Endoscopic Third Ventriculostomy in Normal Pressure Hydrocephalus
Endoscopic third ventriculostomy (ETV) is a well-established treatment for obstructive hydrocephalus. In patients with obstructive hydrocephalus, ETV may replace a shunt system and thus help avoid shunt-related complications, such as shunt infections, shunt dislocations, obstructions, and overdrainage. The development of endoscopes and instruments, as well as video cameras with high definition (HD) technology, has improved the safety of endoscopic procedures. However, for a long time the indication was restricted to pure obstructive hydrocephalus, such as aqueductal stenosis, posterior fossa tumors, and fourth ventricle outlet obstructions. The advantages of shunt avoidance were so obvious that a search began for more indications for ETV. Following this, ETV was also performed in communicating forms of hydrocephalus, which led to an ongoing debate of whether ETV could be helpful in communicating hydrocephalus, even in idiopathic normal pressure hydrocephalus (iNPH). Today, two extreme practices exist: some neurosurgeons simply indicate ETV as a first-line procedure for all patients with hydrocephalus, while others implant only shunts. However, even though ETV is a safe procedure, it is not completely risk-free (see below); therefore, a pathophysiologic rationale should explain why ETV could work in communicating forms of hydrocephalus. From the ethical point of view it is critical to offer a patient an ETV, without understanding why it could work. Presently, several rationales have been developed that make an indication acceptable for ETV, at least in some cases of communicating hydrocephalus and NPH.
12.1 Rationale for ETV in Communicating Hydrocephalus and NPH
The bulk flow theory provides a good explanation of why ETV helps in obstructive hydrocephalus such as aqueductal stenosis: by perforating the floor of the third ventricle a communication between the ventricular system—where cerebrospinal fluid (CSF) is formed—and the subarachnoid space—where CSF is reabsorbed—is established. The obstruction/aqueductal stenosis is bypassed through this communication.
But, how can we explain a successful ETV in communicating hydrocephalus and even in NPH? One explanation could be infratentorial intracisternal obstructions (or infratentorial intracisternal obstructive hydrocephalus [InfinOH]).1 These obstructions may be a result of previous meningitis or infratentorial subarachnoid hemorrhage, or they may be of unknown origin (idiopathic). In these cases, there is still communication between the ventricles and the subarachnoid space/great cistern, which means it is still a communicating hydrocephalus. However, if no real communication exists between the great cistern and the prepontine cistern, CSF cannot reach the supratentorial reabsorption sites. A perforation at the floor of the third ventricle bypasses this infratentorial intracisternal obstruction, and does so in exactly the same way as in aqueductal stenosis. InfinOH is characterized by a downward bulging floor of the third ventricle, and a forward-pushed lamina terminalis as a sign of pressure difference between the third ventricle and the surrounding subarachnoid space (▶ Fig. 12.1). The infratentorial obstruction cannot be visualized directly, but indirect signs are clear, and similar to those of a dam: the prestenotic/predam spaces (ventricles and great cistern) are enlarged, and the poststenotic spaces (prepontine cistern and supratentorial subarachnoid space) are of normal size or narrower. These signs can be seen clearly in thin sagittal T2-weighted magnetic resonance imaging (MRI) slices (see ▶ Fig. 12.1). The rationale is confirmed by resolution of clinical signs of NPH as well as by resolution of the bulging membranes after ETV (▶ Fig. 12.2). Criticism to this InfinOH subtype is that it is no idiopathic but a secondary NPH. It might be that the prepontine obstructions are caused by preceding events such as mild meningitis or even hemorrhage. However, in many patients these events were probably asymptomatic or not remembered by the patient; so, from a clinical point of view (if no cause is found), idiopathic NPH must be assumed. However, the cases of InfinOH account for about only 10% of iNPH2; thus, with this concept successful ETV can be explained only in 10% of iNPH patients (see ▶ Fig. 12.2).
Fig. 12.1 Infratentorial intracisternal obstructive hydrocephalus (InfinOH) pre-endoscopic third ventriculostomy. The floor of the third ventricle bulges downward and the lamina terminal is pushed forward. The normal position is shown with dotted lines. No obstruction of the cerebrospinal fluid pathway can be seen directly.
Fig. 12.2 Infratentorial intracisternal obstructive hydrocephalus (InfinOH) post-endoscopic third ventriculostomy. The floor of the third ventricle is no longer bulging downward nor is the lamina terminal pushed forward.
The hydrodynamic concept of reduced intracranial compliance in the development of iNPH has a very different approach to explaining successful ETV in iNPH. The decrease of elasticity and capacitance of cerebral vessels leads to reduced cerebral blood volume. The consequence is a reduction of cerebral blood flow and intracranial compliance, followed by increased intracerebral pulse pressure. The increased systolic pressure pushes the brain toward the skull and compresses the periventricular spaces; this explains the ventricular enlargement with narrowing of the subarachnoid space.3,4 Opening the floor of the third ventricle allows flow out of the ventricular system, reducing the water-hammer effect of the systolic pulse pressure, and stopping the ongoing pathogenic mechanism of iNPH. The same effect is realized with a shunt, which is even pronounced if a slight overdrainage is present. If this concept is correct, then ETV should be effective in iNPH as well as in chronic hydrocephalus, which is thought to have a similar pathogenesis. However, this theory and the results are very controversial (see below).
12.1.1 ETV in Shunt Failure
ETV is often discussed as an alternative to shunt revision, but the outcome of ETV usually depends on the cause of hydrocephalus. ETV is very successful in obstructive hydrocephalus after shunt malfunction and only partially successful in patients with communicating hydrocephalus.5 When ETV is successful in the latter group this might be explained by the InfinOH and/or by the hydrodynamic concept.
12.2 Technique
ETV is a well-established procedure, and the technique might differ in only a few minor details. ETV can be performed with a rigid or a flexible endoscope. For visualization purposes, the endoscope is connected to a video camera and the images are seen on a screen located in a position that is comfortable for the surgeon.
12.2.1 Preoperative Planning
For planning the endoscopic procedure, MRI—if possible—should be available. Accurate planning is necessary to minimize complications. Imaging should help to find the optimal entry point for the endoscope, should show the size of the foramen Monro, and anomalies of the anatomy of the floor of the third ventricle that could interfere with a safe perforation (i.e., basilar artery aneurysms, elongations of the vessels, arteriovenous malformations). The space between the pons and the clivus should also be observed to determine whether the space is large enough to perform the ETV.
Usually, a rigid endoscope is introduced in the lateral ventricle via a frontal paramedian bore hole. The site of the bore hole should be determined by extension of the line from the tuber cinereum to the foramen of Monro (▶ Fig. 12.3). The tuber cinereum is situated between the mammillary bodies and the infundibulum recess/clivus and represents the position for the perforation of the floor of the third ventricle. The upward extension of the described line toward the skull indicates the site of the bore hole (▶ Fig. 12.3 and ▶ Fig. 12.4). The exact position of the bore hole on the patient’s skin can be determined by measuring the distance from the nasion (see ▶ Fig. 12.3 and ▶ Fig. 12.4) or, of course, by neuronavigation. The bore hole should be about 2 cm from the midline; when coming more from the lateral direction, the rigid endoscope will reach the floor of the third ventricle on the contralateral side, endangering structures such as the hypothalamus, oculomotor nerve, and the posterior communicating artery. With a more medial bore hole, there is risk of lesion of a bridging vein or even of the sagittal sinus. The exact position of the bore hole is especially important if the foramen of Monro is small. Incorrect positioning of the bore hole must be compensated by stretching the foramen of Monro with the endoscope to reach the above described perforation site at the floor of the third ventricle. If the delicate structures around the foramen are pulled or pushed it may lead to its damage and cause neurologic deficits.
Fig. 12.3 Determination of the bore hole site for endoscopic third ventriculostomy (ETV). The site of ETV and the foramen of Monro are marked on a sagittal magnetic resonance image (MRI) (black circles). The trajectory linking these structures, when extended upward to the skull, determines the entry point for the endoscope. The distance from the nasion to the entry point is measured on the sagittal MRI slice, which is then transferred to the patient’s head.
Fig. 12.4 Localization of the bore hole for endoscopic third ventriculostomy: 2 cm paramedial, approximately at the coronal suture; the exact distance is measured from the nasion (orange line), determined by the sagittal magnetic resonance image.
12.2.2 Positioning of the Patient
The patient is placed in a supine position with the head tilted slightly forward so that the bore hole is almost at the highest point of the head. This prevents air from entering the ventricles, which could impair visualization.
12.2.3 Surgical Technique
After preoperative prophylactic antibiotics, skin disinfection, and sterile covering, a skin incision and bore hole trepanation are performed. The dura is opened. The arachnoid, pia, and cortex are coagulated and incised. Depending on the endoscope design and size, the endoscope sheath, with or without the endoscope, is pushed gently through the brain toward the ventricle. It is directed toward the tragus in the sagittal plane and toward the nasion in the coronal plane. Caution is needed if the brain and the ventricle walls are stiff: the ventricular wall may be pushed forward by the blunt endoscope, without the endoscope entering it. A prepuncture with a Cushing needle opens the ventricle wall more easily and helps the endoscope enter into the ventricle without pushing and dislocating the wall of the ventricle.
Once in the ventricle, the surgery can be continued under visual control. Preferably a 0° endoscope optic is used. First, orientation is needed: if the foramen of Monro is not directly in view, the tip of the endoscope must be moved anteriorly if the plexus is visible. The choroid plexus leads toward the foramen. If the plexus is not visible, then the endoscope is in the choroid plexus–free frontal horn and must be moved posteriorly.
The foramen of Monro (▶ Fig. 12.5) is bounded anteriorly and medially by the fornix and laterally by the thalamus. The posterior border is covered by the choroid plexus, which continues to the roof of the third ventricle. At the lateral boundary, the thalamostriate vein can be identified, which drains toward the internal cerebral vein at the roof of the third ventricle. The septal vein is visible at the medial site.
Fig. 12.5 Foramen of Monro with the choroid plexus in the posterior part and the fornix, which together form the anterior and medial borders of the foramen.
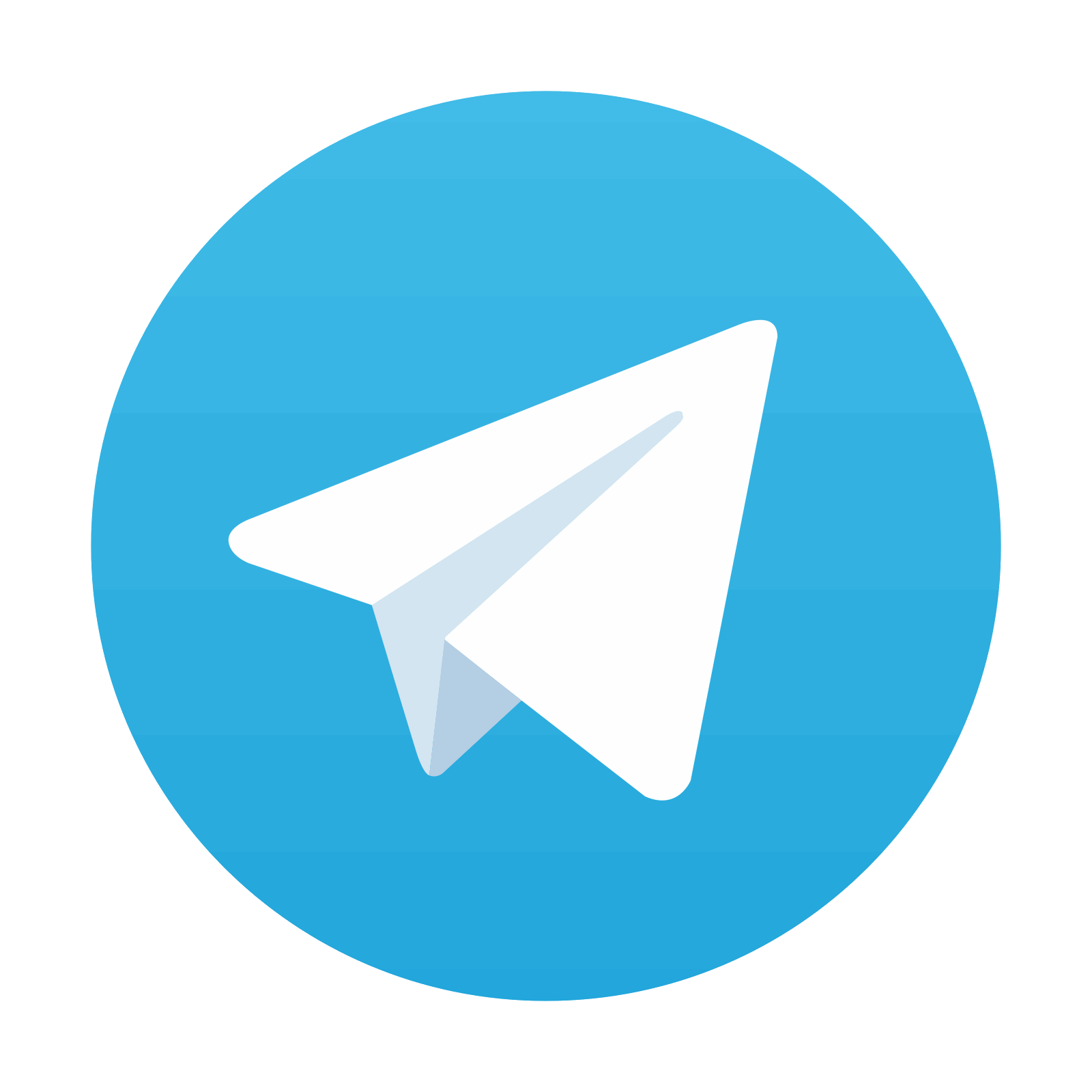
Stay updated, free articles. Join our Telegram channel

Full access? Get Clinical Tree
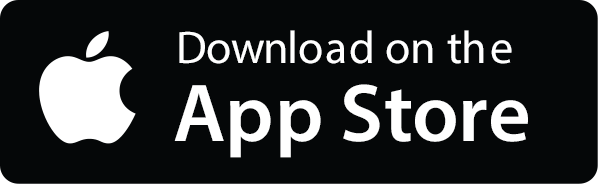
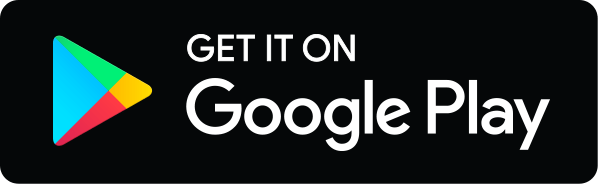