html xmlns=”http://www.w3.org/1999/xhtml” xmlns:mml=”http://www.w3.org/1998/Math/MathML” xmlns:epub=”http://www.idpf.org/2007/ops”>
Introduction
The possibility that exercise could be used as a treatment in mental illness has long been recognized. For example, exercise was considered a popular and effective treatment for alcoholism in the nineteenth century with benefits for cognitive functioning. As Cowles (1898: 108) described:
The benefits accruing to the patients from the well-directed use of exercise and baths is indicated by the following observed symptoms: increase in weight, greater firmness of muscles, better colour of skin, larger lung capacity, more regular and stronger action of the heart, clearer action of the mind, brighter and more expressive eye, improved carriage, quicker responses of nerves, and through them of muscle and limb to stimuli. All this has become so evident to them that only a very few are unwilling to attend the classes and many speak freely of the great benefits derived.
Over a hundred years later there is now a sufficiently robust evidence base to confirm the existence of a strong relationship between physical activity and a number of dimensions of mental health. In terms of using exercise to treat mental illness the most convincing evidence is in the area of clinical depression. The objectives of this chapter are to synthesize recent evidence concerning the use of exercise as a treatment for depression before providing an overview of the effects of exercise on cognitive functioning more generally. Potential mechanisms explaining the benefits of exercise on cognitive functioning will then be reviewed before presenting existing evidence concerning exercise and cognitive functioning for individuals with depression.
What is exercise?
Physical activity is a general term that refers to any movement of the body that results in energy expenditure above that of resting level (Caspersen, Powell, & Christenson, 1985). Exercise is often (incorrectly) used interchangeably with physical activity, but this term refers to a subset of physical activity in which the activity is structured, often supervised, and undertaken with the aim of maintaining or improving physical fitness or health. Examples of exercise include “going to the gym,” jogging, taking an aerobics class, or taking part in recreational sport for fitness. A focus of much research into mental health has considered the effects of well-controlled exercise sessions on mental health outcomes. A more recent focus has been on habitual physical activity – for example, encouraging individuals to increase the number of steps they take throughout the day. Researchers are also interested in both the acute and the chronic effects of exercise and physical activity. In general, exercise prescriptions consider the elements of frequency, intensity, time (or duration), and type of exercise.
The World Health Organization (2010) has issued global recommendations on physical activity for health. These include the recommendation that children aged 5 to 17 years accumulate 60 minutes of moderate to vigorous intensity activity on a daily basis. For adults, the guidance suggests that a minimum of 150 minutes of moderate intensity activity should be accumulated over the course of the week in bouts of no less than ten minutes. Adults are also encouraged to take part in activities that promote muscle strengthening at least twice per week. These guidelines are consistent with most national guidelines for physical activity.
Why consider exercise in the context of depression?
There are a number of attributes that might make physical activity an attractive treatment option if found to be efficacious (Faulkner, Trinh, & Arbour-Nicitopoulos, 2015b). First, it could be a cost-effective alternative for those preferring not to use medication or who cannot access therapy. It can be self-sustaining in that it can be potentially maintained, unlike pharmacological and psychotherapeutic treatments, which usually have a specified endpoint. Second, physical activity is associated with negligible deleterious side-effects. Third, physical activity is an effective method for improving important aspects of physical health (see Lee et al., 2012). The effect of physical inactivity on major non-communicable diseases worldwide is similar to the established risk factors of smoking and obesity. The promotion of physical activity for mental health can be seen as a “win-win” situation with both mental and physical health benefits accruing (Faulkner, Hefferon, & Mutrie, 2015a). Fourth, given the scope of the burden of poor mental health, there is the need to develop population-based promotion, prevention, and treatment strategies. Potentially, physical activity is a scalable, population-level intervention for promoting mental health, unlike pharmacotherapy and counseling (Faulkner et al., 2015b).
Does exercise reduce depression?
The most recent review on the topic of exercise as a treatment for depression was conducted by Cooney et al. (2013), who identified 39 randomized controlled trials (RCTs) that met their inclusion criteria. The meta-analysis of these RCTs showed a moderate effect size (−0.62 (95% confidence interval (CI) −0.81 to −0.42)), for exercise versus no treatment control conditions. For the six trials considered to be at low risk of bias (adequate allocation concealment, intention-to-treat analyses, and blinded outcome assessment), a further analysis showed a small clinical effect in favor of exercise which did not reach statistical significance. Finally, the authors compared the exercise effects to those of cognitive behavioral therapy for the seven trials that had these comparisons and found no significant difference. Similarly, four trials (Blumenthal et al., 1999, 2007, 2012; Brenes et al., 2007) compared exercise with antidepressant medication and no significant difference was found.
The first pioneering study was known as the SMILE study (Standard Medical Interventions and Longterm Exercise). The effects of aerobic exercise were compared to sertraline (Zoloft) treatment among 156 older adults with depression (Blumenthal et al., 1999). Participants were randomized to either aerobic exercise (three times per week at 70–85 percent of their heart rate reserve), sertraline, or a combined sertraline and exercise group for 16 weeks. After 16 weeks of treatment, patients in all three groups exhibited significant reductions in depressive symptoms. However, patients responded more quickly in the medication group. After ten months, remitted (those who no longer met diagnostic criteria for depression) participants in the exercise group had significantly lower relapse rates than those in the medication group (Babyak et al., 2000).
Cooney and colleagues (2013) concluded that exercise appears to be no more effective than psychological or pharmacological therapies. A more appropriate interpretation is that exercise may be as beneficial as other common treatments – not necessarily better (Faulkner et al., 2015a). Compared with earlier meta-analyses examining exercise and depression, reduced effect sizes are now being reported because exercise is being compared with other active treatments such as psychotherapy and medication. However, exercise need not be considered a mutually exclusive alternative to other treatment modalities; rather exercise could be considered an additional treatment option based on patient need and preference.
There are many unanswered questions such as the exact dosage and mode of activity that might work best. One reason for this is the variability in the dosage that researchers examine. A recent review (Stanton & Reaburn, 2014) examined the dose characteristics of five RCTs reporting a significant treatment effect of exercise in the treatment of depression. They concluded that the exercise dose should likely use supervised aerobic exercise, and occur three times weekly at moderate intensity for a minimum of nine weeks in the treatment of depression. The positive effects of exercise on cognitive performance might also be an added benefit of exercise prescription and such benefits have received much attention both in healthy populations and those with cognitive deficits such as dementia. Drawing on relevant meta-analyses, the next sections will provide an overview of evidence focusing on the effects of acute and then chronic exercise on cognitive functioning.
Acute bouts of physical activity and cognition
An examination of five current meta-analyses demonstrates that an acute bout of PA improves cognitive function to some extent in the following domains among healthy individuals: speed of mental processing, memory, executive function, and attention. McMorris and Hale (2012) evaluated how varying intensities of an acute bout of PA would affect speed and accuracy on a variety of cognitive functions using 53 primary studies, all of which used a repeated measures, within-subjects design. Only the timing of the cognitive test (during versus post PA) had a significant effect on accuracy. A significant effect size was found when testing was done post-PA (Hedges’ g = 0.17, p < 0.05), compared with a non-significant effect during PA (McMorris & Hale, 2012). However, acute moderate intensity PA had moderate effects on speed of processing both during (g = 0.48, p < 0.001) and after (g = 0.53, p < 0.001) the PA bout, compared to non-significant effects found for low and high intensity PA. Furthermore, when task complexity was accounted for as a moderator, central executive tasks had a larger effect size (g = 0.77, p < 0.001) than recall and alertness/attention (g = 0.31, p = 0.01).
McMorris and colleagues conducted a meta-analysis of 24 studies that used a repeated measures, within-subject design to specifically examine the effects of an acute moderate bout of PA on both the speed and accuracy of working memory. They reported significant reduction in response time (g = −1.41, p < 0.001) but a detrimental effect on accuracy (g = 0.40, p < 0.01) (McMorris, Sproule, Turner, & Hale, 2011). When moderator analysis was undertaken, such as timing of the cognitive test (during vs. after), duration of exercise, fitness level, gender, and age, no differences were found. All studies included in this meta-analysis used cycling as the mode of PA and the cognitive tests relied heavily on central executive functions.
Roig and colleagues assessed the effects of both acute and chronic PA on memory, including 50 studies in the final analysis with the following study designs: RCTs, clinical (non-randomized) controlled trials, and within-subject designs. They found that an acute bout of PA had a moderate effect (standardized mean difference (SMD) = 0.26, p = 0.03), while chronic PA had a small effect (SMD = 0.15, p = 0.02) on short-term memory (Roig, Nordbrandt, Geertsen, & Nielsen, 2013). They also reported that acute PA had moderate-to-large effect (SMD = 0.52, p < 0.0001) on long-term memory, whereas chronic PA had insignificant effects (SMD = 0.07, p = 0.51) on long-term memory. When moderator analysis was undertaken between acute PA and memory, subtypes of memory and select PA dimensions showed greater improvements. Specifically, acute PA improved visuo-spatial short-term memory (SMD = 0.30, p = 0.005) as well as verbal-auditory (SMD = 0.54, p = 0.001) and procedural (SMD = 0.40, p = 0.05) long-term memory. Short acute bouts of PA (< 20 minutes) (SMD = 0.63, p = 0.01) at a low intensity (< 40% heart rate reserve) (SMD = 0.57, p = 0.01) were reported to be beneficial for short-term memory, whereas short (SMD = 0.53, p = 0.01) or medium (20–40 minutes) (SMD = 0.88, p = 0.01) duration bouts of any intensity had larger effects on long-term memory. Moderator analyses of the effects of chronic PA on short-term memory revealed the following PA characteristics as optimal: walking or running as a mode of PA (SMD = 0.34, p = 0.0005) of medium duration (20–40 minutes) (SMD = 0.47, p = 0.008) at light-moderate intensity (SMD = 0.55,p = 0.005). Furthermore, PA programs of long duration (SMD = 0.19, p = 0.009) were also beneficial. Only verbal-auditory memory (SMD = 0.16, p = 0.02) showed significant improvements when subtype of memory was examined. However, only procedural memory (SMD = 1.73, p = 0.03) was a significant moderator between the effects of chronic PA on long-term memory. In sum, both acute and regular PA appear to be conferring improvements in memory, potentially in different but complementary ways.
Two additional reviews examined how performance on cognitive tests varied according to the nature of the PA design and the cognitive function evaluated. With 50 repeated-measures studies, Lambourne and Tomporowski (2010) found that if the cognitive test is administered during the first 20 minutes of PA, there was a detrimental effect (g = −0.14, p = 0.04). However, there was a small improvement after the bout of PA (g = 0.20, p < 0.01), specifically in the areas of speed of mental processing, memory storage and retrieval. However, Chang and colleagues found that an acute bout of PA has an overall small, significant effect on cognition regardless of when (i.e. during, immediately following, or after a delay) the cognitive test is administered (g = 0.10, p < 0.05). They included 79 studies (1,034 effect sizes), where there were 755 within-subject comparisons and 279 between-subject comparisons. Specifically, they found that cognitive tasks that evaluate executive function (g = 0.19, p < 0.05) and attention (g = 0.42, p < 0.05) immediately after an acute bout of PA had a significant positive effect size, whereas only executive function (g = 0.17, p < 0.05) showed a positive effect size when it was assessed after a delay (> 1 minute post-PA). Furthermore, if cognitive function is assessed immediately after an acute bout of PA, very light (g = 0.15, p < 0.05), light (g = 0.17, p < 0.05), and moderate (g = 0.12, p < 0.05) intensity PA were beneficial. However, when the cognitive test was administered after a delay, moderate (g = 0.20, p < 0.05), hard (g = 0.27, p < 0.05), and very hard(g = 0.47, p < 0.05) intensities were seen as beneficial (Chang, Labban, Gapin, & Etnier, 2012). Those who were considered low (g = 0.17, p < 0.05) or high (g = 0.22, p < 0.05) fitness showed greater improvements if tested immediately after the PA and those who were of moderate (g = 0.20, p < 0.05) or high (g = 0.33, p < 0.05) fitness showed better improvements on tests after a delay post-PA.
The effects of an acute bout of PA are often short lived. Chang and colleagues (2012) reported that only studies that evaluated cognitive function between 1 and 15 minutes after the bout of PA found a small positive effect (g = 0.14, p < 0.05), but when tested post-15 minutes, there was no significant effect. However, the time course of cognitive function post-PA is a relatively unexplored area with regard to the physiological mechanisms that may be mediating the effects. It is possible that the duration of cognitive benefits post-PA may be dependent on the intensity and duration of the bout of PA (Tomporowski, 2003). For example, a common physiological response to an acute bout of PA is the increased plasma levels of epinephrine and norepinephrine, which has been associated with cognitive function (Chmura, Nazar, & Kaciuba-Uścilko, 1994). The rate of production of these substances and their duration in the bloodstream differs based on the nature of the acute PA paradigm (Hughson, Green, & Sharratt, 1995; Rowell & Shepherd, 1996). Second, personal factors, such as physical fitness, past experience, age, and gender may be playing a role as well.
In sum, an acute bout of PA appears to have a small, positive effect on cognitive function among healthy individuals, in particular speed of mental processing, memory, executive function, and attention. Because these individuals are already at an average level of cognitive function at the time of the experiments, it is possible that a ceiling effect is operating. It is possible that individuals with cognitive impairment, due to their reduced baseline functioning, may see greater improvements. The methodological constraints within each primary study and the heterogeneity between these studies that are included in the meta-analyses may also explain the small effect sizes reported. Moderator variables also play an important role in determining the level of improvements seen. Therefore, future research should continue to explore a range of factors that may moderate the relationship between acute PA and changes in cognitive functioning.
Chronic exercise interventions and cognitive functioning
Regular PA has also been shown to confer cognitive benefits. Smith and colleagues (Smith et al., 2010) conducted a meta-analysis with 29 RCTs, comparing an aerobic exercise intervention (> 1 month) with a non-aerobic-exercise condition. They found that individuals assigned to receive the aerobic exercise intervention showed modest improvements in attention and processing speed (g = 0.158, p = 0.003), executive function (g = 0.123, p = 0.018), and declarative memory (memory), which includes retention, recollection, and recognition of previously encountered information (g = 0.128, p = 0.026) compared with the non-aerobic-exercise control condition. Working memory, defined as memory involved in short-term storage and manipulation of information, however, was not seen to improve significantly (g = 0.032, p = 0.642). Moderator analyses were conducted to examine the effect of the mode, duration, and intensity of the exercise intervention as well as baseline participant variables, such as cognitive functioning and age. For attention and processing speed, a combined intervention (aerobic exercise + another type of activity) showed greater improvements (g = 0.250, p = 0.026) than interventions that used aerobic exercises only (g = 0.098, p = 0.152). Improvements in executive function were not significant among individuals with mild cognitive impairment (MCI) (g = −0.004, p = 0.973) compared with those without cognitive impairment (g = 0.153, p = 0.008). Improvements in working memory were seen in combined (e.g. aerobic plus strength training) interventions (g = 0.288, p = 0.028) compared with interventions with aerobic exercise only (g = −0.042, p = 0.567). Furthermore, older age was correlated with better working memory (r = 0.564, p = 0.051). Participants with MCI saw greater improvements in memory (g = 0.237, p = 0.050) compared with participants without cognitive impairment (g = 0.096, p = 0.143). The authors concluded that aerobic exercise provides modest improvements in cognitive function, particularly in the areas of attention and processing speed, executive function, and memory, with preliminary evidence pointing to greater improvements in memory among MCI individuals compared with cognitively normal individuals.
Decline in cognitive function is commonly attributed to increasing age. Three recent meta-analyses reported that regular PA among healthy older adults is protective against cognitive decline. Through the inclusion of 15 prospective studies, Sofi and colleagues (Sofi et al., 2011)found that older adults who reported low-to-moderate levels of PA were significantly protected against cognitive decline (hazard ratio (HR) = 0.62, p < 0.01) at follow-up than those who were not active. Morgan and colleagues (Morgan et al., 2012) included 21 prospective studies in their final analysis. They found an overall protective effect of physical activity against cognitive impairment (odds ratio (OR) = 0.66, 95% CI: 0.52–0.85, p < 0.01) and dementia (OR = 0.78, 95% CI: 0.65–0.94, p < 0.01). These findings were supported by a recent meta-analysis by Blondell, Hammersley-Mather, and Veerman (2014) who also found that individuals who engage in higher levels of PA are at a reduced risk of cognitive decline (relative risk (RR) = 0.65, 95% CI: 0.55–0.76, p < 0.01) and dementia (RR = 0.86, 95% CI: 0.76–0.97, p < 0.01) compared with those who engaged in low levels of PA. This meta-analysis of prospective studies included 21 cohorts that examined the relationship between PA and cognitive decline and 26 cohorts that examined the relationship between PA and dementia. Therefore, evidence consistently points toward a protective effect of regular PA against cognitive decline and dementia later in life.
In addition to having a protective effect, three meta-analyses found regular PA to improve cognitive function in older adults without any known cognitive impairments. Angevaren and colleagues included 11 RCTs that compared an aerobic PA program with any other intervention or no intervention among adults 55 years of age or older. They found that PA interventions had a large effect on motor function (weighted mean difference (WMD) = 1.17, p = 0.02) and auditory attention (WMD = 0.52, p < 0.01). They also found moderate effects for information processing speed (SMD = 0.26, p = 0.02) and visual attention (SMD = 0.26, p = 0.03) (Angevaren, Aufdemkampe, Verhaar, Aleman, & Vanhees, 2008). Eight of the 11 studies found that participants in the aerobic PA intervention saw an improvement in cardiorespiratory fitness of 14 percent, which was related to improvements in cognitive function. Although this meta-analysis is suggestive of a causal link between aerobic PA, cardiorespiratory fitness, and better cognitive function, the authors warn that larger and longer studies are necessary to confirm this effect and to determine the optimal PA intervention dimensions for cognitive improvement.
Kelly and colleagues (Kelly et al., 2014) conducted a meta-analysis evaluating the effects of various types of exercises (aerobic exercise, resistance training, and Tai Chi) on cognitive function in healthy older adults without any known cognitive impairment. Twenty-five RCTs met the inclusion criteria and the control group consisted of a stretching/toning control group, a “no exercise” active control group, or a “no intervention” control group. Significant improvements were found in the resistance training group compared with the stretching/toning group on measures of reasoning (SMD = 0.10, p < 0.005) and when Tai Chi was compared with “no exercise” active controls on measures of attention (SMD = −1.19, p < 0.001) and processing speed (mean difference (MD) = 11.05, p < 0.001). However, no significant differences were found between the aerobic exercise and controls on any of the 26 measures of cognitive function. The authors recommend caution in interpreting the results of this study due to the heterogeneity within the included studies, as there was variation in participant demographics, type of exercise program, and participant adherence to the interventions.
Colcombe and Kramer (2003) conducted a meta-analysis to determine whether physical activity improves cognitive function in healthy but sedentary older adults (55–80 years of age). They included 18 randomized, longitudinal intervention studies in their final analysis and found a consistent positive effect favoring some cognitive functions, in particular executive-control processes, which include coordination, inhibition, scheduling, planning, and working memory. They found that participants in the exercise intervention group had the largest effect for executive control processes (g = 0.68, SE = 0.052, p < 0.05) compared to participants in the control group. There were significant improvements compared with controls in “controlled-processing,” which refers to tasks that originally require controlled, effortful processing but become automatic with practice (g = 0.461, SE = 0.035, p < 0.05), speed (g = 0.274, SE = 0.050, p < 0.05), and visuo-spatial domains (g = 0.426, SE = 0.062, p < 0.05). Interventions that included both strength and aerobic training found greater improvements (g = 0.59, SE = 0.049, p < 0.05) than aerobic training alone (g = 0.41, SE = 0.037, p < 0.05). Exercise programs that lasted more than six months (g = 0.674, SE = 0.048, p < 0.05) saw greater improvements than programs that lasted one to three months (g = 0.522, SE = 0.067, p < 0.05) or four to six months (g = 0.269, SE = 0.047, p < 0.05). Only sessions that lasted more than 30 minutes were found to have significant improvements (31–45 minutes: g = 0.614, SE = 0.052, p < 0.05; 46–60 minutes: g = 0.466, SE = 0.041, p < 0.05). Participants between the ages of 66 and 70 showed the greatest improvements (g = 0.693, SE = 0.056, p < 0.05) compared with participants aged 55–65 years (g = 0.298, SE = 0.044, p < 0.05) and 87–80 years (g = 0.549, SE = 0.058, p < 0.05). In sum, these three meta-analyses illustrate that regular PA does improve cognitive function among older adults, although there is variation about the specific areas that are improved and uncertainty about the optimal PA design that would confer these benefits.
There is also some preliminary evidence to suggest that regular PA may support those who are already affected by cognitive impairment. Two meta-analyses evaluated the effect of PA for cognitive improvement among older adults with cognitive impairment or dementia. Heyn, Abreu, and Ottenbacher (2004) evaluated 30 RCTs and found that those with cognitive impairment who engaged in PA had a moderate improvement in cognitive functioning (ES = 0.57; 95% CI: 0.38–0.75, p < 0.01) compared with control and/or comparison groups. On average, the physical activity program lasted 23 weeks, ranging from 2 to 112 weeks for an average of 3.6 sessions per week (range: 1–6 sessions). Each session lasted 45 minutes on average (20–150 minutes). Most studies used walking as the mode of PA or a combination of walking and isotonic exercises.
Finally, Gates, Fiatarone Singh, Sachdev, and Valenzuela (2013) conducted a meta-analysisevaluating the effect of exercise on cognitive function among older adults with MCI. They included 14 RCTs in their analysis and found only a small improvement in verbal fluency (ES = 0.17, 95% CI: 0.04–0.30, p < 0.05). Of the four trials that evaluated global cognition through aerobic exercise, three found medium-to-large significant improvements. Although aerobic exercise did not show improvements in memory, two studies that evaluated resistance training found large and moderate improvements.
In sum, there is evidence for both a protective and cognitive enhancing role for regular PA. Chronic PA appears to have consistent but selective benefits for cognition with the largest benefits occurring for executive control processes. There is also a general trend seen where individuals who are active throughout their life have the greatest reduced risk of developing cognitive impairment and dementia later in life. Furthermore, improvements in cognitive function have also been seen among individuals affected by cognitive impairment. Therefore, although regular PA throughout life is ideal as it produces the greatest protective effects against cognitive decline, it appears that it is never too late to start to become physically active to protect and enhance cognitive function.
How might exercise impact cognitive functioning?
Current theories on the cognitive improvements following an acute bout of exercise focus on neuroendocrinological responses to exercise (McMorris, 2009), where it is hypothesized that acclimations in resting-state physiology and neuroanatomical structure likely drive cognitive benefits in response to chronic physical activity participation (Berchtold & Cotman, 2013; Cotman, Berchtold, & Christie, 2007). We will briefly discuss some physiological mechanisms theorized to be involved in promoting improved cognition in response both to acute and chronic bouts of physical activity, and their implications for individuals with depression.
Mechanisms: acute effects
McMorris (2009) provides an extensive review of potential neuroendocrine mechanisms explaining acute exercise effects on cognition. We have summarized what we believe to be some of the important neurotransmitter candidates, specifically the catecholamines: norepinephrine and dopamine, as well as serotonin. In a seminal review of the biological mechanisms linking exercise and cognition, Cooper (1973) originally hypothesized that increased tonic activation of key neural pathways through catecholamines drives improved cognitive capacities in response to exercise. As a result, a great deal of attention has been paid to the role of dopamine and norepinephrine in cognitive responses to acute bouts of exercise.
In terms of cognition, norepinephrine and dopamine are important to learning, memory, executive function, and attention (Bäckman, Nyberg, Lindenberger, Li, & Farde, 2006; Chamberlain & Robbins, 2013). However, it is difficult to determine the release patterns of norepinephrine and dopamine in the human brain during exercise. Exercise causes the release of catecholamines peripherally. However, catecholamines cannot readily cross the blood–brain barrier. Thus peripheral measurements of catecholamines or their major metabolites are not necessarily definitive of what is occurring in the brain. In animals, central catecholamine release is dependent on exercise intensity (Hattori, Naoi, & Nishino, 1994). Animal studies demonstrate a nuanced and frequently regionally specific catecholamine response to acute bouts of exercise (for a comprehensive description of studies see Meeusen & De Meirleir, 1995 and Meeusen, Piacentini, & De Meirleir, 2001). For example, whole brain norepinephrine concentrations appear to consistently decrease, or remain unchanged, in response to an acute bout of exercise, but increase in the striatum (Meeusen & De Meirleir, 1995; Meeusen et al., 2001). A similar pattern is observed for dopamine concentration in animal brains, where concentrations of dopamine tend to increase in the striatum, but whole brain dopamine concentrations remain stable (Meeusen & De Meirleir, 1995; Meeusen et al., 2001). These patterns are inconsistent with Cooper’s (1973) original hypothesis that more catecholamine availability leads to improved cognition. However, exercise protocols vary greatly between studies, and may in part explain inconsistent findings.
Ultimately, McMorris (2009) concludes that there is minimal support to suggest that changes in catecholamines in response to exercise influence cognition. Given the myriad connections in the brain and the varying receptor subtypes, each with different post-synaptic effect, it seems highly unlikely that changes in brain catecholamine concentration are a sufficient explanation for the acute cognitive benefits of exercise. Rather, it is more likely that the catecholamine systems interact with glutaminergic, GABAminergic, and other neurons to modify brain region functioning and increase arousal in relevant brain regions. Thus a more nuanced approach is likely necessary to understand neurotransmitter influence on cognition in response to exercise.
Serotonin also plays an important role in memory, learning, and executive function (Cowen & Sherwood, 2013). Thus it is unsurprising that serotonergic neurons project into the hippocampus and frontal cortex. Exercise increases the amount of unbound tryptophan – the amino acid precursor of serotonin – in the blood, which readily crosses the blood–brain barrier (Chaouloff, Kennett, Serrurrier, Merino, & Curzon, 1986; McMorris, 2009). Tryptophan is then converted into serotonin in the brain. In animals, an acute bout of exercise is consistently shown to increase both hippocampal (Meeusen et al., 1996; Wilson & Marsden, 1996) and whole brain serotonin concentration (see Meeusen & De Meirleir, 1995). This evidence has been mirrored in studies of humans, where an increase in free tryptophan in the blood is used as a marker of serotonin production in the brain (Melancon, Lorrain, & Dionne, 2012; Nybo, Nielsen, Blomstrand, Møller, & Secher, 2003). Despite the seemingly straightforward theoretical connection, we did not identify any studies that attempted to connect exercise-induced changes in serotonin production (or a suitable proxy indicator) as a mediator for changes in cognitive outcomes.
Serotonin may play a particularly important role in cognition and depression. Cowen and Sherwood (2013) suggest that different thresholds exist for the beneficial effects serotonin has on depressive and cognitive symptoms, as even those patients whose depressive symptoms are in remission may continue to have cognitive impairments. Exercise could prove to be an effective adjunct for safely increasing serotonin level in conjunction with antidepressant use to help alleviate cognitive deficits. Furthermore, serotonin appears to provide a link between transient cognitive improvements with an acute bout of exercise and neurogenesis effects of exercise attributed to long-term cognitive benefits associated with chronic physical activity. Specifically, mice that lack serotonin in the brain do not exhibit exercise-associated hippocampal neurogenesis (Klempin et al., 2013).
Mechanisms: chronic effects
Several anatomical and functional changes in the brain associated with improved cognitive function are also associated with long-term physical activity participation. In humans, imaging studies demonstrate increased connectivity between the hippocampus, prefrontal cortex, and anterior cingulate gyrus with greater physical activity participation (Berchtold & Cotman, 2013; Burdette et al., 2010). Greater connectivity between these regions, which are responsible for memory and executive function, may indicate more effective communication between these regions (Berchtold & Cotman, 2013). As well, some chronic exercise interventions have found increased hippocampal volume in people with schizophrenia (Pajonk et al., 2010) and older adults (Erickson et al., 2011); and these changes in hippocampal volume are associated with improvements in memory. This evidence is especially relevant to the treatment of cognitive impairment for people with depression, as memory impairment is a common deficit, and people with depression tend to show decreased hippocampal volumes (Arnone, McIntosh, Ebmeier, Munafò, & Anderson, 2012) – even after the symptoms of depression have entered into remission (Neumeister et al., 2006).
These neuroplastic changes in the brain appear to be driven by the release of various growth factors in response to exercise. Some of the most important molecular antecedents of structural and functional change in the brain are brain-derived neurotrophic factor (BDNF), insulin-like growth factor 1 (IGF-1), and vascular endothelial growth factor (VEGF). Cotman and colleagues (Cotman et al., 2007) extensively review the individual function and contribution of each of these molecules in the context of exercise and cognition. In animals, these molecules are released in response to exercise and contribute to increases in neurogenesis, synaptogenesis, and long-term potentiation. BDNF in particular plays a number of roles in the brain, such as inducing long-term potentiation (neural indicator of learning), and neurogenesis, and is present in the hippocampus. As well, blocking BDNF and IGF-1 blocks the benefits exercise has on learning (Berchtold & Cotman, 2013). Furthermore, IGF-1 and VEGF induce angiogenesis in the hippocampus and cortex (Ding et al., 2006; Lopez-Lopez, LeRoith, & Torres-Aleman, 2004), which may further contribute to long-term cognitive benefits for memory and executive function by improving nutrient and oxygen supply to these regions. Together, BDNF, IGF-1, and BDNF molecules work in unison to affect structural and functional changes in the brain. However, these modifications take time, and are therefore more likely to contribute to long-term cognitive benefits accrued through consistent, regular physical activity over the long term in contrast to performing a single bout of PA.
Brain structure can also be affected by social and environmental interactions. Studies in animals demonstrate that environmental enrichment (more stimulating environments which include combinations of the presence of other animals, running wheels, and objects to manipulate) can increase neuroplasticity in much the same way exercise does (i.e. synaptogenesis (Briones, Klintsova, & Greenough, 2004); neurogenesis (Fan, Liu, Weinstein, Fike, & Liu, 2007; Van Praag, Kempermann, & Gage, 1999)), and can reverse hippocampal neuronal loss and memory impairment in chronically stressed rats (Veena et al., 2009). Furthermore, one study showed that running mice with minimal social interactions had greater proliferation of neural progenitor cells in the dentate gyrus at 12 days than a comparison group in an environment enrichment condition (Van Praag et al., 1999). However, four weeks later the mice in both groups showed no significant difference in the number of neural progenitor cell offspring that survived. Although the mice in the environmental enrichment group generated fewer neural progenitor cells, the survival percentage of these cells was significantly higher. Together, this suggests that while exercise and environmental enrichment are both capable of inducing neuroplasticity, and therefore potentially modifying cognitive abilities, the effects may be additive whereby exercise is better able to generate new neural progenitors, while social and environmental stimuli increase survival.
Therefore, increasing social and environmental stimuli for people with depression may have additional benefits for cognition and pathophysiology. For humans, regular physical activity can provide increased opportunities for social interaction by engaging in group activities, and allows for the exploration of novel environments when engaging in outdoor physical activities such as running and hiking. Thus, the context in which someone undertakes physical activity may be important to altering physiology and accruing greater cognitive benefits as a result. Simply put, a walk in the park with friends may have different effects than running at home on a treadmill, or stationary cycling in a laboratory environment. Regardless, patient preference should be sought when recommending exercise, as Van Praag and colleagues (Van Praag et al., 1999) also demonstrated that mice forced to swim showed significantly less neurogenesis than their counterparts who ran voluntarily.
In addition to brain neurophysiology, it is also worth considering the effects that peripheral physiology may play in cognition. In particular, inflammation may play a role in cognition. Higher circulating levels of inflammation mediators, most notably C-reactive protein, in the blood have been associated with poorer scores on cognitive tests (Teunissen et al., 2003; Yaffe et al., 2004). Although exercise has an acute inflammatory response, regular physical activity leads to lower overall levels of inflammation (Mathur & Pedersen, 2008). Additionally, higher body mass indices are associated with cognitive deficits in learning and memory, and some studies have shown cognitive improvements after weight loss (see Sellbom & Gunstad, 2012). These cognitive improvements may be independent of any neuroplastic effects of physical activity given that improved memory was observed 12 weeks after bariatric surgery (Gunstad et al., 2011). Thus, in addition to physiological and brain responses to exercise, it is also important to consider potential contributions peripheral physiology may play in altering cognition.
As a final thought on the mechanisms of altering cognition through exercise in depression, speculatively, engaging in exercise may also play an indirect role in reducing long-term cognitive impairment associated with old age, by preventing the recurrence of depression. Several studies have demonstrated that people with a greater number of major depressive episodes across the lifespan had a significantly greater risk of developing dementia (Dotson, Beydoun, & Zonderman, 2010; Kessing & Andersen, 2004). Thus preventing the recurrence of depression may benefit life-long cognition. In a recent systematic review, Mammen and Faulkner (2013) reported that physical activity participation was associated with lower rates of future depression in 25 of 30 prospective longitudinal studies. Although this review excluded any study that included depressed individuals at baseline – combined with the evidence in support of exercise as a treatment for current depression – it is not unreasonable to consider that physical activity may also prevent recurrent depression. If this is indeed the case, it is important not only to prescribe regular physical activity to individuals who currently have depression, but also to those who have a history of depression as well.
In summary, exercise alters numerous systems that could improve cognition in people with depression. Animal physiological evidence is plentiful; however, confirming that the same mechanisms are also responsible for changes in human cognition is difficult. Responses to acute bouts of exercise are most likely driven by transient changes in brain physiology. Dopamine, norepinephrine, and serotonin are likely candidates responsible for changes in cognition post-exercise, though other neuroendocrine signals are also likely to have an effect. Increased serotonin in the brain is connected to increased release of BDNF and other neurotrophins in key regions associated with cognitive deficits in depression, such as the hippocampus. BDNF does not appear to play a role in acute responses to exercise, but likely plays a significant role in altering brain structure and physiology over the long term when physical activity is engaged in regularly. Increased neurotrophin release, neurogenesis, angiogenesis, and synaptic plasticity occur in the brain as a result of exercise, and are most likely responsible for long-term cognitive benefits accrued from regular physical activity. Finally, the cognitive benefits of exercise may vary based on the social and environmental context in which individuals engage in exercise. Exercise and physical activity can provide the opportunity to engage with others socially and explore stimulating environments, which in turn may improve cognitive function. Several mechanisms are most likely operating in concert, with the precise combination being highly individual-specific (Fox, 1999).
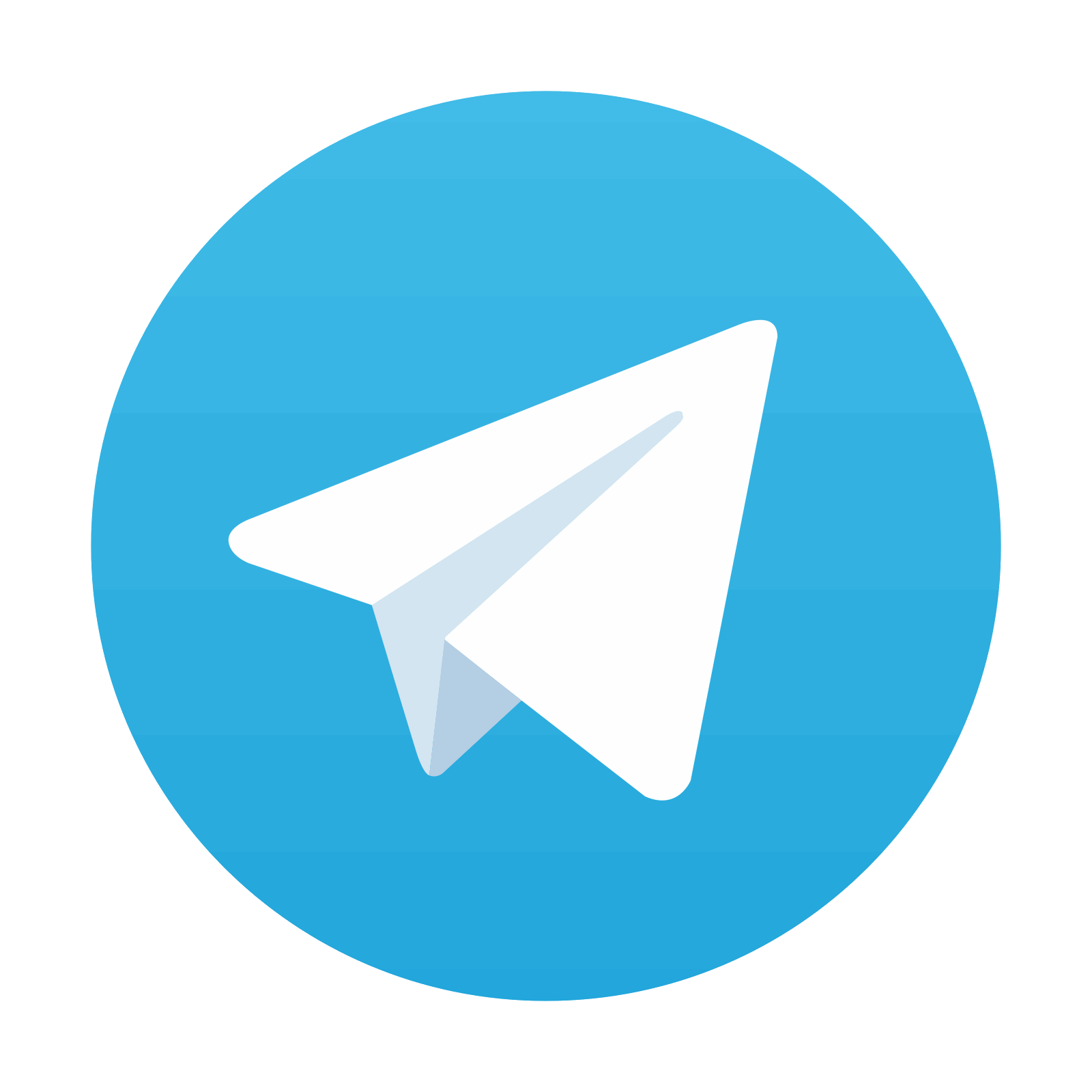
Stay updated, free articles. Join our Telegram channel

Full access? Get Clinical Tree
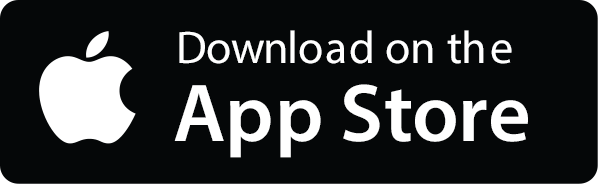
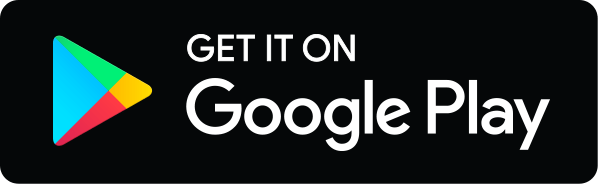