Seizures in the neonatal period are a frequent and ominous sign of a multitude of conditions. Quick and effective treatment is important to prevent late sequelae in cognitive development; treatment efficacy however depends on the underlying cause. It is also hampered by the sometimes-difficult recognition of seizures in the setting of a neonatal intensive care unit in often critically ill children, as neonatal seizures might be very subtle in their manifestation. Simultaneous video EEG studies are at times necessary to correctly identify all seizure events. They may present as focal clonic seizures, oral automatisms, grimacing, complex movements like pedaling, myoclonic seizures or tonic seizures, and rarely as apnea. The most important cause of neonatal seizures is birth asphyxia. Inborn errors of metabolism as a group are also important, and prompt recognition of the few treatable disorders is crucial for preserving the potential of normal development. In this chapter, we would like to present the inborn errors of metabolism presenting with neonatal seizures (see also Tables 15–1 and 15–2) and focus on treatable disorders, offering algorithms for treatment and the diagnostic approach.
Disorder | OMIM Number | Diagnostic Test |
---|---|---|
Pyridoxine dependency | 266100 | Response to pyridoxine; elevated pipecolic acid (CSF, plasma) and α-aminoadipic semialdehyde (urine) |
PNPO deficiency | 610090 | Response to pyridoxal 5-phosphate; elevated glycine, threonine, orthomethyldopa |
Folinic acid dependency | Response to folinic acid; unknown peak in CSF HPLC | |
Phosphoserine aminotransferase deficiency | 610936 | Low CSF and plasma serine and glycine |
Nonketotic hyperglycinemia | 605899 | Elevated CSF: plasma glycine ratio |
Urea cycle defects | Hyperammonemia, plasma amino acids, urine orotic acid | |
Organic acidurias | Urinary organic acids, acylcarnitines | |
Maple syrup urine disease | 248600 | Plasma amino acids: elevation of leucine |
Zellweger syndrome | 214100 | Elevated VLCFA |
Neonatal adrenoleukodystrophy | 202370 | Elevated VLCFA |
Holocarboxylase synthetase deficiency | 253270 | Organic acids |
Molybdenum cofactor disease | 252150 | Sulphite test in fresh urine, fibroblast studies |
Sulphite oxidase deficiency | 272300 | Sulphite test in fresh urine, fibroblast studies |
Adenylosuccinate lyase deficiency | 103050 | Modified Bratton–Marshall test in urine |
Respiratory chain disorders and PDHc deficiency | Lactate elevation, activity of respiratory chain enzymes and PDHc in muscle | |
Glutamate transporter deficiency | 609302 | Glutamate oxidation in fibroblasts, sequencing of SLC25A22 |
Congenital glutamine deficiency | 610015 | Extremely low glutamine in plasma, urine, and CSF |
Neonatal form of neuronal ceroid lipofuscinosis | 610127 | Cathepsin D activity |
Disorder | OMIM Number | Diagnostic Test |
---|---|---|
GLUT1 deficiency syndrome | 606777 | Decreased CSF: serum glucose ratio |
Serine biosynthesis defects | 601815, 172480 | Low serine in CSF amino acid analysis |
Biotinidase deficiency | 253260 | Decreased biotinidase activity |
Creatine synthesis defects (especially GAMT deficiency) | 601240, 602360, 300352 | Absent creatine peak in cerebral 1H-MR spectroscopy; elevated guanidinoacetate; elevated creatine:creatinine ratio |
Organic acidurias | Urinary organic acids, acylcarnitines | |
Aminoacidopathies | Plasma amino acids | |
Ganglioside synthesis deficiency | 609056 | Ganglioside analysis in plasma |
Storage disorders | Enzyme analysis, demonstration of storage material | |
Menke’s disease | 309400 | Serum copper and ceruloplasmin |
First-line diagnostic tests have to rule out easily recognizable and treatable conditions such as hypoglycemia, hypocalcemia or hypomagnesemia. Ammonia concentrations should also be measured, because neonatal seizures may be the first symptoms before hyperammonemic coma develops. Treatment with pyridoxine and pyridoxal phosphate is diagnostic as well as possibly therapeutic—if a neonate responds to pyridoxine, one can concentrate on proving this inborn error instead of running all possible tests. Full resuscitation equipment should be present when cofactors are given. Apnea in pyridoxine phosphate responsive seizures is also possible with oral administration of this vitamin.
The diagnostic approach should be stepwise (Fig. 15–1). First, common and treatable conditions should be sought for; the rare disorders such as GABA transaminase deficiency or the defect in glutamate transport are not among the disorders screened in first-line testing. If there is no response to cofactor treatment, lumbar puncture should be performed including analysis of glucose, cell count, protein, lactate, and amino acids. If possible, 1 or 2 mL of cerebrospinal fluid (CSF) should be stored for further investigations. Analysis of amine neurotransmitter metabolites may be helpful in pyridoxal phosphate-dependent seizures (pyridox(am)ine 5′-phosphate oxidase [PNPO] deficiency), but may also be normal in this condition.12 Fibroblasts and DNA should be stored if there is no definitive diagnosis.
Cranial ultrasound should be performed promptly in all neonates with seizures. Hemorrhage or edema may be found, some malformations as agenesis of the corpus callosum or lissencephaly may be visible. Cranial MRI, if possible with 1H-MR spectroscopy, should be done if seizures persist after treatment with cofactors and the child is sufficiently stable. It allows diagnosis of malformations, neonatal stroke, or asphyxia. In many of the inborn errors of metabolism, initial MR imaging is normal or shows nonspecific abnormalities, which may even imitate those of birth asphyxia.
Cofactors should be given as first-line treatment, even in neonates in whom asphyxia is strongly suspected. Figure 15–2 proposes a practical approach. Any underlying treatable metabolic disorder must be treated appropriately as soon as diagnosis has been made, if necessary, in a specialized centre. If cofactor treatment fails, seizures have to be treated with conventional antiepileptic drugs. Phenobarbital is still the medication of choice although there is concern about increasing nerve cell apoptosis. Phenytoin may be used if phenobarbital does not control seizures. The role of the newer antiepileptic drugs is not yet clear. If, as often is the case, initial treatment was with conventional antiepileptic drugs, cofactor treatment also has to be started. Each institution should have a protocol for treatment and diagnostic approach of neonates with seizures.
Most neonatal seizures are not due to metabolic disorders, but to birth asphyxia or neonatal stroke. Many neonatologists are therefore reluctant to run elaborate diagnostic tests including a spinal tap and trying vitamins to treat seizures. EEG or MRI are not always helpful in the differential diagnoses. In birth asphyxia, neonates typically recover from the initial crisis and can be extubated and weaned off antiepileptic medication. In contrast inborn errors of metabolism, do not improve without specific treatment, and often deteriorate over time. Still, a few metabolic disorders can imitate neonatal asphyxia and can develop into a residual state, which is easily mistaken for the sequelae of birth asphyxia. A pragmatic approach could be always to treat with cofactors, but to reserve further metabolic investigations for those infants without clear evidence of hypoxic–ischemic encephalopathy.
In many cases, a final diagnosis cannot be made. Here, one should always store skin fibroblasts of the patient and DNA, if possible, from all family members, in order to investigate for “new” metabolic or genetic disorders in the future.
Hypoglycemia is a frequent cause for neonatal seizures, and blood glucose should be immediately measured in every infant with seizures.
In recurrent hypoglycemia, the underlying problem has to be actively sought for and appropriately treated. Prolonged untreated hypoglycemia is deleterious and leads to lasting injury of the parietal and occipital cortex and its underlying white matter.1 Treatment with glucose is straightforward and stops the seizures (see below for more detail).
Although pyridoxine dependent epilepsy (OMIM 266100) was first described in 1954;2 the molecular basis was discovered only recently.3 Mutations in the ALDH7A1 gene catalyzing a step in the lysine degradation pathway lead to elevation of Δ1-piperideine-6-carboxylate (Fig. 15–3), which inactivates pyridoxal phosphate by a condensation reaction. Pipecolic acid, a metabolite in this pathway, has been found elevated in plasma, CSF, and urine of patients with pyridoxine dependent epilepsy.4
Another metabolite, α-aminoadipic semialdehyde, is found elevated in urine and is diagnostic for pyridoxine dependent epilepsy.3,5 Therefore it is no longer necessary to withdraw pyridoxine to confirm the diagnosis of pyridoxine-dependent epilepsy.6 Still, one has to remember that not all cases of pyridoxine dependent epilepsy are caused by mutations in ALDH7A1, as there are patients who do not show linkage to this locus.3,7 Negative biochemical testing thus does not preclude this diagnosis, and successful treatment should not be withdrawn because of negative metabolic or genetic testing.
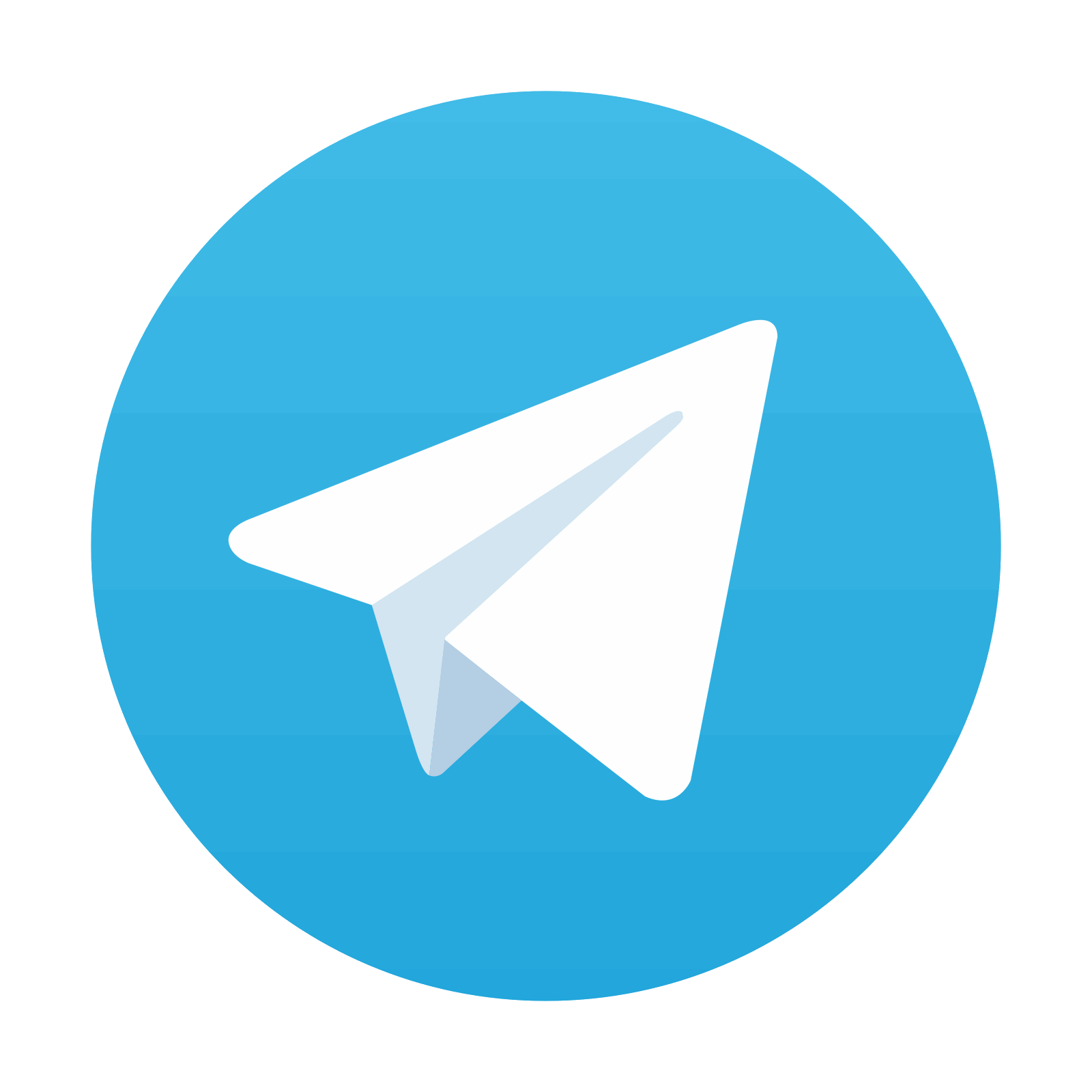
Stay updated, free articles. Join our Telegram channel

Full access? Get Clinical Tree
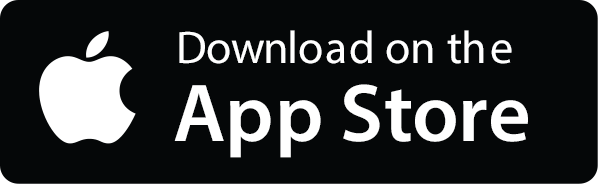
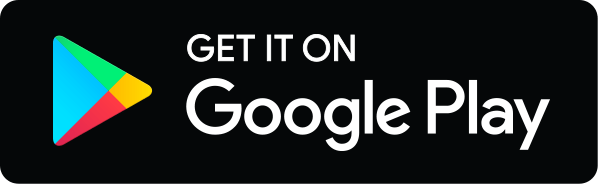