For example, what is the probability that our snoring patient has sleep apnea? We know from literature that about 90 % of patients with obstructive sleep apnea snore (sensibility). We also know that the prevalence of OSAS among men is about 4 % and that about 50 % of adult men, similar to our patient, snore at least sometimes. Hence, the probability of OSAS in our patient is (0.9 × 0.04)/0.5 = 0.072 = 7.2 %.
Mortality rates. Mortality rate or death rate is the proportion of a population who die of a defined cause. The numerator is the number of persons dying, and the denominator is the total population in which the deaths occurred. The unit of time may be one calendar year or a longer period of time. In order to avoid bias arising from disproportions in age, gender, or race between the general (denominator; reference) population and the study population, the data are standardized. The indirect method is more popular than the direct method of standardization. As a result, standardized mortality ratios can be given. In long-term follow-up studies survival, mortality and also morbidity figures may be computed using different survival analyses.
Risk ratio and odds ratio. Risk is often used to describe rate of occurrence of a symptom or disease. Risk refers to probability that an event will occur within a defined period of time. A risk factor, or determinant of risk, is a factor or exposure that increases the probability of occurrence of a specified outcome. A risk factor may or may not be in causal relationship with the outcome.
Risk ratio (RR) or relative risk (also rate ratio) is the ratio of the probability (risk) of getting a disease (outcome) in a risk group (exposed subjects) divided by the probability (risk) of getting the same disease (outcome) in a reference group (non-exposed subjects). In the case of a rare disease, risk ratio approximates the odds ratio (OR). The OR is defined as the odds that an outcome will occur given a particular exposure, compared to the odds of the outcome occurring in the absence of that exposure. Instead of risk ratio, the odds ratio (cross-product ratio) and its 95 % confidence limits are widely used in epidemiological studies. Whenever possible (if meaningful prevalence or incidences are available), calculation of rate ratios (relative risks) is recommended because RRs are often easier to understand than ORs. OR always overestimates the RR. In addition, ORs are often misinterpreted as if they were equivalent to RRs [27]. If the lower limit of the 95 % confidence interval is greater than 1, the rate ratio is “statistically significant” with a P value less than 0.05, that is, the studied exposure is a “risk factor for the specified outcome.” Similarly, if the upper 95 % confidence limit of a rate ratio is less than 1, the factor is “protective.”
Normality and use of medians. What does “normal” mean in epidemiology? Sir Ronald Fisher regarded 95 % of the inner values of a distribution as common and the remaining 5 % as significantly uncommon. The outer 5 % of values is traditionally considered abnormal, but statistical or epidemiological theory does not support this view [3]. According to Feinstein, “Fisher’s proposed boundary of uncommon occurrences was intended for inferential decisions about P values, not for descriptive decisions about normality. Nevertheless, after years of exposure to 0.05 as the magic level of stochastic significance, many clinicians have become thoroughly conditioned to accept the same boundary marker for abnormality” [3]. Many laboratories now use the term “customary range” instead of normal range. This term is more appropriate. It is also good for patients whose laboratory values are outside the customary range, because the values may be without any clinical significance even if they are out of the customary range.
Biological data do not always fit Gaussian normal curve. The distributions are often significantly skewed or bimodal. On such occasions, the usual mean ±2 standard deviations tactics is not appropriate. Unfortunately, one can still find that it is used in medical literature even if the data are not normally distributed. For example, one could read that the mean (± standard deviation) weight of children was 10 ± 12 kg. How does a child look if his/her weight is minus 2 kg (10 − 12 = −2)? One can have negative cholesterol or negative apnea index values, etc, if one gives means and standard deviations alone. Giving the confidence interval and range gives more information. In biological sciences and medicine, use of medians (the “middle value”) and percentiles, even without means, is often better than means and standard deviations alone.
Median is easy to calculate and it describes the population better than the mean. It divides the population in two equal parts. To find the inner 95 % of the data, we need only to locate the 2.5 percentile point and the 97.5 percentile point. The procedure is simple, and by using it, we will never encounter impossible ranges.
Measures of effect size. Different measures may be used to have an idea about results of interventions and clinical trials. In particular, three measures are commonly used in evidence-based meta-analytical studies: relative risk reduction (RRR), absolute risk reduction (ARR), and the number needed to treat (NNT).
Let us cite two examples. (1) Suppose that in a prospective follow-up study, 10 % of subjects treated with CPAP had a new cardiovascular event versus 20 % among the non-treated. These rates are also called risks of having a defined event; in other words, risk can be thought of as the rate of a defined outcome. (2) Suppose that in a randomized clinical trial on restless legs syndrome, 70 % of those with the active drug had significant amelioration of their symptoms versus 30 % in the placebo control group. In the same study, 1 % of those with active treatment and 0.3 % of those with placebo had hallucinations as an adverse effect.
Relative risk reduction. Relative risk measures how much the risk is reduced in the treatment group compared to the control group. In the first example above, 20 % of the control had a cardiovascular event compared to 10 % among those treated with CPAP. The CPAP treatment group had a 50 % (0.5) relative risk reduction. The formula is as follows: RRR = (CER − EER)/CER, where CER is the control group event rate and EER is the experimental group event rate. In the second example, the relative risk reduction of having hallucinations when using a placebo instead of the experimental treatment is 70 %, i.e., (1 − 0.3)/1. As we can see, the RRR is not a good indicator if the occurrence of an event is rare.
Absolute risk reduction. Absolute risk reduction is the absolute difference in outcome rates between the control and treatment groups, i.e., ARR = CER − EER. The absolute risk reduction does not confound the effect size with the baseline risk. In the first example above, ARR is 10 % (20 − 10% = 10 %). In the second example, ARR for hallucinations is 0.7 % (1 − 0.3) when using placebo. This figure is very small and easier to interpret than the large and not clinically significant relative risk reduction of 70 %. The ARR for having amelioration of symptoms is in this example, 70 – 30 % = 40 %.
Number needed to treat. Because the ARR is sometimes difficult to interpret, better indicators are needed. The number of patients needed to treat (NNT) in order to find a significant effect (e.g., cure and significant amelioration of symptoms) is a good indicator of a treatment effect [28]. The number needed to treat is simply another way to express absolute risk reduction. NNT = 1/ARR and it is the number of patients that would need to be treated to prevent one additional bad outcome. For the example of CPAP above, the NNT is 10 (1/0.1) and for the second RLS example, the NNT is 1/0.4 = 2.5. Thus, in the first example, 10 need to be treated and in the second example, only 3 patients. The number needed to have hallucinations, i.e., the number needed to harm (NNH) is in the second example 143 (1/0.007 = 142.8). If we compare 3 patients to 143 patients, we can clearly see that the benefits are much larger than harms in this respect. Of course, one must take all other adverse effects into account when the NNH is estimated. If the NNT is still larger than the NNH, then the treatment could be used. It is interesting to note already at this point that NNT of chronic insomniac patients with sedative hypnotics in a meta-analytic study of Glass et al. was 13 but the NNH was more than twice smaller than that number (NNH = 6). This means that the probability of doing harm to a patient was larger than that for doing good [29].
The NNT can be converted to an NNT for a given patient by estimating the patient’s susceptibility relative to an average control patient in a trial report and dividing the reported NNT by this fraction F (i.e., susceptibility). For example, in the second scenario above, if a physician’s 80-year-old patient is judged to be three times more susceptible as the average control patient to have hallucinations, then F = 3 and NNT/F = 143/3 = about 48. This means that one could still think about treating the patient with that drug, supposing that there is significant evidence about its effectiveness in old patients above 80 years of age. The NNT can be computed also from the odds ratio and control group rate of events; 95 % confidence limits for the NNT should also be given. Different tables and formulas exist in many statistical packages and also in the Internet, e.g., by searching “number needed to treat” using Google.
Effect size. Effect size (ES) is a commonly used index to measure the magnitude of a treatment effect. It is important to note that effect size indices are not statistical tests. Unlike significance tests, they are independent of sample size. ES measures are used especially in evidence-based meta-analytical studies that summarize the findings from a specific topic. Effect size (ampleur d’effet in French) is computed most commonly as the standardized difference between two group means. Originally, Cohen defined effect size d as the difference between the means, M 1 − M 2, divided by standard deviation, s, of either group 1 or group 2, i.e., effect size d = (M 1 − M 2)/s.
This is an approximation of the theoretical formula for the standardized difference between two groups where the denominator should be the standard deviation of the population from which the groups are sampled. If it is unknown, it can be estimated in different ways. If the standard deviations of the groups are approximately the same, it can be assumed that they are estimating common population s. In this case, they can be pooled (s pooled) to calculate Cohen’s d index of effect size d = (M 1 − M 2)/s pooled [30, 31]. Usually, the subtraction of the means between the intervention (experimental) group and control group is done so that a positive difference means improvement and negative difference means deterioration. The pooled standard deviation is found as the root mean square of the two standard deviations. Modern statistical programs usually automatically compute also the pooled standard deviations, that is, the pooled standard deviation is the square root of the average of the squared standard deviations. When the two standard deviations are similar, the root mean square will not differ much from the simple average of the two variances.
Epidemiology of Sleep Disorders
The oldest epidemiological studies about sleep length are from the end of the eighteenth century. Among the most respected studies were those by Clement Dukes from England about the need of sleep of young children. Other well-known early studies are those by Hertel from Denmark, Bernhard from Germany, and Claparède from France [32, 33]. About 80–100 years ago, it was recommended that young children should sleep 10.5–13.5 h, 15-year-olds should sleep 9–10 h, and adults should sleep between 7 h 25 min and 8 h 23 min [34, 35], which does not differ significantly from present day self-reported figures of healthy adults having an average sleep length between 7 and 8 h. Women slept a little bit longer than men except in the case of mentally retarded subjects when the situation was reversed [36, 37].
Sleep disturbances increased with age as they do at present days. In the Laird’s study [35] from 1931, at age 25, about 10 % of men were not satisfied with their sleep. At age 95, everybody reported some wakefulness each night. In the same study, more than 70 % of men reported some difficulty in going to sleep, and more than 40 % reported nightly awakenings. Different methods were used to help one to sleep. Reading was used by 25 %, and 18 % used relaxation techniques. Three percent of men used drugs to help them sleep. Two percent used alcohol to help them sleep [35]. The latter two figures differ significantly from those of present days.
Many epidemiological studies about sleeping habits have been done since 1960. In these studies, the average self-reported sleep duration varied between 7 and 8 h. In a questionnaire survey, 7.4 % of 1278 University of Florida students reported less than 6.5 h of night sleep, and 13.4 % reported more than 8.5 h [38]. In Scotland, 2446 subjects aged over 15 were inquired. Of the older subjects in the age group 65–74 years, 18 % complained of waking up before 5 a.m. The percentage decreased to 12 % after age of 75. Disturbed sleep was reported by less than 10 % of men aged 15–64. In the age group 65–74, disturbed sleep was a complaint in 25 % of men. In women, the respective percentage was 43 % [39]. In one classic study from 1979, Bixler et al. determined the prevalence of sleep disorders among 1006 households in the Los Angles metropolitan area [40]. The prevalence of past or current insomnia was 42.5 %. The prevalence of current insomnia was 32.2 %, and 7.1 % complained of excessive sleep.
Insomnia
The epidemiology of insomnia and insomnia-related symptoms is a bit blurred because of large variance in methods and case definitions used in different studies [41]. The view on insomnia is also changing in itself. Traditionally, insomnia was considered as “integrated into” psychiatric disorders. However, this view has changed. The current psychiatric DSM 5 as well as the 3rd International Classification of Sleep Disorders uses the term “insomnia disorder” as a distinction to “insomnia” as a symptom [42, 43].
Taking existing evidence together, about 2–18 % of adults usually complain of clinically significant persistent insomnia depending on the definition of insomnia and the population. Several studies suggest relatively consistently that about 10 % of middle-aged adults suffer from long-standing insomnia [40, 44–59]. Transient insomnia- and occasional insomnia-related symptoms are very common but prevalence figures vary greatly between studies from about 20–50 % [40, 55, 57, 60–64].
Chronic insomnia is often (in 35–69 % of cases) associated with psychiatric conditions. Most common comorbid condition in chronic insomnia is depressive disorder, which has been reported to be present in about 40–50 % of chronic insomniacs [49]. In one study, 93 % of depressed psychiatric inpatients had insomnia [65]. Among treatment seeking veterans of the 13 separate mental health diagnoses evaluated, all except dementia and schizophrenia were strongly associated with insomnia [41].
Insomnia and insomnia-related symptoms are associated with increasing age, at least up to the age of about 65 [53] and female gender particularly during the midlife years [66–68] being 1.4–2 times more common in women than in men.
About one-third of subjects older than 65 years have chronic insomnia. At very old age, the figures may be lower [46, 69]. In an Australian study, insomnia was persistent in 16.2 % of the community-dwelling population and in 12.2 % of institutional residents. Altogether 14.5 % of the elderly subjects living in the community used hypnotics regularly while the corresponding percentage was 39.7 among the institutionalized subjects [46]. However, while sleep problems may increase with age, active complaints to the physician may decrease with age [41]. Comorbidity and psychosocial influences are strong modifying factors underlying the association of insomnia and increasing age. Highest rates of self-reported insomnia symptoms have been reported for 18–24-year-old and 50–59-year-old men and women [70].
Aging process per se may not be the primary cause of insomnia among elderly people [71]. A 3-year longitudinal study [72] among 6899 men and women aged 65 and older suggested an annual incidence rate of insomnia approximately 5 %. Incident insomnia was associated with depressed mood, respiratory symptoms, fair to poor perceived health, and physical disability. Only 7 % of the incident cases of insomnia occurred in the absence of associated risk factors. Of the nearly 2000 survivors with chronic insomnia at baseline, almost 50 % did not report any symptoms after 3 years. The authors interpreted their results as not giving support to a model of incident insomnia caused by the aging process per se [72].
Among people complaining frequent or chronic insomnia and/or insomnia-related symptoms, the use of sleep medication is very common. It has been estimated that about 57–60 % of individuals complaining of frequent or chronic insomnia use sleep medication [73]. The association between medication use and insomnia is independent of other comorbidities [73]. The healthcare system is oriented to treat insomnia pharmacologically. Up to 60 % of adults seeking treatment for insomnia are prescribed sedative and/or hypnotic medications [73, 74]. This is reflected in recent prevalence figures of hypnotic medication use of 6–10 % among adult US population [75]. In US outpatient care, the number of office visits with insomnia increased by 13 % from 4.9 million visits in 1999 to 5.5 million visits in 2010. At the same time, however, the number of prescriptions for any sleep medication increased by 293 % from 5.3 in 1999 to 20.8 million in 2010 [76]. Somewhat older prevalence estimates among the general population have varied between 4 and 11 % [77–79]. Among 65–year-old or older, 9–15 % have been reported to use sleep medication nightly [80]. The use of hypnotic medication among insomniacs seems to be long standing [78]. The use of hypnotics in particular is associated with the use of psychotropic medication in general resulting in different multiuse combinations of hypnotics and sedatives with anxiolytics and psychotropic drugs [73].
Recently some studies have consistently reported that especially occasional self-reported insomnia symptoms, use of sleep medication, and perhaps also chronic insomnia in some countries show increasing long-term prevalence figures in the general adult population [55, 81–83]. An analogous trend was found also among adolescents in Finland [84]. In addition, the number and percentage of US outpatient office visits due to sleep-related problems and prescriptions for a sleep medication have also significantly increased [76]. The increasing trend of pharmacological treatment for insomnia has prompted warnings against possible overuse of medications among insomniacs [74]. In line with increasing prevalence figures, there is also an ongoing shift in prescribing sleep medication. There is some evidence that physicians have started to replace traditional hypnotics (benzodiazepines and their derivatives) by small doses of antidepressants and antipsychotics [85–87]. Theoretically, the latter medications may be more physiological as they may increase slow-wave sleep though their action on histamine-1 receptors [88]. Long-term epidemiological surveys of their benefits are, however, missing [89]. Self-reported insomnia-related symptoms have complicated underlying associations with individuals’ socioeconomic status, general well-being, and quality of life as well as with different comorbidities [90, 91]. Different kind of information bias may complicate interpretation of various studies. However, it seems to be clear that something is happening in our time to which peoples’ self-reported sleep quality is responding.
Efforts and official initiatives to reduce hypnotic use have been largely unsuccessful in healthcare system [92]. In particular in older individuals, adverse effects on cognitive and psychomotor functioning may result in increased risk of falls and traffic accidents [93]. Also, tolerance and dependence may develop. However, the interpretation of the established epidemiologic association between use of hypnotics and increased mortality has remained controversial. The association was reported first in the American Cancer Prevention Study [94]. Later, the follow-up data were reanalyzed and the “use of sleeping pills” and “tranquilizers” “often” associated with mortality with 1.5 hazard ratio when compared with non-users [66]. It is evident that “sleeping pills” at that time were different compounds and potentially more dangerous than those used today. However, the association has been later replicated approximately 20 times in different countries [75]. The association has been confirmed as regards total mortality [95, 96] and several specific causes of death such as cancer [75, 97, 98] and cardiovascular disease [99], among others. On the other hand, the strength of the association has usually not been especially strong. Median hazard associated with hypnotics among 24 studies was found to be <1.5 and only in four cases >2.0 after adjustments [100]. Negative findings have also been reported [80, 101, 102]. Users and non-users differ from each other in several important ways as regards the comorbid conditions associated with insomnia itself, and it is practically impossible to control for all potential confounders. This makes the statistical analyses (or more accurately statistical inferences) of large observational studies complicated and difficult. The situation has created currently ongoing debate whether the association is causal or caused by confounding [103–106]. In any case, the clinicians should follow the principle of caution and the guidelines for temporary use of hypnotics.
Chronotype and insomnia. Interindividual variance in individuals’ circadian preferences of the timing of their daily behaviors underly their phenotypical differences along a characteristic called chronotype. In parallel, different chronotypes differ in involuntary timing of physiological functions such as core body temperature, blood pressure, and hormonal secretion. Based on these behavioral and physiological differences, individuals can be classified into earlier timed morning types, the intermediate types, and the later timed evening types [107–110].
Epidemiology of chronotypes is new but currently rapidly evolving field of sleep epidemiology, although still in its beginning. Only few large epidemiological studies on chronotype have been so far published. Among the Finnish general adult population, the prevalence of clear morning types has been estimated to vary approximately from 29 to 47 %, and clear evening types from approximately 10–12 % [111–113]. A New Zealand study estimated approximately 10 % of 30–49-year-old population to be “definitely” morning types and approximately 40 % to be “moderately” morning types, 7 % “definitely” evening types and 5 % “moderately” evening types [114]. Evening type has been repeatedly shown to be associated with different health risks, for example, total mortality [113], cardiovascular disease and type 2 diabetes [115, 116], and depression [117, 118]. Of note, evening type is also associated with chronic insomnia complaints 2–3 times more often than morning type as well as use of sleep medication [112, 119].
Seasonal differences of insomnia. Occurrence of insomnia may vary depending on the season of the year. The results from the Nordic countries agree with each other [52, 120]. In Northern Norway, 41.7 % of women and 29.9 % of men had occasional insomnia. In general, complaints of insomnia are more common during winter than during other times of the year [121]. The reasons for this cannot be explained by the season (darker, colder climate, more rain, or snow) itself. Work and different lifestyle factors probably explain most of the variance, but we lack good evidence. In the Tromsø study, occurrence of insomnia during summer (summer insomnia) decreased with age, whereas the other seasonal types of insomnia increased with age [120]. Individuals also differ in their sensitivity or reactivity to seasons. Among high or moderate seasonality individuals, sleep problems suggesting insomnia as well as sleepiness have been shown to be more prevalent than among individuals of low seasonality group [122]. In clinical form, this is reflected in the association between depressive symptoms and seasons among patients suffering from seasonal affective disorder (SAD).
Insomnia in different occupations. Occurrence of sleeping problems varies by occupation and working times [123–126]. In a questionnaire survey of 6268 adults representing 40 different public sector occupations, 18.9 % of bus drivers complained of difficulty falling asleep. Among male directors and male physicians, the respective percentages were 3.7 and 4.9 %. Disturbed nocturnal sleep was complained most often by male laborers (28.1 % waking up at least three times a night) and female cleaners (26.6 %). Disturbed nocturnal sleep was rare among male physicians (1.6 %), male directors (7.4 %), female head nurses (8.9 %), and female social workers (9.4 %) [126]. The association of sleep and type of occupation are partly explained by working conditions, socioeconomic position, income, and probability of possible future unemployment [127, 128]. Accordingly, prevalence figures of insomnia-related symptoms are associated with great shifts in society’s economic situation [129].
Psychiatric Disorders and Insomnia
Insomnia is associated with psychiatric disorders such as depression, anxiety disorders, alcohol dependence, and schizophrenia [130]. Traditionally, insomnia was considered a symptom of psychopathology. Until recently, insomnia was classified as a primary disorder if it was not related to mental disorders, and if it was, a diagnosis of secondary insomnia was given. These were the two most common DSM-IV insomnia diagnoses [131]. The differential diagnosis was, however, sometimes difficult. For example, in one study, 216 patients with insomnia were interviewed by one sleep specialist and one non-sleep specialist. By using DSM-IV criteria, 99 (46 %) were diagnosed as having insomnia related to mental disorders, and 48 (22 %) were diagnosed as having primary insomnia. A psychiatric disorder was rated as a contributing factor for 77 % of patients with a first diagnosis of primary insomnia [132]. It has been suggested that “comorbid” insomnia may be a more appropriate term than “secondary” insomnia [133]. Recently, DSM-V has divested the concept of primary/secondary insomnia and instead introduced “insomnia disorder” as the main diagnostic category for insomnia, leaving it possible to determine whether or not insomnia is comorbid with a psychiatric or somatic disorder [42]. In a large US community survey, the prevalence of insomnia without psychiatric comorbidity was 4.9 % [134]. Among those with comorbid insomnia in the past year, 25 % had major depression, 19 % abused alcohol, 12 % had dysthymia, 9 % had panic disorder, 8 % abused drugs, 8 % had schizophrenia, and 2 % had somatization disorder [134]. In a World Health Organization (WHO) collaborative study, 25,916 primary healthcare patients were evaluated. Sleep problems were present in 27 % of the patients. Of the patients with insomnia, 51 % had a diagnosis of ICD-10 mental disorder, mainly depression or anxiety, abuse of alcohol, or a combination of different psychiatric disorders. 40 % of insomniacs reported using alcohol and over-the-counter medications to help them to sleep [135].
The relationship between psychiatric disorders and sleep is complex with bidirectional causation [136]. Insomnia is not only a symptom of psychiatric disorder but also a predictor of future incident depressive period, PTSD, and substance use. [10, 133, 137–140]. In a recent longitudinal study in 9683 young Australian women who were surveyed in 2000, 2003, 2006, and 2009, a new-onset depression and anxiety were predicted at each subsequent survey with two 2- to 4-fold OR estimates among those who reported sleeping difficulties “often” in 2000 [141]. In another study in 1584 adult patients seeking help from a private sleep medical center, suicidal ideation was consistently associated with different sleep complaints even when depressive symptoms were controlled for [142]. However, one placebo-controlled study in insomnia patients concluded that it may be the use of hypnotics, rather than insomnia itself, which is causal risk factor of future depression [143].
The overlapping of insomnia and psychiatric disorders results also in large mixed psychotropic drug use among insomniacs. In one telephone survey, it was estimated that among non-institutionalized French population 53 % of insomnia sufferers used at least one drug. Approximately, 20 % used hypnotics, 37 % anxiolytics, 7 % antidepressants, and 3 % other psychotropic drug [49]. Consequently, there is a potential risk of mixed drug overuse among insomniacs. Given that insomnia is associated with alcohol dependency one should remember that alcohol may have serious interactions with psychotropics, especially with hypnotics and sedatives. Among alcohol dependent patients, insomnia may also increase alcohol-related adverse psychosocial consequences [144].
Different stress factors belonging to more or less normal everyday life are also associated with insomnia and insomnia-related symptoms. Unemployment, being unmarried, separated, or widowed associate with higher prevalence of insomnia complaints in Japan as well as in other countries [145]. Different psychological complaints, psychological stress, symptoms of work-related stress, and mental exhaustion are associated with high prevalence of insomnia [47, 52, 77, 131, 146–149]. Simple methods, such as the five-item version of the Mental Health Index and some other questions, may be used to effectively screen workers with mental health and sleep problems [145, 150, 151].
Insomnia Among Patients with Neurological and Other Somatic Diseases
Parkinson’s disease and dementia. Many neurological disorders are associated with disturbed sleep and insomnia. Parkinson’s disease [152–161] and dementia [162–168] are typical examples. Sleep disorders occur in over 70 % of patients with idiopathic Parkinson’s disease (PD), adversely affecting their quality of life. Among patients with Parkinson’s disease, sleep disruption takes the form of sleep fragmentation with frequent and prolonged awakenings and daytime sleepiness. Nocturia, difficulty in turning over in bed, painful leg cramps, vivid dreams and/or nightmares, back pain, limb/facial dystonia, and leg jerks are the main causes of nocturnal awakenings in PD patients. Sleep disturbance gradually worsens with disease progression suggesting that it is related to the severity of the disease. Sleep disturbance may also be a complication of chronic levodopa therapy. In a survey of 100 PD patients, significant sleep complaints were found in 74 %. Sleep complaints were unrelated to patient age and the duration of disease but increased in prevalence with longer periods of levodopa therapy. Sleep abnormalities tended to increase in severity with continued treatment of PD. Dyskinetic side effects and on–off syndrome occur as well in patients with or without sleep complaints, but up to 98 % of patients experiencing psychiatric side effects also report sleep disruption [158]. A correlation with sleep disturbance and severity of PD exists [159–161, 169]. In an Indian case–control study, 149 PD patients and 115 age-matched healthy controls were asked about sleep disturbances using a questionnaire. Sleep problems were seen in 42 % of PD patients and 12 % of controls. Sleep disturbances included insomnia in 32 % versus 5 % of controls, nightmares 32 % versus 5 %, and excessive daytime sleepiness by 15 % versus 6 %. All sleep complaints were statistically more common in PD patients compared to controls and correlated with increased severity of the disease [154].
In a follow-up study, 142 of the initial 231 patients were re-evaluated after 4 years and 89 patients after 8 years [157]. Complaints of insomnia remained almost constant while problems related to turning in bed and vivid dreaming or nightmares increased during the follow-up. Insomnia was present in 54–60 % of the patients at each of the three study visits. Insomnia was related to disease duration, depression, and female sex [157].
Stroke and insomnia. Patients with stroke complain often of insomnia, and disturbed sleep may also be a risk factor of stroke or an indicator of other processes that are related to stroke. Post-stroke depression is one such factor, but it does not explain everything. A sample of 277 stroke patients aged 55–85 had a psychiatric evaluation of 3–4 months after ischemic stroke. 56.7 % reported some type of insomnia complaint and 37.5 % fulfilled the DSM-IV criteria of insomnia. In 38.6 %, insomnia complaint had started before the stroke, and in 18.1 %, it started after occurrence of the stroke. Insomnia complaints correlated with increased disability, cognitive decline, anxiety, and increased use of psychotropic drugs [170]. In South Wales, 1986 men aged 55–69 completed a questionnaire on sleep patterns with help from their partners. They were asked about symptoms of disturbed sleep including insomnia, snoring, restless legs, obstructive sleep apnea, and daytime sleepiness (Table 28.1). During 10 years of follow-up, 107 men experienced an ischemic stroke and 213 had an ischemic heart disease event. About 1/3 of the men reported at least one symptom suggestive of sleep disturbance, and 1/3 reported daytime sleepiness. Compared with men who reported no such symptoms, the age-adjusted relative risk of an ischemic stroke was significantly increased in men with any sleep disturbance. The strongest association was with symptoms indicating sleep apnea (OR 1.97; 1.26–3.09) [171].
Table 28.1
Snoring as a risk factor of stroke
Study | Population | Methods; outcome | Stratification/adjustment | Type of snoring | OR (95 % CI) |
---|---|---|---|---|---|
Partinen and Palomäki [419] | 50 male stroke patients and 100 hospital controls | Case–control, personal interview, neurological examination, CT/MRI; stroke versus others | Age, gender (men), BMI | Habitual snoring | 10.3 (3.5–30.1) |
Often or always snoring | 2.8 (1.3–5.8) | ||||
Koskenvuo et al. [406] | General population 4388 men, 40–69 years | Cohort study, 3 years follow-up, questionnaire, registry data; incidence of stroke or IHD | Age, gender (men), BMI, smoking, alcohol, HT | Often or almost | 2.08 (1.5–3.77) |
Always snoring | (Stroke or IHD) | ||||
Palomäki [424] | 177 Stroke patients and 177 hospital control patients | Case–control, questionnaire, neurological examination, CT/MRI; stroke versus others | Age, gender, alcohol, HT, IHD | Often or always snoring | 2.13 (1.29–3.52) |
326 IHD/stroke pat. and 345 community | Case–control, personal interview, neurological examination, CT/MRI; stroke versus others | Age, gender, BMI, smoking, alcohol, IHD, HT, AF, DM | Often or almost snoring | 3.2 (2.3–4.4) adj for age and gender 1.7 (1.3–2.2) adj for all factors | |
Smirne et al. [420] | 164 Stroke patients and 330 hospital control patients | Case–control, personal interview, neurological examination, CT/MRI; stroke versus others | Age, gender, BMI, smoking, alcohol, HT, DM, dyslipidemia | Often or always snoring | 1.86 (1.20–2.87) |
Jennum et al. [416] | 804 70-year-old followed for 6 years | Cohort of 70-year-old men and women, snoring history and other information from medical records | Age, gender, BMI, smoking, alcohol, HT, social class, lipids, glucose, catechol | Often or always snoring | 1.8 (1.1–3.6) |
Neau et al. [417] | 133 Stroke patients, and 133 non-hospital control patients | Case–control, personal interview, spouse present, neurological examination, CT/MRI, stroke versus others | Age, gender, BMI, DM, hypertension, cardiacarrhythmia | Habitual snoring | 2.93 (1.28–6.75) |
Hu et al. [283] | 71,779 Female nurses, 40–65 years | Cohort study, 8 years follow-up, questionnaire, registry data; incident stroke versus no stroke | Age, smoking, BMI, hypertension, DM, hypercholesterolemia, familial IHD history and other covariates | Snoring regularly | 1.88 (1.29–2.74) adjusted for age 1.35 (0.91–1.99) adjusted for all |
Occasional snoring | 1.42 (1.07–1.89) adjusted for all |
Other neurological and somatic disorders. Most subjects with mental retardation complain of some types of sleep disturbance [172, 173]. Insomnia is frequent among many other somatic disorders including different respiratory diseases [147, 174]. In a study by Dodge et al., the prevalence of insomnia was 31.8–52.4 % among adults with cough, dyspnea, or wheezing. Among adults without respiratory symptoms, the prevalence was about 26 % [174]. In an older Swedish study, 18.8 % of men with obstructive pulmonary disease suffered from difficulties in maintaining sleep (DMS) and 12.4 % complained of excessive daytime sleepiness. Among diabetic men, DMS was complained by 21.9 % and difficulty initiating sleep was complained by 21.1 % [175]. In a large US telephone survey, nocturnal awakenings were complained by 41.5 % of patients with gastroesophageal reflux, 38.6 % of heart disease patients, and 27–38 % of patients with other somatic diseases [176].
Is Anticipation of Coming Health-Related Events Possible with Follow-up of Sleeping Pattern and Sleep/Wake Rhythm?
As mentioned above (see “psychiatric disorders and insomnia”), insomnia has been repeatedly shown to be a predictor of future incident depressive period, PTSD, and substance use. There is also increasing evidence that self-reported sleep duration is a statistically independent predictor of all-cause and cardiovascular mortality, and morbidity in large epidemiological follow-up studies in recent meta-analyses [177–179]. The association is U-shaped meaning that often both extreme ends of the self-reported sleep duration distribution are associated with increased risk. However, there is some variation among the results. For example, a U-shaped association was confirmed in some studies [180, 181], only among women but not in men [182], or only among men but not in women [183, 184]. In one study, only short sleep was associated with increased risk of stroke [185], and in some studies mainly long sleep was associated with risk of stroke [178]. A recent study suggested that use of sleep medication may be a strong modifier of the association between short sleep and health outcomes [186]. Also, negative findings have been reported as regards sleep duration and future health [187, 188]. One reason for the inconsistencies among findings may be that the interpretation of the association of self-reported sleep duration and adverse health outcomes is complicated [189, 190], and may be different in different populations or cultures.
Sleep Length; Natural Short and Long Sleepers
As described above, self-reported sleep duration is a statistical predictor of several detrimental future health outcomes. A U-shaped association of self-reported sleep duration and health outcomes suggests that among the general population there are two risk groups showing deviation from the population mean sleep duration. Within these groups, sleep-related health risks are supposed to be increased. The prevalence figure of these risk groups varies depending on the cutoff points used to determine short and long sleep duration. In Finland, it was estimated that among the general adult population there are 14.5 % (16.7 % of men and 12.5 % of women) short sleepers (≤6 h) and 13.5 % (10.5 % of men and 16.1 % of women) long sleepers (≥9 h) [196]. However, subpopulations of short and long sleepers are not homogenous. Cross-sectional studies have shown that in any time point in the general population, self-reported short and long sleep are associated with several health conditions (e.g., obesity, diabetes, hypertension, metabolic syndrome, and cardiovascular disease) and different lifestyle factors [181, 188, 192, 196–198]. In a cross-sectional study of 5419 adult men, a higher prevalence of diagnosed myocardial infarction was found among those who slept more than 9 h, while those sleeping less than 6 h per night had a higher occurrence of symptomatic coronary heart disease. This relationship remained after controlling for age, sleep quality, use of sleeping pills and tranquilizers, smoking, alcohol use, Type A score, neuroticism, use of cardiovascular drug, and arterial hypertension [199]. In a large population-based survey with 218,155 Australian adults aged 45 years and over 6 h versus 7 h sleep was associated with statistically significantly increased odds of heart disease (OR 1.11), diabetes (OR 1.159), stroke (OR 1.25), and high blood pressure (OR 1.08) (1.04–1.11). Long sleep (≥9 h) was also significantly related to heart disease (OR 1.14), diabetes (OR 1.25), stroke (OR 1.50), and high blood pressure (OR 1.04) compared to 7 h of sleep [188]. In a meta-analysis of 12 prospective studies both long sleep (RR 1.45; 1.30–1.62) and in a lesser degree short sleep (RR 1.15; 1.07–1.24) were risk factors for stroke [178].
Consequently, probably relative large part of the sleep duration risk groups is at risk because of existing ill health (baseline comorbidities with deviation from the population mean 7–8 h of sleep duration). Therefore, it is important to adjust for comorbidities or individuals with a previous history of a given disease must be excluded from the analysis. Therefore, attention is paid to healthy short and long sleepers. Are they at risk?
In a given time point, healthy adults who sleep less than 6–7 h per night are called “natural short sleepers” and those sleeping more than 9.5 h of sleep “natural long sleepers” [189, 192, 200–203]. This concept makes an assumption that there is an underlying genetic factor which determines whether or not an individual is “a natural short or long sleeper” [189, 204, 205]. However, there are also individuals who have modified their sleep duration due to different external factors [204]. Consistent short sleepers may have a smaller risk than average sleepers (7–8 h) who have become short sleepers or long sleepers later in their life [189]. Genetically, short or long sleepers are at higher risk than midrange sleepers [189, 192, 206].
The fact that an individual is capable, at least for some time, to modify his/her sleep duration and in this way possibly harm one’s own future health has raised a question: Would it be possible that in our modern society sleep duration is gradually shortening among the general population either voluntarily or forced by external factors? It is clear that sleep is not just a biological phenomenon. Its behavioral execution differs in different cultures. For example, the practice of siesta (daytime napping) varies across different cultures [207]. A historian Roger Ekirch has published a fascinating study suggesting that in preindustrial era people in Western Europe experienced, on most evenings, two major intervals of sleep, bridged up to an hour or more of quiet wakefulness [208, 209]. During the past two centuries, the preindustrial segmented sleep pattern has been replaced by a consolidated nocturnal sleep in modern societies. Some studies have found a small long-term decrease in self-reported sleep duration among the general population, but the effect size is clinically meaningless [55, 210]. Recent reviews have concluded that there is no convincing evidence supporting the belief of a secular trend of sleep reduction in general population [211, 212].
Insufficient sleep is the most common cause of daytime fatigue and sleepiness. Insufficient sleep may be due to poor sleep with nocturnal awakenings, too short sleep, or due to some other cause. The lack of sleep may be due to “bad habits”, or a discrepancy between biological and social circadian rhythms, or to some psychic or somatic pathology. In a Japanese study [202] among 3030 adult people, aged 20 or more, 29 % slept less than 6 h per night, and 23 % reported having insufficient sleep. Short sleep duration was the strongest predictor of excessive daytime sleepiness [202]. Similar figures have been published in Europe and USA [202, 213–215]. In Sweden, 12 % of adults had persistent chronic sleep loss, and 50 % of them also reported other concomitant sleeping difficulties. In subjects without sleeping difficulties, the most common cause of insufficient sleep was too little time for sleep [214]. In another study, the prevalence of insufficient sleep, defined as a difference of at least one hour between reported need of sleep and actual sleep length, was 20.4 % (16.2 % in men and 23.9 % in women). Almost half of those with insufficient sleep at baseline had it still 9 years later showing that the problem is often chronic. One-third of the liability to chronic insufficient sleep was attributed to genetic influences [215]. It should be, however, noted that “insufficient sleep” calculated as a difference between self-reported evaluations of one’s need of sleep and self-reported usual sleep duration is not a direct measure of objectively determined sleep deprivation, and caution should be taken when interpreting the results of epidemiological studies on “insufficient sleep.”
Daytime Sleepiness
Some prevalences of daytime sleepiness are shown in Table 28.2. Depending on the study and wording, the occurrence varies from very small 0.3 % to more than 30 % (see Table 28.2). As an average, the prevalence is between 5 and 15 %.
Table 28.2
Occurrence (%) of daytime sleepiness
Study | Size (N) | Population; age | Definition of sleepiness (wordings) | Methods | Percentage (%) |
---|---|---|---|---|---|
Sleeping too much | |||||
Karacan et al. [520] | 1645 | General population; 18–70 years | Too much sleep | Questionnaire | 0.3 |
Bixler et al. [40] | 1006 | General population; 18–80 years | Sleeping too much | Questionnaire | 7.1 (current 4.2) |
Ford and Kamerow [10] | 7954 | Population sample; 18–65+ years | Ever a period of two weeks or longer sleeping too much | Direct structured interview using Diagnostic Interview Schedule | 2.8 (M), 3.5 (W) |
Involuntary sleep attacks, sleeping episodes, irresistible sleep, chance of dozing | |||||
Partinen [227] | 2537M | Army draftees before military service; 17–29 years | Falls asleep at work | Questionnaire | 6.4 |
Partinen and Rimpelä [521] | 2016 | Population sample; 15–64 years | Involuntary sleep attacks daily or almost daily | Phone interview | 3.4 (M), 2.5 (W) |
Billiard et al. [522] | 58,162M | Army draftees; 17–22 years | Daily sleep episodes | Questionnaire | 4.9 |
Klink and Quan [523] | 2187 | Population sample; 18–64 years | Falling asleep during the day | Questionnaire | 12 |
Saarenpää-Heikkilä et al. [524] | 574 | Schoolchildren; 7–17 years | Sleeping during lessons often or always | Questionnaire (both subject and parents) | 3 (B), 0 (G) |
Hays et al. [525] | 3962 | Population sample; 65–85+ years | Most of the time sleepy, forcing to take a nap | Interview | 25.2 |
Ganguli et al. [526] | 1050 | Population sample; 66–97 years | Ever becoming uncontrollably sleepy so that can’t help falling asleep | Interview | 18.9 (no gender difference) |
Johns and Hocking [527] | 331 | Australian workers; 22–59 years | Chance of dozing; ESS > 10 | Questionnaire including the validated ESS | 10.9 (no gender difference) |
Schmitt et al. [528] | 668 | Working population: post office clerks | ESS ≥ 10 | Questionnaire | 13 |
aMelame Melamed and Oksenberg [529] | 532 | Non-shift industrial workers Mean age 46.3 ± 7.6 years | ESS > 10 | Questionnaire | 22.6 |
Kaneita et al. [530] | 28,714 | General Japanese population; 20–70+ years | Do you fall asleep when you must not sleep (for example when you are driving a car)? | Questionnaire | 2.8 (M), 2.2 (W) |
Pallesen et al. [531] | 2301 | Population sample; 18–90 years | ESS > 10 | Telephone interview | 17.7 |
Excessive daytime sleepiness, sleepiness, feeling sleepy during daytime | |||||
Partinen [227] | 2537M | Army draftees; 17–29 years | Do you often feel sleepy during daytime? Are you more sleepy than your friends or workmates? | Questionnaire | 35.8 9.5 |
Partinen and Rimpelä [521] | 2016 | Population sample; 15–64 years | Sleepier than fellow people | Phone interview | 10 (M), 14 (W) |
Lugaresi et al. [532] | 5713 | Population sample; 3–94 years | Sleepiness independent of meal times | Direct interview | 8.7 |
Kelly et al. [533] | 3543 | Students; 13–18 years | Tiredness | Questionnaire | 29.3 |
Martikainen et al. [224] | 1190 | Population sample; 36, 41, 46, 50 years categories | Tiredness/sleepiness (more tired than fellow people; or daily compulsory desire to sleep; or feeling tired every day) | Questionnaire | 6.9 (M), 12.0 (W) |
Saarenpää-Heikkilä et al. [524] | 574 | Schoolchildren; 7–17 | Daytime sleepiness always or often | Questionnaire (both subjects and parents) | 20 (B), 22 (G) |
Janson et al. [147] | 2394 | Population sample; 20–44 years | Feeling drowsy in the daytime 3 per week | Questionnaire | 16.0 |
Hublin et al. [201] | 11,354 | Population sample; 33–60 years | Daytime sleepiness daily or almost daily | Questionnaire | 6.7 (M), 11.0 (W) |
Ohayon et al. [534] | 4952 | Population sample; 15–100 years | Feeling sleepy during the day on a daily basis at least 1 month a lot or greatly (severe sleepiness) or moderately (moderate sleepiness) | Telephone interview using the Sleep-Eval system | Severe: 4.4 (M), 6.6 (W) Moderate 15.2 |
Ohida et al. [535] | 106,297 | Japanese adolescents; 12–19 years Junior and Senior High Schools | Do you feel excessively sleepy during the daytime? | Questionnaire | 33.3 (B), 39.2 (G) |
Sleepiness may be interpreted differently in different languages. The English word “sleepiness” is defined in the Random House Unabridged Dictionary and the Scribner-Bantam dictionary as “inclined to sleep, drowsy,” and in Stedman’s Medical Dictionary as “an inclination to sleep.” Synonyms for “sleepy” include words such as tired and somnolent. Fatigue is a more general term describing, according to Stedman’s, a state of lessened capacity for work and reduced efficiency of accomplishment, usually accompanied by a feeling of weariness, sleepiness, or irritability [216]. A more original description of fatigue is found in the highly appreciated French Dictionnaire des Termes Techniques de Médecine by Garnier and Delamare published in 1969, where fatigue is defined as “a state resulting from prolonged activity of an organ or system. It is translated as decrease of function and a particular sensation (feeling of fatigue) which is specific to each organ. The aim of training is to retard apparition of fatigue.” Tiredness comes from the word tired, which is synonymous to weary, wearied, exhausted, fatigued, jaded, and bushed. In the Random House Unabridged Dictionary, “tired” suggests a condition in which a large part of one’s energy and vitality has been consumed. One who is “tired” has used up a considerable part of his or her bodily or mental resources. One who is “fatigued” has consumed energy to a point where rest and sleep are demanded. Simple rest may decrease tiredness and feelings of fatigue but sleep is needed to relieve sleepiness. In sum, sleepiness is a preferred term to describe inclination to sleep or tendency to fall asleep.
Sleep-related breathing disorder is the second most common reason of excessive daytime sleepiness among adults after lack of sleep [201, 202]. Also, the use of hypnotic agents, other sleeping difficulties, and irregular sleep–wake schedule are related to daytime sleepiness. In most sleep laboratory populations, more than 75 % of patients with daytime sleepiness have sleep-related breathing disorders (most commonly obstructive sleep apnea syndrome), 20 % have narcolepsy, and 5 % have restless legs, periodic movements in sleep, or other sleep disorders. This does not reflect distribution in general population, where insufficient and/or poor quality of sleep explains most cases of sleepiness.
Narcolepsy
The prevalence of narcolepsy varies in population-based studies between 21 and 56 per 100,000 persons [217–235]. In some studies, sampling has been, for example, by newspaper advertisements and TV programs and these studies do not represent well-defined population samples. In addition, there are many unknown assumptions in some of the early studies and therefore these are not taken into consideration [226, 236–238]. The highest figures, around 160 or up to 590 per 100,000, are from Japan [219, 220, 230]. The lowest frequency, 0.23 per 100,000 population, is found in Israel [231, 239].
A simple screening method called the Ullanlinna Narcolepsy Scale (UNS) has been developed and validated [222]. The UNS consists of 11 items assessing cataplexy-like symptoms and tendency to fall asleep. The score varies from 0 to 44 and the cut point for diagnosing narcolepsy is 14. Using the UNS, the prevalence of narcolepsy with cataplexy was 26 per 100,000 population in adult Finns [223]. Very similar figures have been published from Hong Kong. Using the validated Chinese version of UNS, the prevalence rate of narcolepsy among southern Chinese was 34 per 100,000 (95 % CI 10–117). In Hong Kong, all narcoleptic subjects were HLA DRB1-1501 positive and 50 % were DQB1*06:02 positive [233]. Using the same methodology, the prevalence among Korean students is around 15 per 100,000 [240].
Cataplexy is one of the core symptoms of narcolepsy, but occasional cataplexy-like attacks may occur also in healthy subjects. Among young men, 16.5 % experience at least sometimes sudden weakness in some muscle groups that are associated with emotions. 3.7 % of young men had experienced such cataplexy-like symptoms often or almost during the past month [227]. In another study, 29.3 % of the people reported (at least once during his or her lifetime) feelings of limb weakness associated with emotions [223]. If this is considered as evidence of cataplexy and combined with the occurrence of daytime sleep episodes at least 3 days per week, 6.5 % of the population would have narcolepsy according to the minimal diagnostic criteria for narcolepsy of the ICSD. Clinical, polysomnographic, and CSF examinations of hypocretin allow more exact diagnosis and more precise occurrence rates [241–246].
In the current ICSD-3, narcolepsy is divided into narcolepsy type 1 (narcolepsy with certain cataplexy; narcolepsy with low CSF hypocretin; NT1) and narcolepsy type 2 (no cataplexy, CSF hypocretin normal; NT2). NT1 is probably an autoimmune/autoinflammatory disease. NT2 is more heterogenous and iNT2, and idiopathic hypersomnia are very difficult to distinguish from each other. Using the new diagnostic classification, NT2, as well as idiopathic hypersomnia, seem to be much less common than NT1, but population-based epidemiological studies do not yet exist. Also, the existing prevalence studies, as shown in Table 28.3, must be interpreted with caution, as the criteria for diagnosis of narcolepsy have been variable. For example, in the Longstreth study only 50 % of the diagnosed narcolepsy with cataplexy were HLA DQB1*0602 positive [234], contrasting to almost 100 % positivity among NT1 patients in some recent studies [247–249]. Also, normal people may report of occasional cataplectic attacks and therefore it is uncertain if all subjects in that study, in fact, had true narcolepsy.
Table 28.3
Prevalence of narcolepsy. Studies on representative population samples
Study | Number (age range) country | Population, methods and comments | Prevalence per 100,000 (CI) |
---|---|---|---|
Solomon [229] | 10,000 (16–34) USA | Black men. No precise information on cataplexy | 20 (0–4.8) |
Solomon [229] | 189,196 USA | Male population sample. No precise diagnosis | 3 (0.6–5.7) |
12,469 (12–16) Japan | Population sample, Que, personal interview | 160 (9–230) | |
Partinen [227] | 2537 (18–29) Finland | Young male population, recruits, Que, PSG | 79 (6–287) |
Franceschi et al. [218] | 2518 (6–92) Italy | Hospital patients, Que, PSG | 40 (0–118) |
Wilner et al. [231] | 1800 (30–57) Israel (Jews) | Hospital patient sample, PSG, HLA typing | 0.23 (N/A) |
Martikainen et al. [224] | 1190 (36–50) Finland | Population sample, Que | 168 (18–604) |
Tashiro et al. [230] | 4559 (17–59) Japan | Population sample, Que | 590 (369–816) |
Al Rajeh et al. [217] | 23,227 (all ages) Saudi Arabia | Population sample, personal interview, clinical examination | 4 (0–13) |
Wing et al. [232] | 342 (N/A), Chinese, Hong Kong | Patient population, PSG, HLA typing | 1–40 (N/A) |
Hublin et al. [223] | 11,354 (33–60) Finland | Population sample, Que, UNS, Phone interv., clin. exam., PSG, HLA typing | 26 (0–56) with cataplexy |
Ohayon et al. [225] | 4972 (15–100) United Kingdom | Population sample, telephone survey | 40 (0–96) |
Ondzé et al. [235] | 14,195 (15+) France | Patients consulting physicians, Que | 21 (4–62) |
Silber et al. [228] | Census data of Minnesota, USA | Record linkage of diagnosed patients; Incidence 1.4 (0.95–1.9)/100,000 persons/year | 56 (42–73) 36 (25–50) with cataplexy |
Wing et al. [233] | 9851 Chinese Hong Kong | Population sample, phone interview, UNS, clin. exam., PSG, HLA typing | 34 (10–117) with cataplexy |
Ohayon et al. [226] | 18,980, UK, Germany, Italy, Portugal, Spain | Population samples, telephone surveys | 47 (N/A) |
Shin et al. [240] | 20,407 (14–19) Korea | Student population, UNS, interview, PSG, HLA typing | 15 (0–31) |
Longstreth et al. [234] | About 1.4 million (18+), USA, King County | Multiple overlapping methods, newspaper advertisements, newsletters, patients groups etc., interviews, HLA typing | 30.6 (27.6–33.5) 21.8 (18.8–28.8) with cataplexy 15.3 (12.8–17.9) with DQB1*0602 |
Incidence of narcolepsy. Studies on incidence of narcolepsy are scarce, but it seems that the incidence of narcolepsy is approximately 1 per 100,000 people per year [228, 248, 250, 251]. In Minnesota, the incidence of definite and probable narcolepsy with or without cataplexy was 1.37 per 100,000 persons per year (1.72 for men and 1.05 for women) [228]. In the same study, incidence of narcolepsy with cataplexy was 0.74 per 100,000 persons per year being highest in 10–19-year-olds (1.8/100,000/year) [228]. Computed from their data, the incidences of narcolepsy with cataplexy were 1.0 per 100,000 persons per year in people aged less than 40 years and 0.1/100,000/year in people aged 40 years or more.
After the adjuvanted H1N1-vaccinations, the incidence of narcolepsy increased significantly, especially in those European countries, where Pandemrix® vaccination was the only vaccination used [247, 250, 252–259]. In Finland, all patients with Pandemrix-associated narcolepsy were HLA DQB1*06:02 positive and all patients who have had a lumbar puncture have been type 1 with low CSF hypocretin levels [247, 248]. After Pandemrix vaccination in Finland, the average incidence of narcolepsy increased in people aged under 17 years from 0.3 to 5.3 per 100,000 people per year [247]. In a retrospective cohort study, the incidence of narcolepsy was 9.0 in the vaccinated as compared to 0.7/100,000 person-years in the unvaccinated individuals, the rate ratio being 12.7 (95 % confidence interval 6.1–30.8) [252]. Reports from other countries have also shown a statistically significantly increased risk of developing narcolepsy after Pandemrix® vaccination [250, 253–259]. The incidence rate in children before vaccination campaign was lower, e.g., in Finland than in Minnesota [228], and it is possible that some children had remained undiagnosed before the vaccination campaign. The incidence rates in children, adolescents, and also in adults rose anyway to a much higher level than ever before indicating a relationship with the vaccination. Recent immunological studies provide a link between Pandemrix and narcolepsy. The results have moved the focus from adjuvants onto the H1N1 viral proteins [248, 249, 260].
There are some interesting associations with time of birth and narcolepsy. In southern China, an excess of winter births has been found in subjects with narcolepsy-cataplexy [261]. In a large multicenter (Montpellier, Montreal, and Stanford) study, birth dates of 886 patients with a clear-cut diagnosis of narcolepsy with cataplexy were compared with birth dates of the comparative general populations. The birth rate of narcoleptic patients was highest in March (OR 1.45) and lowest in September (OR 0.63 compared to general population). No gender or country of origin differences were observed [262].
Snoring and Sleep Apnea
Habitual Snoring
Snoring is an inspiratory noise (occasionally also occurs during expiration) caused by vibration of the soft upper airways tissues, mainly soft palate and posterior faucial pillars. Snoring corresponds to partial obstruction of the upper airways, and complete obstruction is followed by an apnea. Almost everybody snores sometimes especially when sleeping in a supine position. It is well known that alcohol increases snoring. Habitual (almost every night or every night) snoring is practically always present in patients with obstructive sleep apnea syndrome (OSAS). Children and elderly people may not snore loudly. Instead, they are breathing with their mouth open. In the first large-scale epidemiological study on snoring, about 24 % of San Marino men and 14 % of San Marino women were reported to habitually snore [263]. In Finland, 9 % of adult men and 3.6 % of adult women reported snoring always or almost always when asleep [264]. A recent Finnish study found that when individuals with prior history of cardiovascular disease were excluded from the Health 2000 sample of adult (≥30 years of age) population among the remaining 5177 individuals 17.8 % (11.9 % of women and 25.2 % of men) reported to snore habitually [265]. Among Hispanic–American adults, the age-adjusted prevalence rate of regular loud snoring was 27.8 % in men and 15.3 % in women [266]. Snoring increases with age up to 60–65 years and decreases in older age [264, 266–273]. In many studies, prevalence of snoring has been higher but in such cases history of snoring has often been asked with only two or three possible response alternatives. Because almost everybody snores sometimes snoring figures are high. Occasional snoring or snoring as a dichotomous variable is not related to significant pathology [265, 274, 275]. On the contrary, habitual almost every night snoring seems to be a risk factor for cardiovascular and cerebrovascular diseases [265, 274, 276]. For a clinician, this is important. Having a positive response to snoring is not sufficient. One needs to know how often someone is snoring. When asking for frequency, semi-quantitative scales with defined time frames should be used. For example, “often” can mean once a month for someone but almost every day for another. Snoring history is significant if someone snores on at least 5 nights per week; in other words, someone is a habitual snorer. One can ask further also about presence of sleep apnea (breathing cessations) and snoring stertorousness.
If someone snores almost every night very loudly and intermittently and someone (a cohabiting person) has noticed breathing pauses on about 3–5 nights per week, the probability of obstructive sleep apnea is high. If the subject has been snoring for more than 13 years, it is almost certain that he/she has sleep apnea [277]. The probability of sleep apnea increases further if the clinical examination is suggestive of sleep apnea. Some of the best-known strong indicators of possible sleep apnea include thick neck, large waist circumference (visceral fat), increased fat under the chin, pathological Mallampati score with large tongue and/or large tonsils, and narrow upper airways with high ogival hard palate. If the snoring and apnea history are positive and the clinical examination is suggestive of sleep apnea, the prior probability can be higher than 90 %. In other words, the diagnostic certainty is better than that of a screening sleep recording. It is also important to recognize false positive responses from screening recordings. Unfortunately, a good clinical history and clinical examination are sometimes overlooked trusting too much on the results of sleep recordings.
The main underlying mechanism behind the associations of sleep-disordered breathing (habitual snoring) and cardiovascular outcomes is obstructive sleep apnea (OSA). However, heavy habitual (every night) snoring (i.e., partial upper airway obstruction) may influence pulmonary arterial pressure even without apneas, and expose the heart and circulation to a cascade of detrimental stimuli such as increased intrathoracic pressure, autonomic dysregulation, platelet activation, oxidative stress, and endothelial dysfunction resulting in the development of cardiovascular disorders [278], daytime sleepiness, insulin resistance, metabolic syndrome, and other health consequences [277–295].
Sleep Apnea
Sleep-related breathing disorders (SRBD) and sleep-disordered breathing (SDB) refer mostly to sleep apnea. In a large population-based study, more than 2000 adults, aged 40–85 years, had a polygraphic sleep recording [296]. The median apnea-hypopnea index (AHI) was 6.9/h in women and 14.9/h in men. The prevalence of moderate to severe sleep-disordered breathing (defined according to AASM as AHI ≥ 15) was 23.4 % (20.9–26.0) in women and 49.7 % (46.6–52.8) in men [296]. Can these results be interpreted as meaning that almost 50 % of over 40-year-old men have SRBD, which should be treated by nasal CPAP as current medical textbooks are suggesting? Many questions remain open. Sleep apnea is very common and it is associated with many health-related outcomes. More prospective studies as well as randomized studies are needed anyway. Using the current “pathological limit” of AHI (AHI ≥ 5), even more than 80 % of men and 60 % of women could be defined as “patients with sleep apnea,” if we use the recent Swiss figures [296]. The current definitions of sleep apnea using AHI indices must be revised, as the “original” historical limits of AHI (mild ≥5, moderate 15–30, severe ≥30) have been “taken from the pocket” and they have not originated from physiological background.
Currently, the generally accepted prevalence of symptomatic and clinically significant OSAS (SRBD with excessive daytime sleepiness) among adult men is probably somewhere between 3 and 7 %, and in women the prevalence of OSAS varies from 2 to 5 %. There is an age relationship. The prevalence of OSAS among men aged 40–59 may be greater than 4 % or even much greater than 10 %. Although sleep apneas are frequently found among elderly subjects, the occurrence of clinically significant sleep apnea is less common in older age groups than among people aged 40–65. In young women, OSAS is rare, but after menopause, up to the age of 65, it is almost as common as among men. Sleep apnea is not rare among children. Apneas with AHI > 5 may be found in more than 14 % but symptomatic sleep apnea syndrome is rare [267, 269, 297–304]. In the first population-based studies, the prevalence of OSAS varied between 1 and 4 %, but recent estimation have been higher. Men have higher figures than premenopausal women. Among adults, children, and also among subjects with mental retardation, OSAS is highly associated with central obesity [13, 14, 269, 270, 272, 273, 279, 298, 299, 304–321].
Obstructive sleep apneas are part of the complex of “heavy snorer’s disease” as defined by Lugaresi et al [322]. As stated above, heavy snoring is as such, without sleep apnea syndrome, a risk factor for many health outcomes.
Sleep apnea should be properly quantified, not only by an apnea index (AI) (or apnea-hypopnea index; AHI) but also by the number of oxygen desaturations, limitation of air flow, degree of daytime sleepiness, and by cardiovascular function. An AI of 5 or AHI of 10 is commonly used as a criterion of OSAS. The diagnostic criteria should be adjusted for age [315–317, 323–325]. It may be that while the occurrence of sleep apnea increases with age the clinical meaningfulness of apnea decreases among elderly people. Hence, occurrence of clinically significant sleep apnea syndrome is rare among elderly people, who often have many other diseases that may be indirectly associated with sleep apnea [326–330].
Risk Factors for Snoring and Sleep Apnea
Central Obesity, Thick Neck, and Obstructed Upper Airways
Central visceral obesity is the most important risk factor for snoring and sleep apnea. Obesity is commonly measured by body mass index (BMI), which is calculated as weight in kilograms divided by square of height in meters [331]. According to the WHO criteria, adults with a BMI of 25 kg/m2 and over may be considered as overweight and those with BMI of 30 kg/m2 and over are considered as obese. According to WHO, in 2006, more than 60 % of US and UK citizens were overweight and almost 30 % of US citizens were obese with BMI over 30 kgm−2. In the Nauru Islands almost 80 % and in the French Polynesia 36 % were obese (see, e.g., WHO Web pages on statistics of BMI/obesity [332]).
The frequency of snoring and sleep apnea increases with obesity in all published epidemiological reports. The same is true for heavy snoring and sleep apnea. For example, in the Katz’s study habitual snoring was found to occur in 7 % of men and 2.8 % of women with a BMI of less than 27 kg/m2 and in 13.9 % and 6.1 %, respectively, of those above this level [203].
Using multivariable analysis, the Oxford group of John Stradling first reported that neck size is more closely related to severity of sleep apnea than BMI [303, 333–335]. Neck size may be easily measured, and it is a useful indicator of upper body obesity. Several lines of evidence show that central obesity (large waist circumference) is related to increased risk of cardiovascular disease, diabetes, and metabolic syndrome [336–342]. Other clinical markers that are risk factors for sleep apnea include high Mallampati score and small cricomental space [335, 341, 343–348]. If the cricomental space is more than 1.5 cm, obstructive sleep apnea is unlikely; in the study of Tsai et al., its negative predictive value was 100 % (95 % CI 75–100 %) [348].
Anatomically narrow upper airways. Lean people may have abnormal upper airways. Anything that obstructs upper airways is a risk factor for heavy snoring and sleep apnea. Among known risk factors are large adenoids or tonsils, and rhinitis [349–353]. Other abnormalities in upper airways are, e.g., those found in different dysmorphic syndromes, in mentally disabled people, in acromegaly, and familial amyloidosis [354–362].
In sum, obesity is linked with probability of snoring and sleep apnea, but BMI is not the best indicator of obesity. Therefore, at least, waist circumference, neck circumference, and cricomental space should be measured in all future clinical and epidemiological studies on snoring and sleep apnea. Waist- and neck circumference as well as the cricomental space may be estimated relatively well also in surveys that are based on questionnaires using different pictures of the head and neck. Responders can then choose the picture that resembles most their own faces. The collar size of a shirt correlates well with actual neck circumference.
Other Risk Factors for Snoring and Sleep Apnea
Among 2187 subjects representative of a general adult population in Tucson, major independent risk factors for snoring were male gender, age between 40 and 64 years, obesity, and cigarette smoking [363]. Snoring was more common in subjects who regularly used alcohol or hypnotics. The effect of smoking may be related to upper airway inflammation and edema caused by cigarette smoke.
Alcohol increases upper airway resistance and tends to induce obstructive sleep apnea in healthy people and especially among chronic snorers [349–353, 364–368]. This is probably due to the acute centrally depressing effects of alcohol. Among other risk factors, hostility is associated with habitual snoring [369].
Ethnic Differences in Occurrence of Snoring and Sleep Apnea
Prevalence and incidence of sleep apnea vary according to ethnicity (Table 28.4). In a study in Singapore comparing prevalence of snoring among Chinese, Malay, and Indian, the average prevalence of snoring was 6.8 % (53–83 %). The ethnic differences were significant. Among Chinese, 6.2 % (4.4–8.1), among Malay 8.1 % (6.1–10.2 %), and among Indians 10.9 % (85–13.4 %) snored. The minimum whole population prevalence by the most restricted symptom criteria for defining sleep breathing-related disorder was 0.43 % (0.05–0.8 %) [308]. In Singapore, some estimations have given a prevalence of 15 % for sleep apnea [370]. This figure is based, however, only on a small sample of people and it may not be representative of the whole Singapore population. Therefore, the figure is probably too high. The reasons may be related to differences in craniofacial anatomy and also to environmental effects. Examples of the latter include obesity which seems to be significantly more common, e.g., among African Americans and also among Saudi Arabian women than in people of Caucasian origin [308, 316, 371–374]. Reasons for higher occurrence of obesity are multiple, and socioeconomical factors should not be forgotten. Unemployment, alcoholism, and lack of financial security are known to be associated with higher figures of obesity. It must be emphasized that there is still a considerable lack of epidemiological data regarding the prevalence of OSA in Asian communities and consequently all inferences about the differences across Asian and European ethnicity should be made with caution [375].
Table 28.4
Occurrence of obstructive sleep apnea and sleep apnea syndrome
References, country | Population subjects | Age (years) | Criteria; comments | Prevalence (%) |
---|---|---|---|---|
Lavie [302], Israel | 1262 men | 18–67 | AI > 10, symptomatic | 1.0–5.9 |
Telakivi et al. [304], Finland | 1939 men | 30–69 | Habitual snoring, EDS, RDI > 10 | 0.4–1.4 |
Gislason et al. [269], Sweden | 3201 men | 30–69 | Habitual snoring, EDS, AHI > 10 | 0.7–1.9 |
Cirignotta et al. [267], Italy | 1170 men | 30–39 40–59 60–69 | AI > 10, symptomatic AI > 10, symptomatic AI > 10, symptomatic | 0.2–1.0 3.4–5.0 0.5–1.1 |
Stradling and Crosby [303], Great Britain | 893 men | 35–65 | ODI4 > 20, symptomatic ODI4 > 10 ODI4 > 5 | 0.3 1.0 4.6 |
Haraldsson et al. [301], Sweden | 846 men | 30–69 | Positive history of OSA, EDS, and PSG | 2.8–5.5 |
Young et al. [13], USA | 352 men 250 women | 30–60 30–60 | Hypersomnia and RDI ≥ 5 | 4.0 (M) 2.0 (W) |
Gislason et al. [306], Iceland | 2016 women | 40–59 | Habitual snoring, EDS, PSG | >2.5 |
Olson et al. [273], Australia | 1233 men 969 women | 35–69 | AHI ≥ 15 AHI ≥ 10 AHI ≥ 5 | 4–18 7–35 14–69 |
Bearpark et al. [300], Australia | 294 men | 40–65 | RDI ≥ 10 EDS and RDI ≥ 5 | 10.0 ≥3.0 |
Gislason et al. [306], Iceland | 555 children | 0.5–6 | Habitual snoring or apneas, ODI4 > 3 | >2.9 |
Ohayon et al. [318], Great Britain | 2078 men 2894 women | 15–100 15–100 | N/A, telephone survey; no PSG N/A, telephone survey; no PSG | 2.4–4.6 0.8–2.2 |
Kripke et al. [297], USA | 165 men 190 women | 40–64 40–64 | ODI4 > 20 ODI4 > 20 | 5.4–13.2 2.1–8.3 |
Marin et al. [298], Spain | 1360, men and women; quota | >18 | Habitual snoring, apneas, EDS, clinical examination, oximetry | Men: ≥2.2 Women: ≥0.8 |
Bixler et al. [315], USA | 4364 men 741 in lab | 20–100 | AHI ≥ 10 and daytime symptoms with EDS | 3.3 45–64 years: 4.7 |
Ng et al. [308], Singapore | 2298 | 20–74 | Questionnaire with strict criteria; no PSG | 0.43 (0.05–0.8) |
Puvanendran and Goh [370], Singapore | 220 interviews, 106 in lab | 30–60 | Habitual loud snoring, EDS, AI > 5 | 15 |
Zamarron et al. [299], Spain | 76; random sample | 50–70 | Medical history, examination, AHI ≥ 5 | AHI ≥ 5: 28.9 % OSAS: 6.8 % |
Bixler et al. [317], USA | 12,219 women 1000 in lab | 20–100 | AHI ≥ 10 and daytime symptoms with EDS | 1.2 Pre: 0.6 Post + HRT: 0.5 Post: 2.7 |
Özdemir et al. [312], Turkey | 2638 men 2701 women | 20–107 | Questionnaire. History of stopping to breath when sleeping; no PSG | 6.4 |
Heinzer et al. [296], Switzerland | 1024 men 1097 women | 40–85 | Polysomnographic recordings | Men: AHI ≥ 5: 83.8 % AHI ≥ 15: 49.7 % Women: AHI ≥ 5: 60.8 % AHI ≥ 15: 23.4 % |
Snoring and Sleep Apnea in Children
Snoring or obstructive sleep apnea is common in children of all ages. It must be noted that among children snoring may not be loud. A manifestation of obstructed upper airways may be that the child always sleeps with open mouth. Among 1615 Italian children, aged 6–13, 118 children (7.3 %) were habitual snorers. Children with rhinitis were more than twice as likely to be habitual snorers than others. There was a positive correlation between parental smoking and the presence of snoring in children [349]. In Iceland, 3.2 % of 555 children aged 6 months to 6 years snored often or always [270]. The estimated minimal prevalence of obstructive sleep apnea in that age group was 3.2 %. In one study among 4–5–year-old children, significant sleep and breathing disorders occurred in 0.7 % [376]. In a Spanish study, the prevalence of sleep-disordered breathing among adolescents aged 12–16 was 1.9 % [377]. Adenotonsillar hypertrophy is the most common cause of upper airway obstruction in infants and children [352, 378, 379].
Sleep Apnea Among Elderly People
Habitual snoring seems to decrease after age of 65 or 70 years [380]. In a random sample of 5201 Medicare enrollees, 65-year-old or older, 33 % of the men and 19 % of the women reported loud snoring. Snoring was less frequent in people that were aged over 75. Observed apneas were reported by 13 % of men and 4 % of women [381]. In California, 19–24 % of people older than 65 years have an AI > 5, and 62 % of elderly people have a respiratory disturbance index (RDI) ≥10 [324, 325, 380]. The clinical significance of the high frequency of sleep apnea among elderly people remains to be seen. Presence of sleep apnea in an elderly subject does not mean that he/she has OSAS, and it does not mean that all elderly subjects with AHI or RDI over 10 should receive CPAP. In a cohort of 426 elderly people those with a RDI ≥30 had significantly shorter survival but the RDI was not an independent predictor of death among the elderly subjects during five years of follow-up when age, cardiovascular disease, and pulmonary disease were used as covariates [326]. The frequent occurrence of sleep apnea means, on the contrary, that we should perhaps use higher cut-point values of AI and AHI for elderly people [315].
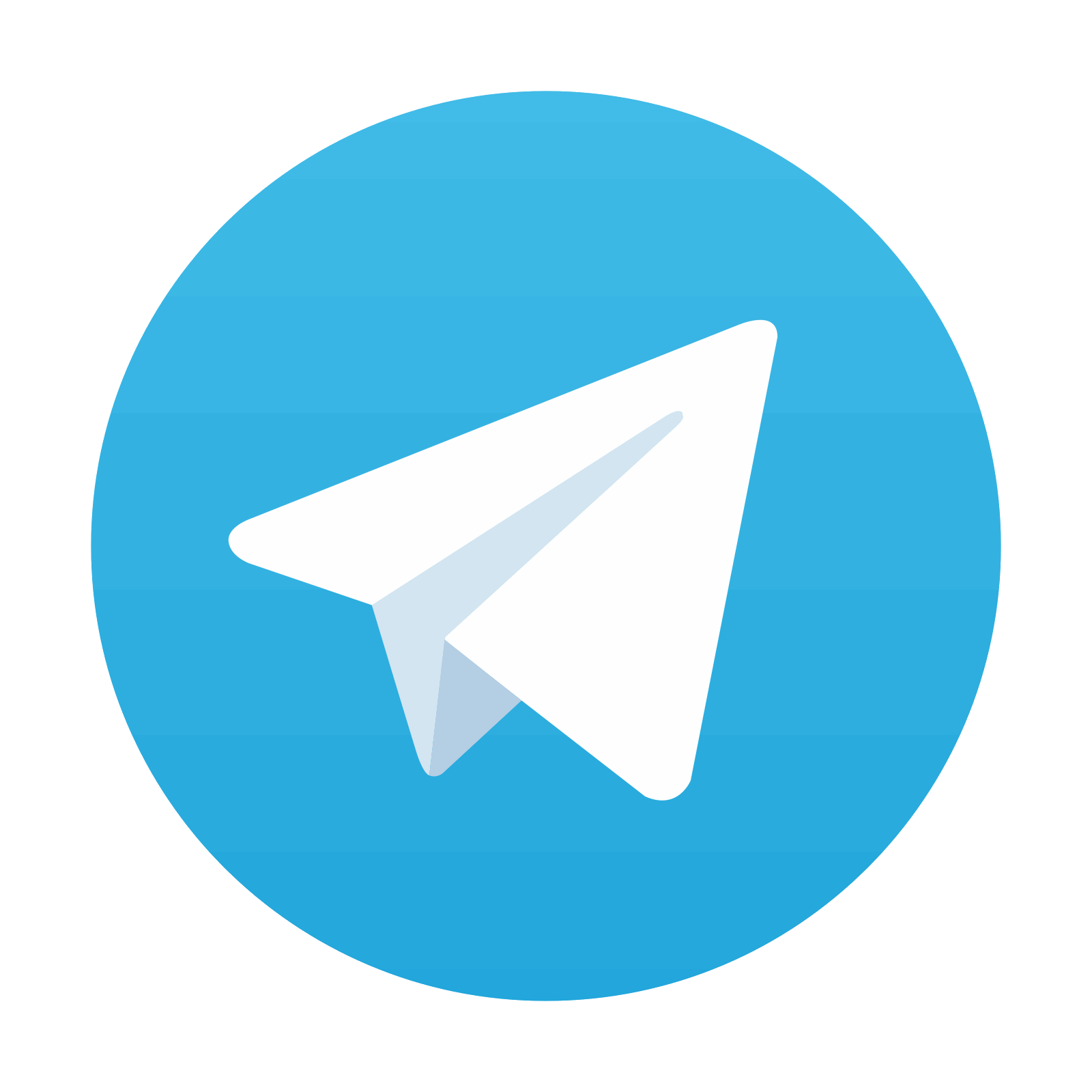
Stay updated, free articles. Join our Telegram channel

Full access? Get Clinical Tree
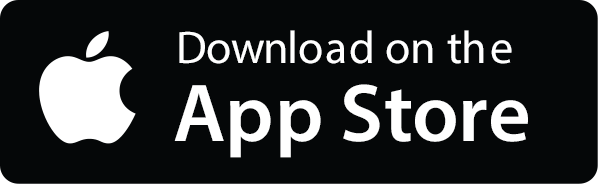
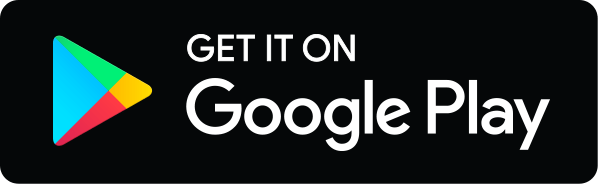