Introduction
Central nervous system (CNS) tumors encompass histologically diverse entities with widely varying prognostic and therapeutic implications. Within the brain, tumors can be divided into two main categories: primary and secondary brain tumors. Primary brain tumors arise from the brain parenchyma, meninges, nerves, or glands within the cranial cavity. In order to uniformly classify these tumors, the World Health Organization (WHO) has established an internationally accepted nomenclature and criteria based on histopathologic analysis of tumor tissue. The most recent update (WHO 2016 classification) has integrated molecular markers, which reflect genetic changes that are felt to underlie tumorigenesis, with well-established histologic criteria to classify gliomas (see Chapter 1 for molecular classification of brain tumors). Secondary brain tumors metastasize to the brain from another part of the body and are more common than primary brain tumors. The most common types of cancer that metastasize to the brain include lung, kidney, melanoma, and breast cancer.
Although ultimately an exact diagnosis frequently requires tissue confirmation, neuro-imaging is the initial step in the evaluation of patients with suspected CNS tumors. On initial presentation, patients often have clinical symptoms, such as seizures or hemiparesis, attributable to the location of the lesion or mass effect. Correlation of the patient’s neurologic symptoms with imaging is a critical step to initial management (i.e., steroid and anti-epileptic administration; surgical resection versus biopsy), as the ease of obtaining MRI these days has increased the risk of incidental findings. Furthermore, imaging can aid in creating an initial differential diagnosis of the suspected tumor and surgical planning.
This chapter provides an approach for the general neurologist or related practitioner to use when conducting an initial encounter of patients with different types of brain tumors. We focus on tumors that are commonly encountered in clinical practice. First, we present an overview of currently used standard and advanced imaging modalities in the evaluation of suspected brain tumors. Next, we present four clinical vignettes, each with a different type of tumor, in which we review imaging, prognosis, and initial management. Internists and neurologists are on the “front lines” of evaluating these patients and are often the first to identify patients with a suspected brain tumor. Our hope is that this chapter will improve familiarity with recognizing the characteristic radiographic appearance of common brain tumors, facilitating diagnostic testing in an expeditious fashion, as well as informing prognosis and different therapeutic strategies.
Imaging techniques
A CT or MRI of the head or brain is generally the first step in the evaluation of a patient who presents with neurologic symptoms. Although MRI is more sensitive for identifying CNS tumors, CT is usually much faster, cheaper, and more widely available—and therefore usually the first screening test for a brain tumor. In this section, we review pitfalls and pearls of both CT and MRI in the evaluation of brain tumors at time of diagnosis.
Computed tomography. A CT scan combines a series of x-ray images taken from different angles, and then processes these images through digital geometry processing to generate a three-dimensional cross-sectional view of the intended object. In addition to tumors, CT scans of the head are also used to detect hemorrhage, infarction, or bone trauma. Hyperdense structures (i.e., blood, calcifications) attenuate x-rays to a greater degree and will appear brighter on CT, whereas hypo-dense substances (i.e., air, infarction, edema) are radiolucent and will appear darker on CT. One important distinction is that of cytotoxic versus vasogenic cerebral edema. Cytotoxic edema, commonly seen in cerebral ischemia, is the result of an inability to maintain the ATP-dependent sodium/potassium membrane pumps on cell surface, which are responsible for the high extracellular and low intracellular Na+ gradient. When these pumps fail, Na+ accumulates within the cell and therefore draws in chloride (Cl−) and water, along an osmotic gradient—which in turn results in cellular swelling. There is no compromise of the blood-brain barrier (BBB). Imaging reveals effacement of cerebral sulci and edema affecting both grey and white matter. Vasogenic edema, seen in malignant brain tumors and cerebral abscesses, is the result of BBB disruption and resultant extracellular edema via leakage of fluid from capillaries. This generally affects white matter only and spares the outer cortical gray matter. On imaging, grey-white matter differentiation is maintained. In cases of persistent neurologic symptoms, hyperosmolar therapy (i.e., mannitol, hypertonic saline) is beneficial for cytotoxic edema, whereas corticosteroids are helpful for vasogenic edema.
The use of iodinated contrast with CT can improve detection of tumors, as abnormal enhancement can reflect disruption of the BBB and increased vascularity, which can be seen in malignant tumors. Despite these advantages, MRI is generally preferred over CT due to limitations with CT such as posterior fossa beam hardening artifact, poor soft tissue characterization, and inferior resolution (compared with MRI). CT, however, remains a reasonable alternative in patients with contraindications to MRI (i.e., MRI-incompatible implants [Autoimatic Implantable Cardioverter Defibrillator (AICD), pacemaker]; inability to lie still for a prolonged period).
Magnetic resonance imaging. MRI is based off of the magnetization properties of protons in the water molecules of tissue being examined. During an MRI scan, a powerful magnetic field is first used to align protons, which are normally randomly oriented, into one plane. This alignment is then perturbed by an externally applied radiofrequency (RF) pulse. After some time, the protons eventually return to their resting alignment through various relaxation processes and, in doing so, emit RF energy. These emitted signals are collected and processed through Fourier transformation to create a specific MRI pulse sequence. By varying the sequence of RF pulses applied and collected, different sequences are created. Different pulse sequences measure different aspects of molecular behavior, which in turn characterize various nervous system structures, such as grey and white matter, cerebrospinal fluid (CSF), and meninges.
The most common MRI sequences are T1-weighted and T2-weighted images. On T1-weighted images, grey matter is darker than white matter, and CSF is dark. On T2-weighted images, grey matter is brighter (i.e., more hyperintense) than white matter, and CSF is bright. The T2-fluid attenuated inversion recovery (FLAIR) sequence is a T2-weighted image with an additional RF pulse that suppresses the bright signal of CSF. Inflammation (i.e., edema, demyelination) is dark on T1-weighted images and bright on T2-weighted and FLAIR images. In clinical practice, the T2-weighted or FLAIR images are useful to track treatment response in low-grade gliomas, or to qualitatively assess for vasogenic edema in high-grade gliomas and lymphomas.
T1-weighted images can also be performed while injecting gadolinium, a paramagnetic contrast enhancement agent. In general, fat, protein, hemorrhage, melanin, and gadolinium contrast will be bright on T1-weighted images. The use of gadolinium with MRI is helpful in evaluating BBB breakdown, which is implicated in high-grade gliomas and lymphomas. Longitudinal comparison of T1-weighted post-contrast MRIs is the current standard of care in evaluating treatment efficacy in high-grade gliomas and lymphomas. This method, however, is not always reliable in brain tumor evaluation, as increasing contrast enhancement can be seen in both true tumor progression (i.e., active tumor) and post-treatment inflammatory changes associated with radiation, targeted therapy, or immunotherapy (i.e., dying tumor). The latter is known as pseudoprogression, and is commonly seen in MGMT-methylated gliomas that have recently undergone treatment with radiation and temozolomide. Imaging changes may also lead to tumor pseudoresponse—which reflects a rapid decrease in contrast enhancement and edema after administration of anti-angiogenic therapy (i.e., bevacizumab). In such cases, there is generally no real change in actual tumor size as seen on T2-weighted imaging but contrast enhanced sequences are dramatically improved. Due to these issues, several radiographic response criteria including the Response Assessment in Neuro-Oncology criteria (RANO) and Immunotherapy Response Assessment in Neuro-Oncology criteria (iRANO) have been developed to standardize assessment of tumor response and progressive disease. Additional advanced imaging modalities that reflect an underlying tumor’s physiologic response or genomic and molecular changes are under development and may be a solution for this response assessment conundrum in the future.
Diffuse-weighted and susceptibility weighted MRI. Two advanced MRI sequences frequently encountered in clinical practice are diffusion-weighted imaging (DWI) and susceptibility-weighted imaging (SWI). DWI is a measure of the random Brownian motion of water molecules within different tissues. This free movement of water is restricted in several pathologic conditions, such as acute ischemic stroke, abscess, and hypercellular tumors such as lymphoma and, occasionally, high-grade gliomas. On imaging, “restricted diffusion” manifests as high signal on isotropic images (DWI) and corresponding low apparent diffusion coefficient (ADC) values for the voxel in question. SWI is an MRI sequence that is particularly sensitive to compounds with paramagnetic and diamagnetic properties that distort the local magnetic field. The most common clinical use of SWI is for detection of small amounts of blood products or calcifications that may not be picked up on other MRI sequences or CT. The presence of a microhemorrhage within a brain tumor can be associated with high-grade gliomas, or with parenchymal tumors recently treated with radiation. Calcifications within tumors can be seen in lower-grade meningiomas or oligodendrogliomas, as these entities are more indolent and chronic in nature. Notably, different MRI vendors have different names for these sequences and there may be slight differences in how the images are acquired. For example, a gradient echo (GRE) sequence provides similar information as an SWI sequences.
Positron emission tomography (PET). Due to increasing awareness that standard post-contrast anatomic MRI can be misleading for brain tumor assessment, other imaging modalities are currently being explored. Positron emission tomography (PET) is a nuclear medicine functional imaging technique that can illustrate aspects of tumor metabolism. At present, there are two main classes of radiotracers: glucose metabolism tracers and amino acid transport tracers. 18 F-2-fluoro-2-deoxy-D-glucose ( 18 F-FDG) is a widespread and relatively cheap glucose metabolism tracer that was previously felt to be useful in delineating high-grade gliomas, given a positive correlation between glycolysis rate and malignancy. Unfortunately, due to high physiologic glucose metabolism within the brain, 18 F-FDG has poor spatial and diagnostic accuracy for gliomas. Due to relatively low tracer uptake in normal grey matter, amino acid PET tracers (i.e. 11 C-methyl-methionine [ 11 C-MET] and 3,4-dihydroxy-6- 18 F-fluoro-L-phenylalanine [ 18 F-DOPA]) can detect gliomas with greater sensitivity and may be helpful in differentiating progression from pseudo-progression. , These tracers, which are still under development, currently have major limitations (i.e., short half-life requiring close proximity to cyclotron, nonspecific uptake in other areas) that limit clinical use.
MR spectroscopy (MRS) and perfusion MRI (pMRI). Other sequences that are currently incorporated in clinical practice are MR spectroscopy (MRS) and perfusion MRI (pMRI). MRS is used to study the metabolic changes within the tissue in question. This sequence determines relative concentrations of a variety of cellular metabolites, such as choline (associated with membrane turnover and synthesis), creatine (associated with energy metabolism), N -acetyl-aspartate (NAA, associated with neuronal health), and lactate (associated with anaerobic glycolysis). High-grade gliomas are associated with a low NAA and creatine peak, and elevated choline peak—which correlate with increased membrane turnover and neuronal loss. In clinical practice, although MRS does correlate with tumor type and grade, it is not sufficiently sensitive or specific enough to replace histologic diagnosis, nor is it able to reliably differentiate between pseudoprogression and true tumor progression. pMRI assesses cerebral microvasculature by modeling the concentration of blood in different voxels. Kinetic analysis of this data enables quantitation of several physiologic biomarkers, such as cerebral blood flow, cerebral blood volume, and mean transit time. These measures are sometimes helpful in directing brain biopsy to the most aggressive portion of a lesion, distinguishing radiation necrosis from progressive tumor, and assessing response to anti-angiogenic agents (i.e., bevacizumab). In clinical practice, even with perfusion imaging, tissue analysis is still frequently required, and guidelines for pMRI data acquisition and processing have not been standardized across centers.
Clinical cases
Case. A 71-year-old female has a history of localized non–small-cell lung cancer under remission. She presents to medical attention after a first-time generalized tonic-clonic seizure. She denies fevers or recent infections. From a lung cancer perspective, this was diagnosed 5 years ago, and she underwent a video-assisted thoracoscopic surgery with right middle lobe resection. Staging systemic imaging revealed no sites of metastases. Since then, she has been monitored with surveillance imaging, which has demonstrated continued remission.
After the patient presented to the emergency department (ED) for new seizure, a brain MRI with and without gadolinium contrast was obtained. This revealed an intra-axial right frontal lobe lesion that measured approximately 3.9 × 3.6 × 4.2 cm, and was associated with vasogenic edema ( Fig. 6.1 ). The radiologic differential was felt to include a high-grade glioma versus less likely a metastasis or abscess. After imaging, a neurosurgical consultation was requested. The patient was started on levetiracetam 750 mg bid and dexamethasone 4 mg bid, and admitted to the hospital. Three days later, she was taken to the operating room for a gross total resection of contrast-enhancing disease. Histopathologic analysis revealed a glioblastoma (GBM). She was discharged from the hospital 2 days afterwards, and was referred to Radiation Oncology and Neuro-oncology for consideration of fractionated radiation and chemotherapy.

Teaching Points: Imaging Features of Supratentorial GBM. This case illustrates several classic features of GBM. GBM is a WHO grade IV malignant astrocytic tumor characterized by necrosis and neovascularity. It is the most common primary malignant intracranial neoplasm, and has an estimated incidence of 15,000 new cases yearly in the United States. Headaches (50–60%), seizures (20–50%), and focal neurologic deficits (10–40%) are the most common presenting signs of a GBM. Fig. 6.1 is a characteristic appearance of a GBM. T2/FLAIR imaging often demonstrates a heterogenous and hyperintense mass with adjacent tumor infiltration and vasogenic edema, although it can be difficult to distinguish infiltrative tumor from perilesional edema. T1 post-contrast imaging generally illustrates thick, irregular ring-enhancement surrounding an area of central necrosis. Although not seen in this case, there is often involvement of the corpus callosum, which signifies involvement of the white matter tracts and spread to the contralateral hemisphere (e.g., “butterfly” lesion). Corpus callosal involvement is more commonly associated with GBM and lymphoma, and rarely for metastases or strokes. There is usually no diffusion restriction. SWI often has artifact that correlates to the area of central necrosis seen on T1 post-contrast imaging.
Imaging differential for HGG . Other considerations on the imaging differential for GBM include a solitary brain metastasis, abscess (given ring-enhancing lesion, though should have prominent diffusion restriction), HIV-related primary CNS lymphoma, “tumefactive” demyelination, or subacute ischemia. This patient’s history of lung cancer does increase clinical suspicion for metastasis; however, she had a locally treated cancer that was likely cured. There are, however, some cancers such as melanoma or renal cell carcinoma that can appear years after a primary tumor has been treated and the patient is in remission. Although it can be difficult radiographically to discern a solitary metastasis from GBM, metastases are generally smaller, located in the grey-white junction, and do not have as much vasogenic edema. GBM tends to have irregularly shaped contrast enhancement and FLAIR hyperintensities due to its predilection for spread along white matter tracts.
For this patient, we note the lack of diffusion restriction and rather thick contrast enhancement is not consistent with an abscess. A demyelinating lesion would be atypical in a patient of this age range. Additionally, there was not a history of an abrupt-onset neurologic deficit several days ago, and to have such extensive vasogenic edema would be uncommon for a stroke. This being said, unusual presentations of all the above can occur. To date, there is no advanced imaging modality (i.e., MRS, pMRI, diffusion tensor imaging) that can accurately diagnose a GBM and thus supplant the need for tumor tissue in diagnosis.
Patient management . Standard of care for adjuvant treatment of GBM and other high-grade gliomas is covered in a separate section. On initial presentation, we emphasize the importance of an urgent neurosurgical consultation. The goal of surgery is to confirm a pathologic diagnosis and to achieve maximal safe resection consistent with preservation of neurologic function (see Chapter 2 , Case 2.2 for further discussion of surgical management of high-grade brain tumors). Doing so provides the benefit of cytoreduction, prior to adjuvant radiation and chemotherapy, which has demonstrated improved outcomes—compared with a biopsy only. Furthermore, maximal safe resection will provide adequate tissue through which molecular analyses can be run, and sampling bias can be avoided. Subtotal resection or stereotactic biopsy may be required, however, depending on the location and extent of the tumor and the patient’s functional status. If the suspicion for a high-grade glioma is high, a CT chest/abdomen/pelvis (to assess for a systemic malignancy) can be deferred given the need for tissue diagnosis. Once the diagnosis of GBM is confirmed, patients should start fractionated radiation and systemic therapy within 4–6 weeks of surgery, as per National Comprehensive Cancer Network guidelines.
Clinical Pearls
- 1.
The imaging differential for a single ring-enhancing supratentorial lesion includes high-grade glioma, solitary brain metastasis, cerebral abscess, tumefactive demyelination, and subacute stroke.
- 2.
For such a lesion, surgical consultation and evaluation are required to establish a tissue diagnosis and guide treatment decisions.
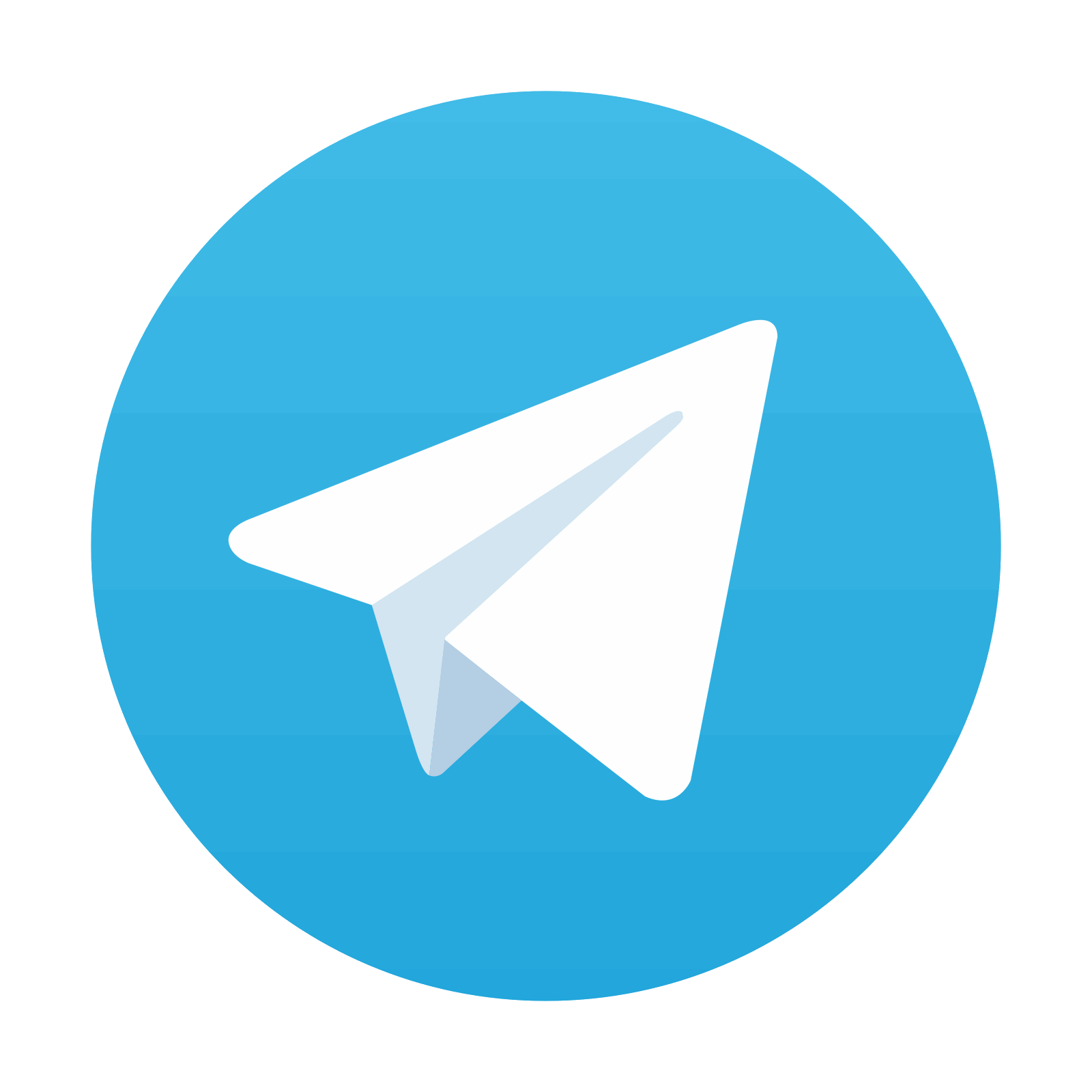
Stay updated, free articles. Join our Telegram channel

Full access? Get Clinical Tree
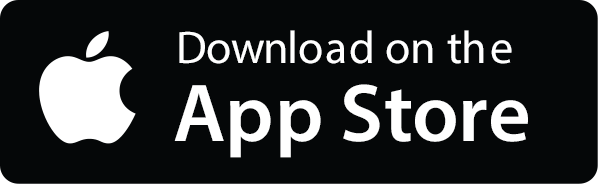
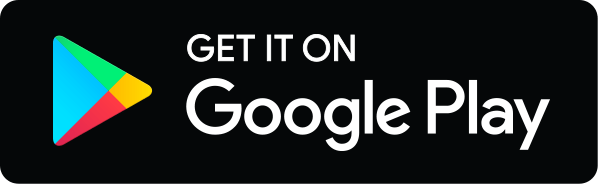
