7 Ependymal/Deep Dissection “Ependymal” dissection may be a confusing term because many arteriovenous malformations (AVMs) do not reach a ventricle, but it is meant to describe the deep dissection that comes at the end of the resection. In terms of the AVM box, the ependymal plane is the bottom, the sixth and final side. In terms of brain anatomy, the ependymal layer is the tip of the classic AVM cone tapering to ependyma. This phase of dissection, whether actually ependymal or not, is deep and diametrically opposite to the position of the neurosurgeon, and, as such, is inherently difficult to see, like the dark side of the moon. The ependymal plane is darkened and physically blocked by AVM nidus, making it the most challenging one. To make matters worse, this plane receives perforating and choroidal artery supply which can be troublesome. I always anticipate some bleeding during ependymal dissection; I am prepared if it comes, and pleasantly surprised if it does not. Therefore, before beginning ependymal dissection, all of the parenchymal dissection should be completed, the other AVM sides should be free, and the nidus should be mobile. It is easy to get lured down to the ependymal plane and lose sight of unfinished parenchymal connections on another side. The resection cavity is lined circumferentially with Telfa strips to protect parenchyma from manipulation or retraction, but more importantly, to deal with deep bleeding. If bleeding fills the surgical corridor, Telfa strips provide extra-anatomic landmarks that are more orienting than bland parenchyma, and they are protective. Retractors solve the problem of deep visualization. A self-retaining retractor placed on the AVM mobilizes the nidus from side to side, and importantly elevates it as well. Lifting an AVM enhances the view of the ependymal plane and places deep connections on traction. Although I try to limit fixed retractors to just one, a retractor on the brain may also angle the viewing trajectory across the ependymal plane when the walls of the cavity are steep and deep. Retractors and retraction pressure can inadvertently kink or tear superficial draining veins, and retractors can be bumped by passing instruments or resting hands. With attention focused at the cavity’s depths, occlusions of superficial draining veins may go unrecognized with bleeding consequences. Secondary draining veins that limit deep exposure are safe to sacrifice at this stage. Deep hematomas should be found and evacuated to open up the deep plane. Perforating artery supply differs from cortical artery supply. Perforators traverse deep parenchyma rather than cortical surfaces, and are smaller in caliber, thin walled, fragile, and difficult to cauterize. Bleeding can draw the neurosurgeon into critical neural structures where visualization is compromised by overlying nidus, and tolerance to bleeding and electrocautery is low. Whether from lenticulostriates to a basal ganglial AVM, insular perforators to an insular AVM, thalamoperforators to a thalamic AVM, or basilar perforators to a pontine AVM, bleeding is brisk and hazardous with consequences for the surgical outcomes. The answer to the deep perforator is, simply, the AVM microclip. Although cautery typically explodes the perforator and initiates a torrent, the microclip shuts it effectively and bloodlessly. It is almost not worth trying cautery because a bleeding perforator is so much more difficult to control. If it does bleed, the perforator is controlled by drying the field with suction on a thin cottonoid, using dynamic retraction to see into remote corners, chasing the bleeder through white matter, freeing a short perforator segment proximal to the bleeding point, drawing it into the sucker to steady it, and finally applying the microclip (Fig. 7.1). After microclip application and flow arrest, perforators are more responsive to cautery. They are coagulated along a segment rather than a point, and divided. Bleeding from the distal stump might require a second microclip on the nidal side if uncontrolled with cautery. Perforators are so delicate that slight manipulation or even microclip application can induce bleeding, in which case more dissection proximally exposes the perforator for additional microclips. Progressive proximal dissection leads to larger perforators with less branches and more coagulability, but intrudes deeper into white matter tracts. The decision where to cross a tangle of perforators must balance the ease of a more proximal interruption with proximity to eloquence. Fig. 7.1 Steps in controlling a deep perforating artery: (a) drying the field with suction on a thin cottonoid; (b) attempting to coagulate it with bipolar cautery; (c) proximal dissection into white matter to free a segment of artery proximal to the bleeding point, sometimes drawing it into the sucker; and (d) applying the microclip. The battle with perforators, fortunately, is usually brief. Perforator supply is typically limited to less than five arteries, and in some cases may be just one. They coalesce at the ependymal tip or the AVM’s extension into white matter. They must be controlled one by one and never packed. Thin cottonoids are very helpful to protect brain, apply suction, retract against, and define the surgical plane. However, too many cottonoids will create a tangle of strings. Perforators are difficult to see angiographically because they are small, few, and overshadowed by larger inundating arteries. However, their presence should never surprise, even with cortical, lobar, or cerebellar AVMs. Any AVM that breaches white matter can attract these perforating arteries. Furthermore, as insignificant as they might appear angiographically, they probably increase their shunt flow during the course of circumdissection as other inputs are throttled. Perforators are typically the last of the arterial supply, and I often notice that a draining vein that was bright red at the beginning of a perforator skirmish is dark blue at the end. Choroidal supply is generally more cooperative than perforator supply because these arteries are thicker, more coagulable, and floating freely in the ventricle. Choroidal arteries run in tela choroidea and choroid plexus, making them far more visible than parenchymal perforators buried in white matter. Ventricles open widely; transcortically, transcallosally, or through a choroidal fissure; and offer anatomic orientation, working space, and proximal control. Therefore, even though they are deep, choroidal arteries are like an artery in a cistern or subarachnoid space that can be identified with clear landmarks and controlled. Other choroidal arteries may intersect the AVM diametrically opposite to the AVM. These feeding arteries are poorly visualized, lack proximal control, and are difficult to access. These difficulties are encountered with bulkier AVMs that have a large ependymal surface rather than just an ependymal tip, and a network of choroidal supply from the anterior choroidal artery (AChA), medial posterior choroidal artery (mPChA), and lateral posterior choroidal artery (lPChA), rather than a single input. Bleeding from diametrically opposite arteries hides from view, causing unchecked intraventricular hemorrhage and, in the extreme, outward brain herniation. Microclips can be useful with choroidal arteries when access behind a bulky AVM makes them difficult to coagulate. Fig. 7.2 Anatomy of an AVM’s ependymal tip. (a) Ependymal dissection that does not enter the ventricle may truncate the AVM and (b) leave a remnant supplied by choroidal arteries and drained by ependymal veins with persistent arteriovenous shunting. (c) Additional dissection into the ventricle surrounds the AVM’s tip, confirms that the draining vein is dark, and completes the circumdissection.
The Dark Side
Perforating Artery Supply
Choroidal Artery Supply
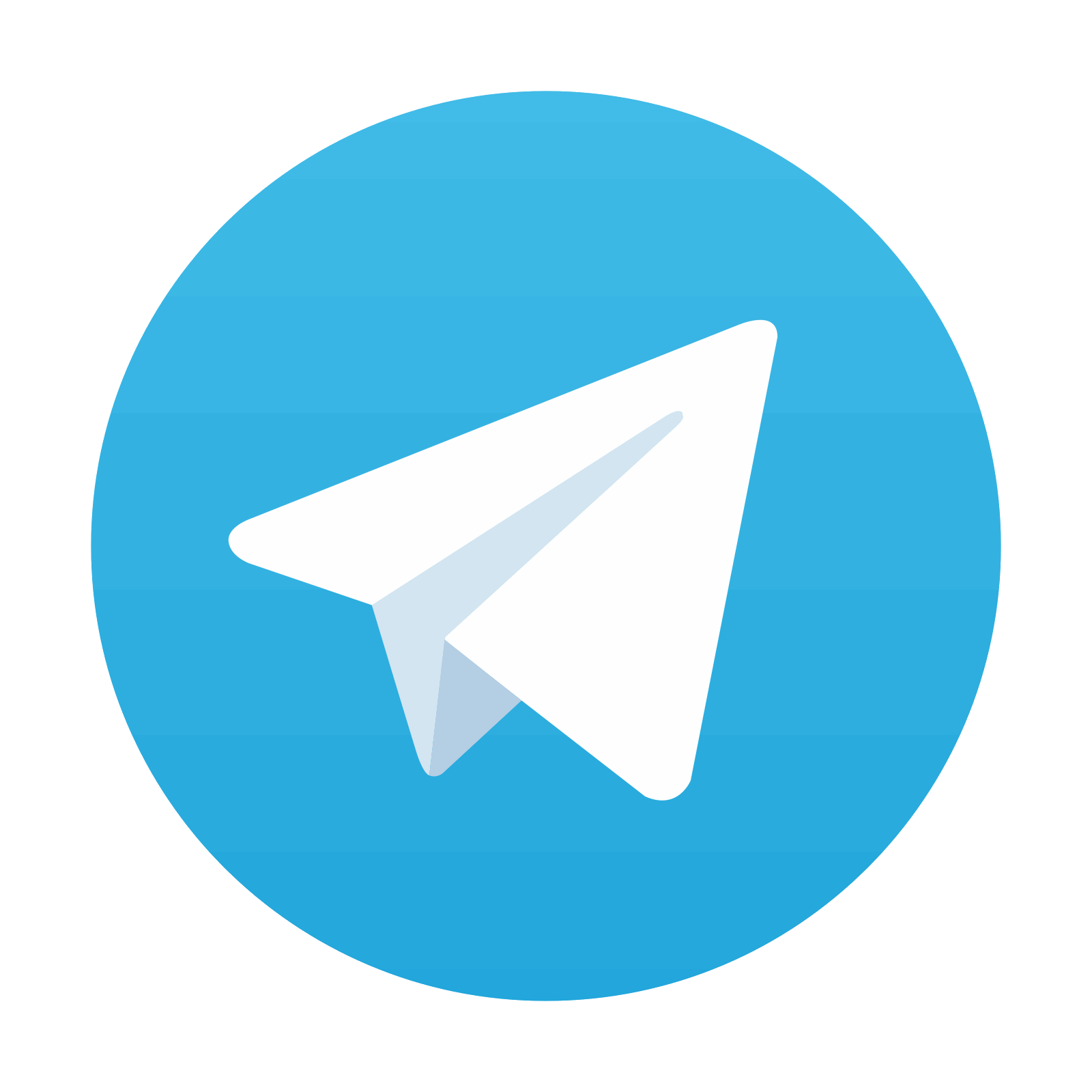
Stay updated, free articles. Join our Telegram channel

Full access? Get Clinical Tree
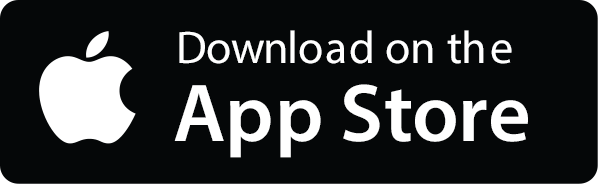
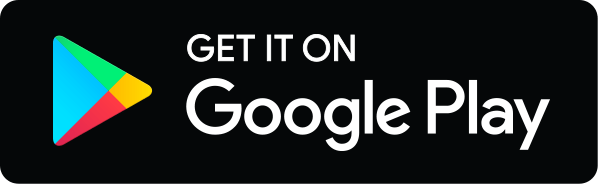