Excitatory Synaptic Transmission
Karen S. Wilcox
Peter J. West
Marc A. Dichter
Introduction
Information processing throughout the central nervous system (CNS) occurs through synaptic transmission between neurons coupled via chemical synapses. Excitability at chemical synapses is controlled by both the amount of excitatory neurotransmitter released and by the way in which the postsynaptic neuron responds to the released transmitter. Multiple regulatory mechanisms exist within the CNS to maintain a controlled and focused level of excitability and to prevent excess excitation. These include mechanisms intrinsic to excitatory systems and mechanisms extrinsic to these systems; regarding the latter, direct inhibition and indirect modulation are noteworthy. Excess activation of excitatory pathways in specific circuits can lead to epileptic activity, neuronal damage, and even cell death. This chapter discusses what is known concerning the inotropic and metabotropic glutamate receptors that underlie excitatory synaptic transmission and the role(s) that they play in epilepsy.
Excitatory Neurotransmitters
A variety of endogenous compounds have been identified as agonists at excitatory amino acid (EAA) receptors. However, the two EAAs that are thought to be the most likely neurotransmitter candidates are L-glutamate and L-aspartate. Both compounds act as agonists at all subtypes of inotropic glutamate receptors. Other endogenous compounds present in the CNS that act as agonists at EAA receptors and can depolarize neurons include N-acetylaspartylglutamate (NAAG), quinolinic acid, and the sulfur-containing amino acid analogs of glutamate: Cysteic acid, homocysteic acid, cysteine sulfinic acid, homocysteine sulfinic acid, and S-sulfocystein.28 Whether any or all of these act as neurotransmitters at specific synaptic sites remains to be determined.
Glutamate Synthesis and Uptake Mechanisms
Glutamine and α-ketoglutarate are thought to be the major precursors for glutamate, which is subsequently packaged into vesicles for future release into the synaptic cleft. Glutamine is taken up into the presynaptic terminal via an active, sodium (Na)-dependent uptake protein. It is then transported to mitochondria, where it is converted via phosphate-activated glutaminase to glutamate and ammonia. α-Ketoglutarate is also actively taken up into the presynaptic terminal, where it is transaminated into glutamate. The glutamate anion in the terminal is then actively taken up into vesicles for future release. Upon release into the cleft, the glutamate either is actively taken back up via a neuronal glutamate transporter and repackaged, or it diffuses away from the cleft and glial glutamate transporters, most often excitatory amino acid transporter (EAAT)-2, internalize the extracellular glutamate.17
Once in astrocytes, the glutamate can either be (a) released again into the synaptic cleft via a calcium-dependent vesicular release,13 (b) metabolized via glutamine synthetase into glutamine, or (c) metabolized into α-ketoglutarate by either glutamate oxaloacetate transaminase or glutamate dehydrogenase. This glutamine and α-ketoglutarate is then actively transported out of the glial cells and back into the presynaptic terminals for subsequent resynthesis of glutamate (Fig. 1).51 Recent work by a number of groups suggests that patients with temporal lobe epilepsy (TLE) may have a deficiency in glutamine synthetase in the astrocytes of sclerotic tissue. This reduced glutamine synthetase may underlie the elevation in extracellular glutamate often associated with TLE47,155 by reducing the rate of glutamine–glutamate cycling.119
Clearly, glutamate uptake molecules perform a vital function in maintaining large precursor levels for glutamate synthesis and low extracellular concentrations of glutamate. To date, five high-affinity glutamate transporter proteins have been cloned.17,66,67,120,145 All five transporter proteins have been shown to be sodium-dependent, and they are preferentially located in neurons, astrocytes, or other types of glial cells. The recent development of highly specific antagonists of these glutamate transporters will prove quite valuable in ascertaining the contribution these transporters make to neurotransmission at excitatory synapses.
Ionotropic Excitatory Amino Acid Receptors
Three pharmacologically distinct classes of inotropic glutamate receptors (iGluRs) have been identified. The names of these iGluR subfamilies are based on the synthetic agonists that bind to the specific receptor subtypes and selectively open the associated ion channels: The α-amino-3-hydroxy-5-methyl-4-isoxazole propionic acid (AMPA) receptor, the kainic acid (KA) receptor, and the N-methyl-D-aspartate (NMDA) receptor. This initial pharmacologic classification was supported by the subsequent identification of separate gene families whose sequence similarities were correlated with their affinity for the agonists previously described: GluR1 through GluR4 (which encode AMPA receptor subunits); GluR5 through GluR7; KA1 and KA2 (which encode low- and high-affinity KA receptor subunits, respectively); and NR1, NR2A through NR2D, and NR3A and NR3B (which encode NMDA receptor subunits). Two orphan receptor subunits, GluRδ1 and GluRδ2, have also been identified.69
All iGluR subunits have an extracellular N-terminus, an intracellular C-terminus, three transmembrane domains (M1, M3, M4), and a membrane re-entrant loop (M2) that forms the pore (Fig. 2). The ligand binding domain of iGluRs is formed by
stretches of amino acids in the N-terminal domain (S1) as well as the linker between M3 and M4 (S2). This ligand binding domain has been crystallized in various functional conformations, and has provided insight into key features of glutamate receptor agonist affinities, activation, desensitization, subunit association, and receptor assembly. Several articles offer excellent in-depth review of this topic.86,92,93 Functional receptor-gated ion channels are formed when subunits within, but not between, families come together to form homo- or hetero-oligomers. Although the precise subunit stoichiometry of iGluRs was debated for some time, recent studies now suggest that these receptors are composed of four subunits.91 The reader is directed to several excellent reviews on this topic.42,69,139
stretches of amino acids in the N-terminal domain (S1) as well as the linker between M3 and M4 (S2). This ligand binding domain has been crystallized in various functional conformations, and has provided insight into key features of glutamate receptor agonist affinities, activation, desensitization, subunit association, and receptor assembly. Several articles offer excellent in-depth review of this topic.86,92,93 Functional receptor-gated ion channels are formed when subunits within, but not between, families come together to form homo- or hetero-oligomers. Although the precise subunit stoichiometry of iGluRs was debated for some time, recent studies now suggest that these receptors are composed of four subunits.91 The reader is directed to several excellent reviews on this topic.42,69,139
AMPA Receptors
Molecular Biology
Post-transcriptional Modification.
The AMPA receptor subunits GluR1 through GluR4 are each comprised of approximately 900 amino acids and share approximately 70% sequence homology. Additional diversity among the subunits can be generated by post-transcriptional modifications (alternative splicing and RNA editing). All four AMPA receptors undergo alternative splicing of a 38-amino acid sequence in the extracellular domain between M3 and M4. This gives rise to two splice variants, “flip” and “flop.”141 These receptors exhibit differing biophysical and pharmacologic characteristics; the flip variant tends to desensitize more slowly and to a lesser extent than do flop variants, which can influence the amplitude of the AMPA receptor responses.104 Flip variants also have a greater sensitivity to the allosteric modulator cyclothiazide than do the flop variants. Expression levels of these variants are regulated developmentally, regionally, and in a cell-type–specific manner, and are also modified by disease states such as epilepsy.
In addition to the flip and flop splice variants, the GluR1, GluR2, and GluR4 AMPA receptors can undergo alternative splicing of their C-termini. This splicing results in isoforms with “long” and “short” cytoplasmic C-terminal domains, and these variants are named as such. Variation in the length of these receptors’ C-terminal domains can influence their interactions with cytoplasmic proteins through the presence (“short” C-termini) or absence (“long” C-termini) of a PSD-95/Disc-large/ZO-1 (PDZ) binding domain.39
It has been observed that homomeric or heteromeric AMPA receptors that lack the GluR2 subunit are permeable to calcium and exhibit a voltage-dependent block by intracellular polyamines that results in an inwardly rectifying current-voltage (IV) relationship. The ability of the GluR2 subunit to influence these AMPA receptor properties resides in a single amino acid substitution (the genomically encoded glutamine is converted to arginine) in the pore forming M2 segment. This Q/R substitution is the consequence of post-transcriptional editing of premessenger RNA.142 AMPA receptors that contain edited GluR2(R) are impermeable to
calcium, have a low single-channel conductance, and have linear IV relationships.147 Most neurons in the adult CNS have been shown to possess AMPA receptors with these qualities, and this suggests that the GluR2(R) subunit is a constituent of most native AMPA receptors. One notable exception to this rule is receptors expressed in various interneurons that appear to be calcium permeable, inward-rectifying, and are therefore believed to lack the GluR2 subunit.73
calcium, have a low single-channel conductance, and have linear IV relationships.147 Most neurons in the adult CNS have been shown to possess AMPA receptors with these qualities, and this suggests that the GluR2(R) subunit is a constituent of most native AMPA receptors. One notable exception to this rule is receptors expressed in various interneurons that appear to be calcium permeable, inward-rectifying, and are therefore believed to lack the GluR2 subunit.73
A second known post-transcriptional modification to AMPA receptors via RNA editing is the substitution of the genomically encoded arginine (R) for glycine (G) in GluR2, GluR3, and GluR4. This R/G site is located just before the flip/flop cassette in the extracellular domain between M3 and M4. Generally speaking, homomeric and heteromeric assemblies of edited (G) receptors have faster kinetics for recovery from desensitization (resensitization) than do their unedited (R) counterparts. Variations also occur in the kinetics of desensitization in edited (G) versus unedited (R) receptors, with a tendency toward slower desensitization rates. Akin to the other post-transcriptional splice variants and RNA-edited forms of AMPA receptors, the R/G edit progresses with brain development in a subunit and splice variant-dependent manner.42
Post-translational Modifications.
As another way to generate additional diversity, AMPA receptors also undergo post-translational modifications in the forms of glycosylation and phosphorylation. All AMPA receptors contain extracellular glycosylation sites but different subunit combinations appear to be affected differently by the presence or absence of bound oligosaccharides. For example, with the exception of GluR2, the lectin concanavalin-A potentiates AMPA receptor–mediated currents by binding to these carbohydrates and inhibiting desensitization.50 Although it is not entirely clear what the function of glycosylation is in these receptors, it is thought that this modification is involved in the maturation and transport of receptors and possibly the protection of AMPA receptors from degradation.116
Additionally, AMPA receptors have been shown to be phosphorylated basally or in response to varying types of synaptic activity. Although commonalities exist, the particular phosphorylated amino acid residues and kinases responsible for these modifications vary on a subunit basis. Phosphorylation of these residues leads to alterations in the receptor-gated channel properties. One of the most extensively studied instances of this phenomena is the phosphorylation of specific residues in the C-terminal domain of GluR1. GluR1 subunit phosphorylation potentiates receptor activation and leads to an increase in channel conductance and open probability,7,38,124 and these modifications have been shown to be involved in forms of plasticity such as long-term potentiation (LTP) and long-term depression (LTD).76 A complete description of this work is beyond the scope of this review, and the reader is directed to an excellent review.116
Structure and Function of AMPA Receptors.
The structure of the ligand-binding core of AMPA receptors, as well as other iGluRs, has been determined through the application of X-ray diffraction, and this has led to considerable insight into the molecular mechanisms of receptor activation and desensitization.56 By using a water-soluble construct of the li- gand binding domain (S1 and S2), the structure was determined to have a hinged clamshell shape, in which the agonist binds in the cleft between each shell. In the absence of agonist, the clamshell is mostly open, whereas in the presence of agonist, the clamshell closes to varying degrees depending on the particular agonist. The degree of closure was shown to correlate with the degree of receptor activation (in which kainate, a partial agonist for AMPA receptors, caused an intermediate degree of closure relative to the full agonists AMPA and glutamate). Furthermore, competitive antagonists like 6,7-dinitro-quinoxaline-2,3(1H,4H)-dione (DNQX) were shown to stabilize the open conformation of the ligand-binding clamshell. Finally, the observation that these receptor fragments tended to favor crystallizing as dimers led to hypotheses and subsequent studies examining the tetrameric stoichiometry of these receptors (a dimer of dimers), as well as the conformational changes that are necessary for the closing of the clamshell
binding pocket to translate to opening of the channel and desensitization of the receptor complex.
binding pocket to translate to opening of the channel and desensitization of the receptor complex.
Electrophysiology
As noted earlier, the ligand-binding pocket of iGluRs consists of a bilobed “hinged clamshell” structure that adopts differing conformations in the absence or presence of agonist. Upon binding of the full agonists glutamate or AMPA, the cleft between the two lobes (S1 and S2) closes by approximately 20 degrees relative to each other, whereas binding of the partial agonist kainate induces a cleft closure of approximately 12 degrees.56,86 In either event, this cleft closure is coupled to receptor activation and ion channel opening. In most natively expressed receptors, the ion channel is equally permeable to Na+ and potassium (K+), and the resulting current has a depolarizing effect on the neuron by driving the membrane potential toward an equilibrium potential of approximately 0 mV. However, in AMPA receptors that either lack the GluR2 subunit, or in which the GluR2 subunit is unedited, there is also a significant permeability to calcium (Ca2+).
Electrophysiologic studies using single-channel recordings of recombinant AMPA receptors have shown that homo- and heteromeric channel assemblies display multiple conductance levels whose amplitudes vary in a subunit-dependent manner, as well as in response to post-transcriptional and post-translational modifications.38,129,147 For example, homomeric receptors composed of the unedited GluR4(Q), as well as heteromeric receptors composed of unedited GluR4(Q) and GluR2(Q), have single-channel conductance between 20 and 30 picosiemens (pS). On the other hand, inclusion of edited GluR2(R) into these heteromeric assemblies reduces the conductance to approximately 10 pS, and homomeric assemblies of GluR2(R) have conductances in the femtosiemens (fS) range.147 Native AMPA receptors in a variety of neurons have single-channel conductance similar to those reported for recombinant receptors. For example, cultured cerebellar granule cells have AMPA receptors that fall into three categories based on their single-channel conductance: High–conductance (10–30 pS), low-conductance (5–10 pS), and femtosiemens channels (<1 pS).35,162
One of the most dramatic features of this receptor, relative to the NMDA receptor, is the rapid desensitization observed when the receptor is exposed to the full agonists AMPA or glutamate.151,154 After rapid application of an agonist, the channel opens. However, in the continued presence of the agonist, the amount of current flow is rapidly reduced, with a time constant of decay of <10 msec. If the agonist application is terminated and then reapplied, the current is again quite large, so that desensitization does not last long in the absence of agonist. Electrophysiologic studies have led to the conclusion that desensitization probably contributes, at least in part, to the rapid decay of excitatory postsynaptic currents (EPSCs) observed during neurotransmission at excitatory synapses.151,152
Pharmacology
AMPA receptors are responsible for most of the rapid excitatory neurotransmission within the vertebrate CNS. When compared to NMDA receptors, AMPA receptors have a relatively low affinity for glutamate, the endogenous amino acid that represents the most likely candidate in mediating neurotransmission at both these receptors.117 In addition to AMPA and glutamate, AMPA receptors can be activated following the binding of quisqualic acid, derivatives of willardiine, and natural toxins such as domoic acid. However, as mentioned earlier, the most potent selective agonist for this class of EAA receptor is AMPA67a. As noted earlier, binding of agonists to AMPA receptors often results in various degrees of desensitization, and the desensitization observed appears to be agonist-specific. Little to no desensitization occurs when a partial agonist, such as kainate, is used to induce a current. However, AMPA, quisqualic acid, glutamate, and many other full agonists result in a desensitizing current at this receptor.
Both competitive and noncompetitive antagonists of AMPA receptors have been described. Competitive antagonists of this receptor include 5-cyano-7-nitroquinoxaline-2,3-dione (CNQX) and other analogs of the quinoxalinedione family such as 1,2,3,4-tetrahydro-6-nitro-2,3-dioxo- benzo[f]quinoxaline-7-sulfona- mide (NBQX), DNQX, and 1,4,7,8,9,10-hexahydro-9-methyl-6-nitropyride[3,4-f]quinoxaline-2,3-dione (PNQX).60 This family of antagonists has limited utility in discriminating between AMPA and kainate receptors. Fortunately, noncompetitive antagonists that have a sufficiently higher affinity for AMPA than kainate receptors have been developed; 2,3-benzodiazapines, such as GYKI 52466 and 53655, are relatively selective for AMPA receptors and have allowed for the functional examination of kainate receptors (see below).69
Regulation of AMPA Receptor Subunit Expression and Function
Anatomic Distribution.
The anatomic distribution of AMPA receptors in the CNS has been determined by in situ hybridization, receptor autoradiography, and immunocyto-chemistry.69 These receptors are widely present in projection neurons and interneurons throughout the brain, although regional differences exist in the relative amounts of each of the receptor subunits. For example, whereas GluR1, GluR2, and GluR3 are rather ubiquitously expressed throughout the CNS, GluR4 expression is limited to select regions of the thalamus and cerebellum. In addition, whereas principal neurons utilize AMPA receptors including the GluR2 subunit, interneurons are more likely to express AMPA receptors lacking GluR2 and are thus permeable to Ca2+. AMPA receptors are thought to be located primarily postsynaptically, although some evidence suggests that presynaptic AMPA receptors are present in some neurons and can influence neurotransmitter release.
In addition to neurons, AMPA receptors are also found in glia, where they are thought to be able to sense nearby neuronal activity.156 With regards to epilepsy, the functional properties of these receptors may be modified by changes in the expression levels of individual subunits and/or their pre- and post-translational modifications. For example, it has been demonstrated that GluR1 receptors expressed in hippocampal astrocytes have an elevated flip-to-flop ratio in tissue from humans with pharmacoresistent TLE.135,136 This increase in the flip isoform could result in receptors with slower desensitization and enhanced depolarizations in astrocytes.
Developmental Regulation.
GluR1, GluR2, and GluR3 are all present and expressed at birth in the rat, whereas the GluR4 subunit is not expressed until approximately postnatal day 14. There is considerable developmental regulation of the splice variant forms of the AMPA receptor, and these changes over time can dramatically impact the function of the receptor and ultimately the excitability of the neuron.102 In general, in the adult animal, most AMPA receptors contain the flop form of the receptor subunits. This results in receptors that are generally impermeant to calcium and desensitize rapidly. However, in the young brain, a greater flip-to-flop ratio is present, which results in receptors that are more permeant to calcium and open for longer durations. This contributes greatly to the hyperexcitability observed in the young brain.
Trafficking and Associated Proteins.
Interestingly, it is thought that AMPA receptors are not initially present at excitatory synapses, although NMDA receptors are present at postsynaptic sites at birth. Furthermore, transcription as well
as insertion of AMPA receptors into postsynaptic sites is tightly regulated and, for some forms of the receptor, is activity dependent. Recent work has dramatically increased the understanding of AMPA receptor trafficking at synapses, and the reader is encouraged to see some excellent recent reviews on this extensive topic.111,116
as insertion of AMPA receptors into postsynaptic sites is tightly regulated and, for some forms of the receptor, is activity dependent. Recent work has dramatically increased the understanding of AMPA receptor trafficking at synapses, and the reader is encouraged to see some excellent recent reviews on this extensive topic.111,116
Briefly, AMPA receptors are assembled in the endoplasmic reticulum, transported through the Golgi, and targeted to the dendrites. The exact mechanisms whereby the receptors are targeted to dendrites and delivered there are currently under investigation. However, because AMPA receptors lack motor components, it is believed that associated proteins are involved in propelling the receptors along cytoskeletal tracks to the dendrites. For GluR2 and GluR3 receptor subunits, interactions with the C-terminus with glutamate receptor interacting protein/AMPA binding protein (GRIP1/ABP) is thought to be necessary for proper dendritic delivery, whereas the interaction of the C-termini of the GluR1 and GluR4 receptors with protein 4.1N seems to be essential for proper delivery of these subunits.16,49,111,138 Although cytoplasmic vesicles are responsible for initial delivery of AMPA receptors, it is unclear whether they deliver them to the postsynaptic or extrasynaptic sites. Indeed, a number of studies indicate that extrasynaptic receptors are free to diffuse to synaptic sites, where they are then anchored in place by a number of scaffolding proteins.
Once at the dendrite, GluR2- and GluR3-containing receptors are free to rapidly cycle between the postsynaptic sites and intracellular compartments constitutively in an activity-independent fashion. Thus, it is thought that these receptors help to maintain a constant level of receptors at the synapse. In contrast, AMPA receptors containing GluR1 and GluR4 subunits are tightly regulated at the synapse in an NMDA- and activity-dependent fashion. It is believed that this activity-dependent regulation of receptor number at the synapse results in both LTP and LTD (see Chapter 35).
Critical to the ability of AMPA receptors to be inserted into the postsynaptic membrane is the presence of the transmembrane AMPA receptor regulatory proteins, or TARPs. These auxiliary proteins have been found to be important for the selective delivery of AMPA receptors to extrasynaptic regions. In addition, TARPs have now been shown to regulate the activity of AMPA receptors. For example, the first TARP to be examined, stargazin, has been shown to increase the affinity for glutamate at the AMPA receptor and also decrease desensitization and deactivation rates.121,134,153 An interesting aside to this story is that the stargazin gene has been implicated in absence seizures observed in the stargazer mouse, although the seizures appear to be linked to the proteins’ interaction with voltage-gated calcium channels in the thalamus.82,83
Different AMPA receptor subunits combine with different combinations of auxiliary proteins that can oversee a myriad of functions of AMPA receptors. In general, the associated proteins fall into one of two categories: PDZ domain–containing proteins and non-PDZ domain–containing proteins. A thorough description of all associated proteins is well beyond the scope of this chapter, and the reader is referred to several recent reviews.116 However, as we learn more about the assembly, targeting, and regulation of function of AMPA receptors, a number of potential therapeutic targets for the treatment of a variety of seizure disorders will no doubt emerge.
AMPA Receptors and Seizures
Clearly, excitatory synaptic transmission is involved in many aspects of synchronization and seizure generation, and there is no doubt that AMPA receptor number and function are critically involved in these mechanisms. Not only are there alterations in excitatory circuits in some forms of epilepsy (most notably mossy fiber sprouting in TLE), but a number of studies have demonstrated that following seizures, changes occur in a number of cell types with regards to the type of AMPA receptor subunits that are expressed. Changes in subunit expression have been observed in both animal models of epilepsy as well as in human epilepsy.37,43,57,61,90,122,131,143 Clearly, these alterations in subunit expression have important ramifications with respect to the function and regulation of excitatory synaptic transmission, and these alterations are just beginning to be understood at the circuit level. Many of these changes in excitatory synaptic transmission are addressed in a number of other chapters in this book and will not be elaborated upon here.
Kainate Receptors
Molecular Biology
Cloning studies have revealed the existence of five kainate receptor (KAR) subunits: The low-affinity subunits GluR5, -6, and -7, and the high-affinity subunits KA1 and KA2. (This topic has been reviewed.)42,80 Multiple alternative splice variants have been identified for GluR5 through GluR7. Additionally, post-transcriptional editing occurs in GluR5 and GluR6 at the glutamine/arginine (Q/R) site, but this does not occur in the GluR7, KA1, or KA2 subunits. GluR5 through GluR7 can form functional homomeric KARs in expression systems, whereas the KA1 andKA2 subunits cannot. However, KA1 and KA2 subunits can assemble with GluR5, -6, and -7 to form functional heteromeric receptors. Clearly, this inherent molecular diversity can lead to the assembly of a sizeable number of different KARs with distinct stoichiometries. As was the case for AMPA receptors, subunit assembly can dictate the functional properties of the resulting receptor-gated ion channels, including permeability, conductance, and pharmacology. For example, homomeric assemblies of GluR5 or GluR6 that possess an arginine at the Q/R site are functionally different from receptors composed of subunits possessing a glutamine.10 These edited, arginine-containing receptors have a reduced calcium permeability, a linear or slightly outward rectifying current-voltage relationship instead of an inward or double rectifying relationship, a single low conductance state as compared with multiple conductance states, and an increased chloride permeability.
Electrophysiology
Postsynaptic Kainate Receptors.
Functional postsynaptic KARs are present in a variety of cell types. Examples include cerebellar granule cells and Golgi cells18,140; retinal bipolar cells40; neurons of the superficial dorsal horn,84 lateral superior olive,158 and motor and somatosensory cortex2,45,71; and a variety of neurons in the hippocampus and amygdala.22,31,32,52,53,54,58,127,157,160 As originally described in CA3 neurons of the hippocampus, KAR-mediated EPSCs are defined as being resistant to inhibition by the AMPA receptor-selective 2,3-benzodiazepine GYKI 53655 (or GYKI 52466), but sensitive to inhibition by CNQX.22 Furthermore, KAR-mediated EPSCs are smaller than their AMPA receptor-mediated counterparts, have significantly slower rise and decay kinetics, and are not potentiated by cyclothiazide.22,157 Even though the KAR contribution to combined AMPA/kainate-mediated EPSCs is relatively small, postsynaptic KARs participate in synaptic transmission and may even be segregated to their own synapses.31 Data using glutamate uptake blockers suggests that the KARs’ slow kinetics are due to intrinsic characteristics18,71 and may impose unique integrative properties to neurons.53
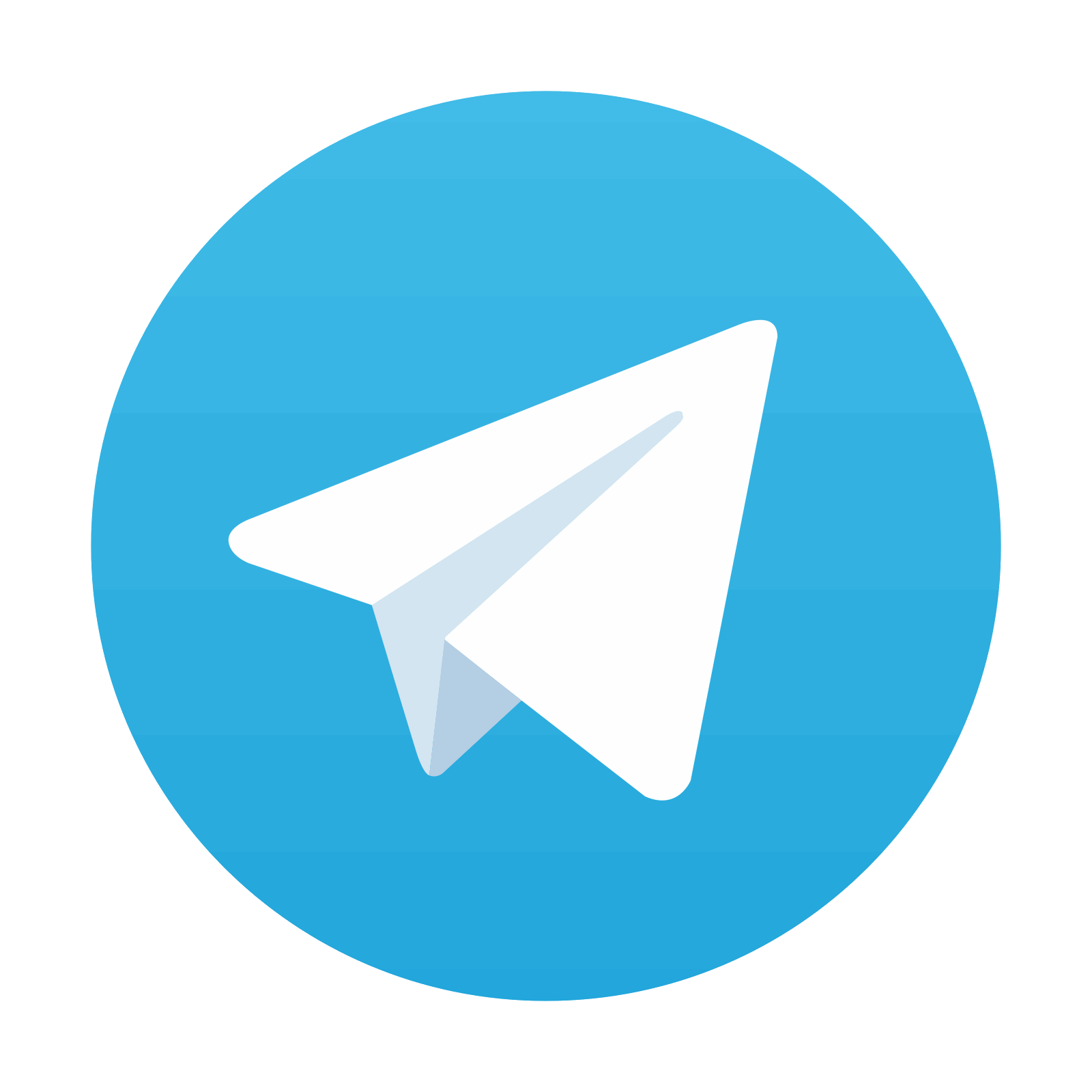
Stay updated, free articles. Join our Telegram channel

Full access? Get Clinical Tree
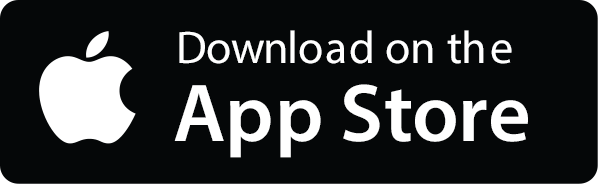
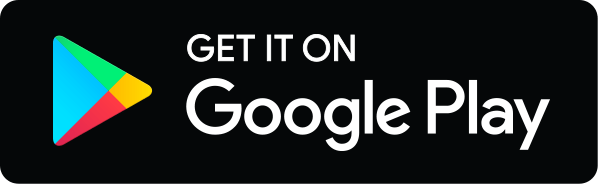
