2
Essential Concepts in TBI Biomechanics and Neuropathology
Michelle C. LaPlaca
GENERAL PRINCIPLES
Traumatic Brain Injury as a Continuum
Traumatic brain injury (TBI) can be defined as transient or persistent brain dysfunction, occurring as the result of head movement and/or collision between the head and an object or surface that causes suprathreshold loading to the brain. TBI occurs as a continuum of events, from the traumatic insult to the primary injury to secondary injury to the clinical outcome. Biomechanics plays a critical role in understanding the TBI continuum.
• Traumatic insult: The external cause is categorized by the mechanism (e.g., motor vehicle collision) and the intent (e.g., unintentional) [1]. Traumatic loading typically occurs on the order of milliseconds to seconds.
• Primary injury: The immediate (milliseconds to seconds) result of the associated traumatic event. This is the mechanical response to the insult.
• Secondary injury: The cascade of changing biochemical and molecular events (seconds to weeks) that results from the primary injury.
• Injury outcome (weeks to years): Consequences of the primary and secondary injury, combined with recovery and repair of the brain. The ability to heal will depend on many factors, including metabolic state and endogenous repair capacity of the brain. The cell and tissue level events in the brain can manifest as sensory, motor, cognitive, behavioral, affective, systemic, and/or somatic conditions.
Confounding Factors in the TBI Response
The TBI response is the result of numerous interrelated processes that have inherent heterogeneity and complexity across time scales (the continuum) and system levels (subcellular [nano], cellular [micro], tissue/organ [meso], and systemic/organism [macro]).
Secondary injury mechanisms that mediate TBI pathologies are variable due to:
• Heterogeneity in traumatic insults and individual responses
• Complexity of interactions among cellular signaling pathways
• Constantly changing cellular activities and systemic adaptations [2]
• Periods of hyperactivity and hypoactivity
Types and Sources of Heterogeneity
TBI is extremely heterogeneous due to its nature [3]. Heterogeneity stems from the variation in the inherent nature of a system and/or in the response to a changing state.
A. Preinjury heterogeneity (innate, premorbid):
1. Innate heterogeneity (nonchangeable): individual characteristics such as sex, age, stature, genetic variation, and neuroanatomic and musculoskeletal variations
2. Premorbid factors (potentially changeable): individual preexisting medical conditions or disease (e.g., previous head injury exposure, cardiovascular disease); health state at time of injury (e.g., hydration, nutritional status, recent sleep pattern, stress state)
B. Event heterogeneity (biomechanics, environmental):
1. Biomechanics of the causative event: magnitude and direction of force and acceleration, surface area of impact (i.e., force distribution), loading duration
2. Environmental heterogeneity at the time of the event: temperature, humidity, head protection, impact surface, degree of anticipation
C. Peri-injury heterogeneity (early injury response, clinical intervention):
1. Primary injury or biomechanical response: dependent on tissue mechanical properties, tissue orientation, loading direction
2. Early secondary injury: complex cell signaling cascades dependent on type and number of cells affected, intrinsic cellular characteristics
3. Clinical intervention: variations in the time window to care and prehospital protocols
D. Postinjury heterogeneity (secondary injury, repair capacity, clinical management):
1. Secondary injury: overlapping signaling cascades, systemic influence
2. Repair capacity: innate and premorbid factors superimposed with the injury response and ability to stably repair (i.e., neuroplasticity)
3. Clinical management: variable stabilization protocols, treatment options, and rehabilitation strategies [4]
BIOMECHANICS AND PRIMARY INJURY
The event biomechanics (traumatic insult and resulting head movement) and the mechanical response (primary injury to the brain) are linked. For a TBI to occur, the traumatic load to the head must be transduced to the brain tissue. Force transduction to the tissue and cells is not well understood because each loading event and individual is unique. Details of the biomechanical conditions around a traumatic insult are typically not known.
Event Biomechanics: Load → Dynamic Head Response → Transduction to Brain → Brain Response
• Loading conditions: The forces, rates, and other parameters of the traumatic insult. Load is usually a combination of impact and inertial forces:
Impact load: Force resulting from a moving person striking a mass or obstruction, or a moving object or obstruction striking a person. The collision transfers energy and produces contact phenomenon (focal injury).
Inertial or impulsive load: Load resulting when a person is put in motion by an applied force. Unless there is rigid support, the head will rotate, with the neck as a pivot. Diffuse injury may result from the inertial load (especially high angular acceleration) and from the brain movement upon deceleration.
Penetrating load (e.g., bullet): It has high velocity and energy transfer over a small impact area.
Loading rate is important: Slowly applied force will result in lower stress than rapidly applied force.
The force produced by loading and subsequent head movement (acceleration pattern) will be transduced to the brain tissue and the cell components.
• Acceleration = velocity/time of the head can be angular or linear (usually a combination). An impact will cause the head to accelerate, then decelerate.
• Force = mass × acceleration. The lower the mass, the higher the acceleration must be to keep the force constant.
• Protective gear and safety measures aim to distribute and dissipate force.
The biomechanical response is a function of material properties and cell orientation and will dictate the primary injury.
• Material properties across brain regions vary [5]. The brain is a relatively soft tissue. Cell orientation and tissue composition (e.g., blood vessels, white matter tracts) contribute to differences in properties and overall tissue response.
• More force + softer tissue = more strain; suprathreshold force will deform tissue to the point of damage. Deformation (or strain) can be tensile (stretch), compressive, and/or shear (note: the brain is especially vulnerable to shear strain).
• Higher strain rate is more damaging than lower strain rate.
• Structural failure of the brain includes microtears, macrotears, compression, tension and/or shearing within and between brain regions, damage of vascular structures (resulting in bleeding) (meso and macroscale).
• Insults to the neural cells will, in turn, depend on mechanical response of the cells (strain and strain rate). Primary damage may manifest in neurons, glial cells, and vascular cells as axonal stretching, membrane disruption, ion imbalance, interruption in normal conduction and synaptic transmission, and glial damage (nano and microscale).
Injury threshold: The insult level at which structural and functional compromise or failure takes place; described using injury tolerance criteria. Development of tolerance criteria requires understanding of the relationship among traumatic insult, mechanical response of the brain tissue, and the resulting injury cascades and neuropathology.
SECONDARY INJURY
Primary structural damage (membranes and cell processes, intercellular connections) initiates and exacerbates secondary injury. The following general categories of secondary injury processes are interrelated:
A. Neurochemical and electrochemical imbalances (ions, neurotransmitters, cell signaling)
1. Disruption of ion homeostasis (Ca2+/Na+ influx) [6], ion channel dysfunction [7], leading to membrane potential changes, high energy demand, and mitochondrial overload.
2. Excess excitatory neurotransmitter release (e.g., glutamate); overactivation of glutamate receptors, leading to excitotoxicity [8].
3. Aberrant cell signaling: ion channel dysfunction, abnormal G-protein activation, and subsequent second messenger pathways, leading to abnormal action at downstream targets [9].
4. Electrophysiological abnormalities such as hyperexcitability, leading to network disturbances [10].
B. Impaired brain metabolism (oxygen and glucose availability and use)
1. Changes in cerebral perfusion result in hypoxia, exacerbating secondary injury [11].
2. Fluctuation in metabolic demand and glucose metabolism [12,13].
3. Impaired mitochondrial function, diminished glucose substrates, leading to reduced cellular respiration and less adenosine triphosphate (ATP) production, increases in CO2 (hypercapnia), H+ (acidosis).
C. Biomolecular degradation (enzyme activation, free radical attack)
1. Proteases attack cytoskeleton (e.g., calpain [14]), lipases upset lipid metabolism (e.g., phospholipase A2), endonucleases initiate nucleic acid damage.
2. Free radical production (reactive oxygen species [ROS]; reactive nitrogen species [RNS]). Increase in hydroxyl radicals causes lipid peroxidation and compounds degradative processes [15].
3. Secondary membrane damage (e.g., lipid peroxidation) impairs transport and signaling, as well as stability of membrane phospholipids [16].
D. Axonal damage and diffuse axonal injury (DAI) [17,18]
1. Axons are stretched during the traumatic insult, causing transient axolemmal permeability.
2. Both unmyelinated and myelinated axons are affected.
3. Cytoskeletal damage impairs axonal transport, leading to axonal swelling.
4. Oligodendrocyte damage may contribute to myelin degradation, further affecting white matter tracts and network function.
E. Vascular dysfunction (permeability, hemorrhage, reactivity) [19]
1. Blood–brain barrier (BBB) disruption causes infiltration of blood-borne proteins, cells [20].
2. Vascular leakage and microbleeding cause brain toxicity, hypoxia, coagulation, and hematoma formation.
3. Uncoupling of cerebral blood flow and metabolism, hyperemia, uncontrolled cerebral perfusion pressure, vasospasm, contribute to edema, and raised intracranial pressure.
F. Inflammation (central and peripheral contribution) [21,22]
1. Acute inflammatory response (release of cytokines, histamines, arachidonic acid, bradykinin, nitric oxide) can become chronic/degenerative.
2. Activation of microglia (increase in pro-inflammatory cytokines: e.g., IL-1-β, IL-6, TNF-α) [23].
3. Pro-inflammatory and anti-inflammatory processes both contribute to the overall response [24].
G. Cerebral edema [25]
1. Cerebral edema can occur when osmolality changes, resulting in fluid movement from one compartment to another.
2. Underlying causes are increased intravascular hydrostatic pressure (vasogenic edema), increased interstitial fluid pressure due to blood pressure and protein leakage/abnormal oncotic pressures (interstitial edema), and cellular swelling due to ionic imbalance and osmosis (cytotoxic edema).
H. Cell death and cytotoxicity [26]
1. Apoptosis: unscheduled “programmed” cell death; portions of cell death pathways are activated by the insult.
2. Necrosis: traditionally distinct from apoptosis; likely one of many death pathways; features are end stage; enzymatically driven; exacerbated by inflammation.
3. Autophagy: cell death through phagocytic processes [27].
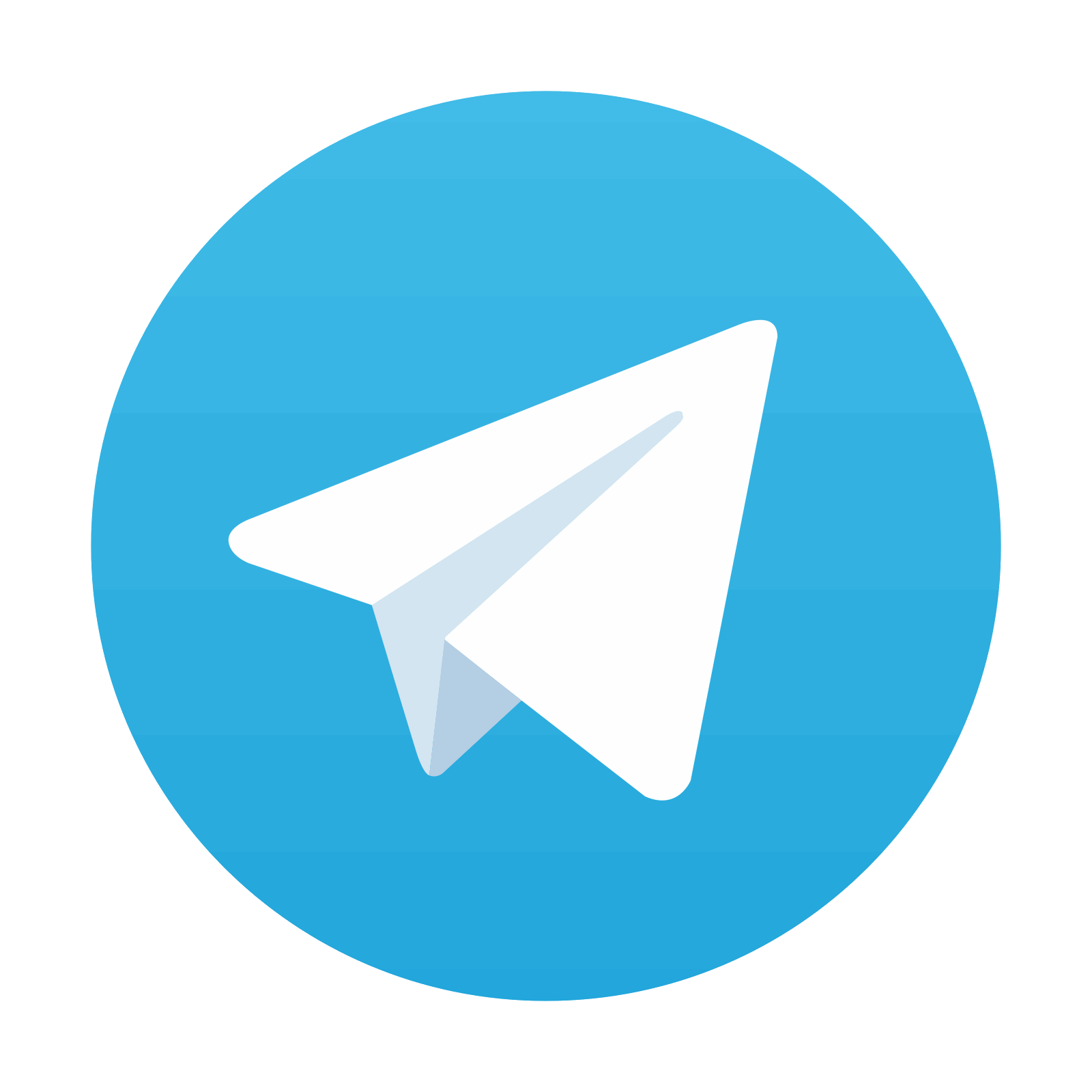
Stay updated, free articles. Join our Telegram channel

Full access? Get Clinical Tree
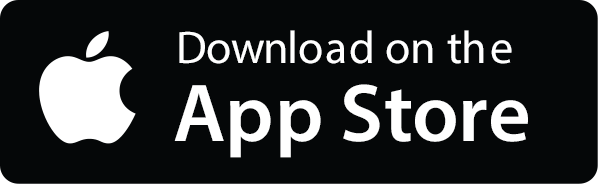
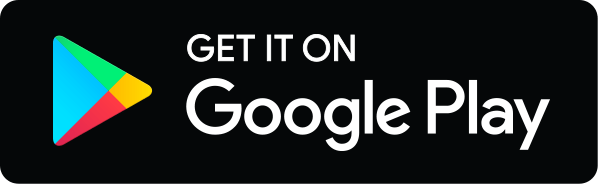