Chapter 22 During the past two decades, neuroendovascular therapy has exponentially evolved into a medical specialty of its own driven by a heterogeneous group of specialists that includes cardiologists, neurologists, radiologists, neurosurgeons, vascular surgeons, and neuroscientists in general. This evolution has been largely induced by improving materials science technology that has allowed for the development of novel devices and delivery tools that are enhanced, safe, and effective. For many indications, neuroendovascular therapy has become the standard of care, replacing or complementing traditional gold standard therapies, such as carotid endarterectomy, surgical clip ligation of intracranial aneurysms, and intravenous (IV) thrombolysis of acute stroke. Carotid stenting, coiling of intracranial aneurysms, and intraarterial (IA) thrombolysis/thrombectomy are prime examples of the ongoing endovascular evolution.1–4 In this chapter, we focus on the newest technical developments and evolving technology of endovascular therapy with respect to intracranial revascularization for cerebral ischemia. Many endovascular revascularization techniques are derived from applications for treatment of peripheral or coronary vascular disease. However, unlike coronary and peripheral vessels, cerebral vessels are curved and lack structural support, being surrounded only by cerebrospinal fluid. The composition of the arterial walls is different, with intracranial arteries having loss of vasa vasorum and external elastic membranes, near absence of the adventitia, and a tunica media composed principally of smooth muscle cells.5–8 Visualization of the intracranial vasculature has limitations. The bony cranium obscures the view. Frequently, because of vessel tortuosity, multiple angles are required to properly visualize intracranial vessels. Absolute measurements of cerebral blood flow remain a challenge. Access to the intracranial circulation can be dramatically limited because of tortuous aortic arch variations (type II or type III aortic arch). Endovascular therapy for intracranial disease, therefore, requires special devices and skills. Heterogeneity of specialists with very different training experiences substantiates this limitation. Thus, one direction of the new development is the invention, production, and application of newer catheters allowing proximal access (aortic arch, common carotid arteries) and distal access (intracranial platform catheters). In addition, modification of existing technology (i.e., coronary stents) to allow intracranial navigation (i.e., increased flexibility, softness) is another direction of the technical advancement. Further, the scientific evidence (prospective multicenter randomized controlled trials) supporting the application of endovascular procedures is still limited. A large body of evidence for neuroendovascular approaches is derived from studies that are retrospective and mostly anecdotal in nature. Because of the constant and rapid evolution/development of newer methods, evidence-based medicine lags behind. Thus, there is currently a large effort to increase level I clinical evidence to support the application of endovascular techniques as a standard of care for multiple indications, including intracranial revascularization for ischemic disease, particularly stroke. Another limit is the local availability and acceptance of endovascular technology and techniques. Frequently, institutional protocols govern the endovascular practice. Many hospitals still do not have the basic equipment (i.e., biplane angiography) to perform neuroendovascular procedures safely. The lack of these modalities is slowing the spread of endovascular evolution throughout the country as well as internationally. In summary, current endovascular therapy has some limitations, including proper visualization, technical access to the intracranial space, navigability of tools, heterogeneity of operator skills and training, scientific (databased) validation, and local availability of the technology. But truly, only imagination is the limit for what the future holds for this field. Intravascular ultrasound (IVUS) is a medical imaging modality that uses a specially designed catheter with a miniaturized ultrasound probe attached to the distal end of the catheter. The proximal end of the catheter is attached to computerized ultrasound equipment. IVUS allows the application of ultrasound technology to see from inside blood vessels out through the surrounding blood column, visualizing the luminal wall structure of the blood vessels. This imaging modality has become an important diagnostic and therapeutic adjunct for coronary artery angioplasty and stenting. It is used in the coronary arteries to determine the amount of atheromatous plaque built up at any particular point in the epicardium and is useful in the determination of plaque volume within the wall of the artery and/or the degree of stenosis of the artery lumen. It can be especially useful in situations in which angiographic imaging is considered unreliable, such as for the lumen of ostial lesions or where angiographic images do not visualize lumen segments adequately, such as regions with multiple overlapping arterial segments. IVUS is also used to assess the effects of treatments of stenosis, such as with hydraulic angioplasty expansion of the artery, with or without stents, and the results of medical therapy over time. It has been increasingly used in research to better understand the behavior of the atherosclerosis process in humans. We routinely use IVUS to evaluate carotid atherosclerotic disease before carotid angioplasty and stenting to establish plaque characteristics that may indicate high and low risk plaques in terms of plaque stability. Similarly, after carotid angioplasty and stenting but prior to removal of proximal or distal protection, we routinely perform IVUS to visualize any intraluminal thrombus, in particular to evaluate the cheese grating effect of the stent.9 We have used IVUS to assist intracranial angioplasty and stent placement in a patient who underwent angioplasty and stenting for high-grade restenosis of a basilar artery atherosclerotic lesion and in another patient who underwent stenting to treat an occlusive dissection of the left internal carotid artery (ICA) that occurred during arteriovenous malformation embolization; in each case, IVUS provided important information in terms of lesion evaluation, stent selection, and stent placement.10 Currently, however, the IVUS catheter, which measures only 1 mm in diameter, is still too stiff to be delivered reliably into the intracranial circulation, particularly into the supraclinoidal anterior circulation. Optical coherence tomography (OCT) is a novel imaging system that is analogous to ultrasound, but imaging is performed with light instead of acoustic waves. OCT measures light reflected from turbid structures.11–13 This technology is based on principles of low-coherence interferometry allowing the detection of back-scattered near-infrared photons from selected depths and rejection of photons being scattered from other layers. Unlike direct reflective techniques (such as angioscopy), it visualizes the depth at which back-scattering occurs. OCT provides three-dimensional information about the scattering properties of living tissue. The spatial resolution that can be reached is near-histologic (5–8 micron) scale, which makes OCT suitable for in vivo optical biopsy, allowing for the visualization of tissue cross-sectional microstructure, even without a contrast agent. Because signals from different depths are separated by the measurement approach, OCT can detect deeper events more easily than a reflection approach. Endovascular imaging using this technology has mainly been performed in coronary arteries. OCT imaging identifies the structural components of atherosclerotic plaques such as lipid-rich areas, fibrous content, calcified portions, and inflammatory plaques, and has been successfully used to identify thin-capped fibroatheromas and distinguish them from thick-capped and fibrous plaques.14–18 OCT imaging enables visualization of stent tines postdeployment, stent-wall apposition, poststent stenosis, as well as microdissections and luminal/mural thrombus.14–18 Feasibility studies on the use of OCT for neuroendovascular imaging in patients have been reported, although further literature in this relatively new field is scant.11,12 Potential uses include diagnostic imaging of atherosclerotic plaques as well as of endovascular neurointerventions, such as aneurysm coiling and stenting. Similar to IVUS, development of pliable and trackable OCT delivery catheters designed for intracranial delivery hold the promise to be revolutionary during endovascular therapies for intracranial and extracranial atherosclerosis. Because of its higher resolution, OCT may provide greater data on plaque stability during extracranial atherosclerotic disease management with angioplasty and/or stenting. It could be highly valuable in the identification of true versus false lumens during endovascular management of dissections, particularly during management of intracranial dissections with stents where deployment of a device in the false lumen may have disastrous consequences. Again because of its higher resolution, this technique has the potential to deliver greater information about intracranial atherosclerotic plaques as well as thrombus morphology during revascularization of acute stroke. In cases of stent deployment, important information about stent-to-vessel wall apposition and presence of intraluminal thrombus may be obtained with OCT imaging. The safety of a percutaneous vascular intervention largely depends on accurate visualization of both the device and the surrounding anatomic structures. Fluoroscopic images provide high temporal and spatial resolution of the vascular lumen. By necessity, neuroendovascular fluoroscopic imaging is endoluminal, yielding very little information about the vascular wall and surrounding tissue. Both the patient and the examiner are exposed to radiation. Radiation exposure has immediate and long-term consequences from alopecia and skin burn to neoplasia. This is of particular importance to interventionists who spend many hours every day in an environment with an abundance of radiation. Magnetic resonance imaging (MRI) guidance has the potential to offer soft tissue contrast to visualize the vessel lumen, the vessel wall, and the surrounding structures; and this guidance can provide flow velocity data without any radiation exposure. Gadoliniumbased preparations have very short intravascular retention time, and administration of the maximal approved dose of these preparations can be achieved during an interventional procedure. Wacker et al19 were able to perform catheterization, angioplasty, and stenting in abdominal and renal vessels as small as 2 mm in pigs using MRI guidance with ultrasmall superparamagnetic iron oxide SH U 555 C as an intravascular contrast agent. Therefore, a huge potential exists for radical changes in the way interventions are performed, making them safer for both the patient and the interventional staff in the interventional suite. However, many hurdles remain before MRI guidance for neuroendovascular interventions becomes a reality. MRI hardware and sequence design have to be developed to achieve acceptable patient access and to allow real-time imaging. Further, interventional devices that allow for adequate visualization, safety, and effectiveness have to be designed for the heavy magnetic field environments. The current direction of pharmacologic therapy for acute stroke is thrombolysis with a variety of agents resembling recombinant tissue plasminogen activator (rtPA) that can be administered IV or IA. Standard medical therapy currently approved for acute stroke by the U.S. Food and Drug Administration (FDA) and the European Union is IV alteplase (rtPA). However, IV rtPA treatment for acute stroke remains far from ideal for multiple reasons. Few patients (~3%) receive IV rtPA treatment because of the narrow window for intervention and broad ineligibility criteria.20 Moreover, the recanalization rates from IV rtPA are of little benefit – only 10% for carotid occlusion and 30% for proximal middle cerebral artery (MCA) occlusions. The rate of clinical improvement and outcomes after IV rtPA are not satisfactory at this time (modified Rankin scale score of ≤2 in 36%).21 All these reasons imply a need for more effective treatment strategies. IA thrombolysis alone or in combination with IV rtPA has shown promise in an acute setting in patients with thrombotic stroke.1,22,23 The IA approach is associated with higher recanalization rates using a lower dose of thrombolytic agent and has extended the time window for treatment. Newer and experimental thrombolytic agents with different mechanisms of action are being used and explored for IA thrombolysis, including plasminogen activators, direct fibrinolytics, and fibrinogenolytic agents, as summarized in Table 22.1.
Evolving Technology for Endovascular Revascularization
Current Limitations of Endovascular Revascularization
Intravascular Ultrasound
Optical Coherence Tomography
Magnetic Resonance Imaging–Guided Endovascular Procedures
Advances in Intraarterial Pharmacology
Plasminogen Activators |
|
Monteplase | Not investigated in stroke |
Lanoteplase | Not investigated in stroke |
Pamiteplase | Not investigated in stroke |
Staphylokinase | Not investigated in stroke |
Desmoteplase | Promising in some studies; from saliva of the vampire bat; more selective and potent |
Fibrinolytics |
|
V10153 | Recombinant human plasminogen activated to plasmin |
Microplasmin Alfimeprase | Isolate from southern copperhead snake venom |
Fibrinogenolytics |
|
Ancord | Isolate from Malayan pit viper venom; inactivates fibrinogen |
Newer Revascularization Technologies
Acute Thrombolysis Augmentation Technologies
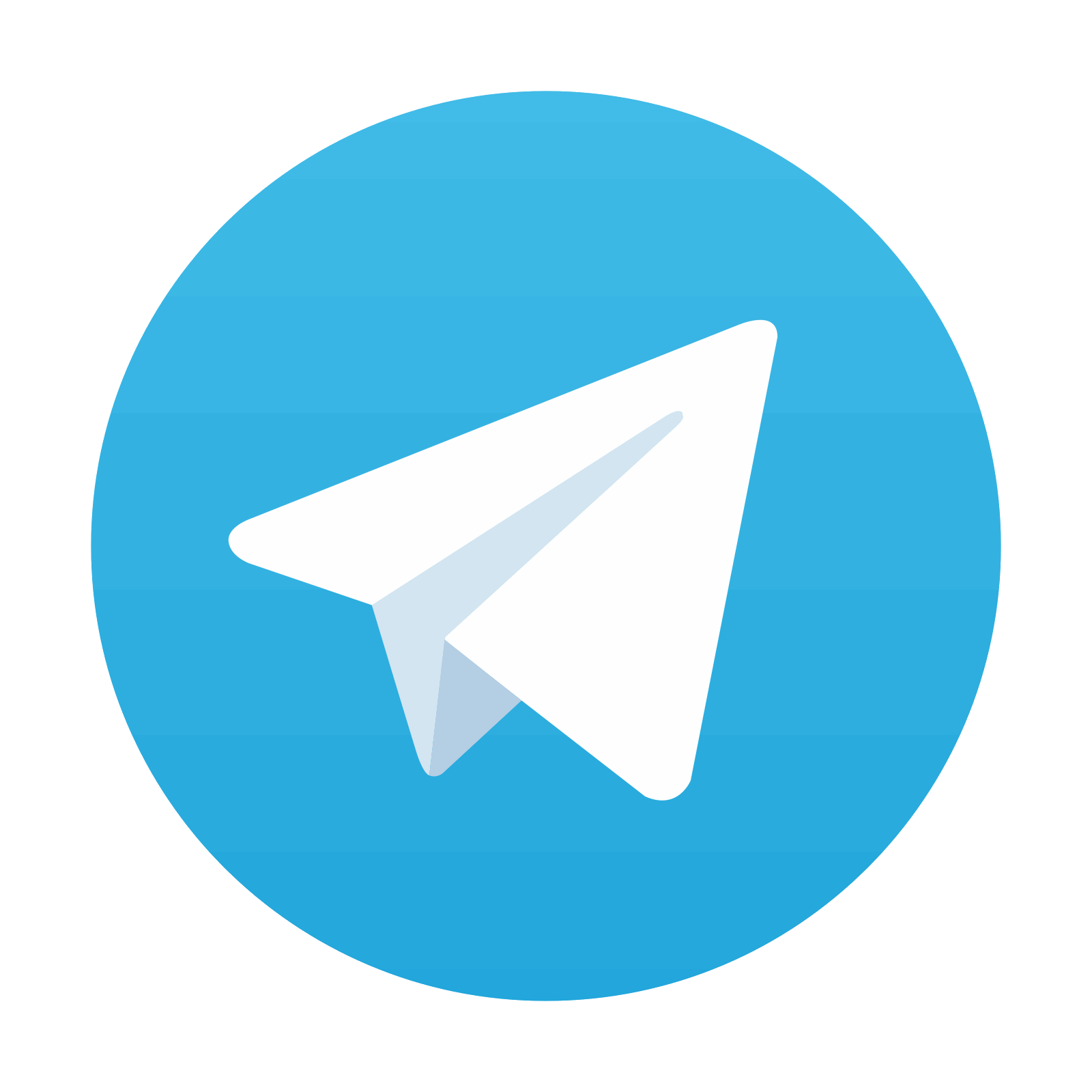
Stay updated, free articles. Join our Telegram channel

Full access? Get Clinical Tree
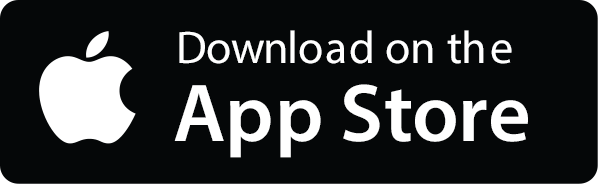
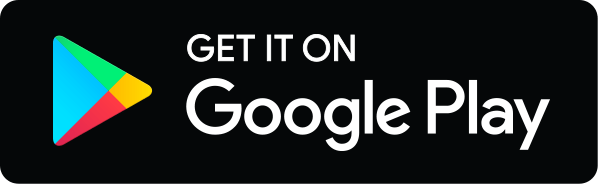