses), like the Yellow 560 filter (Karl Zeiss, Germany).48 , 49 , 50 Traditionally, intrathecal application of sodium fluorescein has been used to detect cerebrospinal fluid (CSF) leaks through the endonasal corridor,51 , 52 which also may aid the reconstruction of the surgical defect after intradural and intra-arachnoid dissection for the treatment of vascular lesions. Since there are not many endoscopy systems that integrate an adequate filter for the use of sodium fluorescein, previous attempts to use this technique have been made through hybrid microscopic/endoscopic procedures for fluorescence-guided endoscopic resection of sellar tumors53 or by adapting filters to regular endoscopy systems in a case of endoscopic use of fluorescein video angiography for transcranial aneurysm clip, as reported by Hashimoto et al.54
To date, there are no publications on the use of sodium fluorescein for the endonasal endoscopic treatment of vascular lesions; however, since the principles are similar to endoICG video-angiography, we may expect further reports of endoscopic filters for this fluorophore.
52.3.3 Intraoperative 3D Angiography (3D-DSA)
Hybrid procedures (neuroendovascular and microsurgical) for aneurysm clipping are becoming more and more frequent as newer hybrid operating rooms are being built around the world. The use of intraoperative 3D-DSA offers many advantages55 , 56 , 57 , 58 such as:
●Detect the presence or absence of residual aneurysm in the neck
●Evaluate patency of parent and neighboring arteries
●Detect and/or treat vasospasm
●Provide endovascular proximal control
●Obtain better image quality and to manipulate it in a three-dimensional fashion
●“Road-mapping”-guided clipping technique
52.4 Reconstructive Techniques in EEA
An effective and safe reconstruction technique is as important as the EEA itself. Over the last decades, the reconstruction techniques have evolved in order to reduce the risk of CSF leak.59 The multilayer techniques that were mainly used in microsurgical approaches have been adapted to endoscopy and have become the standard of care in EEA, with some variations. The NSF is currently considered the mainstay reconstructive method for all dural defects along the midline.60 For the purpose of this chapter, only the reconstruction techniques that we have used for the treatment of vascular pathology will be described, mainly to cover the sellar, parasellar, planum sphenoidale, and upper and middle clivus regions.
The reconstruction technique for EEA is divided into three phases: nasal, sphenoidal, and sealing/packing.61
52.4.1 Nasal Phase
Most of the EEAs tailored to treat vascular pathology are expected to have a high-flow intraoperative CSF; therefore, a wide NSF must be harvested at the beginning of the surgery and a reverse flap may be considered to protect the denuded septum.16 , 62 , 63 We usually preserve the middle turbinate to preserve nasal physiology as much as possible, but it can be removed if more lateral access is needed. The NSF is stored in the nasopharynx through the choana until the approach is completed; however, in expanded clival approaches, it may be placed inside the maxillary sinus.63
52.4.2 Sphenoidal Phase
Once the rostrum sphenoidale is removed, the vascular pathology is treated and careful hemostasis is performed; we make sure all the mucosa surrounding the surgical defect is denuded ( Fig. 52.2a) to ensure better adherence of the NSF to the osseous opening.61 Two things must be considered at this stage: first, an excessive bony removal and a dural defect larger than 3 cm will complicate reconstruction, risking a CSF leak; and second is that NSF must be protected at all times during the vascular pathology treatment. The NSF is the cornerstone of the reconstruction.64 Therefore, the exposure must be large enough to adequately treat the underlying pathology but small enough to permit a better reconstruction.

Full access? Get Clinical Tree
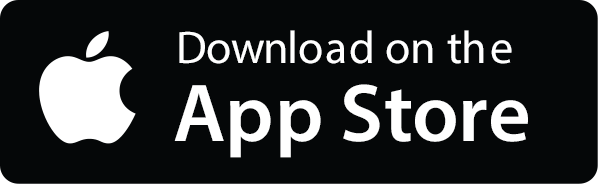
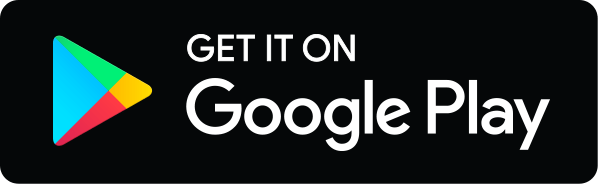