Study
Source and dose of LPS and way of application
N
Behavioral effects
Pollmacher et al. (1993)
S. abortus equi, 0.4 ng/kg i.v.
15M
Reduction in wakefulness and REM sleep, increase in non-REM sleep during the night
Korth et al. (1996)
S. abortus equi 0.8 ng/kg i.v.
10M
Slight reduction of non-REM sleep in daytime
Mullington et al. (2000)
S. abortus equi 0.2 or 0.4 or 0.8 ng/kg i.v.
19M
Non-linear, dose-dependent effects on wakefulness, REM sleep, and non-REM sleep
S. abortus equi, 0.8 ng/kg i.v.
20M
10Ma
Decrease in mood, decrease in verbal and non-verbal memory correlating with increases in pro-inflammatory cytokines. Improvement of working memory associated with altered AChE cleavage
Strike et al. (2004)
S. typhi 25 μg i.m. (Typhim Vi®)
26X
Mood decrease
Wright et al. (2005)
S. typhi 25 μg i.m. (Typhim Vi®)
30M
Mood decrease, correlation with IL-6
Krabbe et al. (2005)
E. coli 0.2 ng/kg i.v.
12M
No effects on declarative memory, but negative correlation with IL-6
S. typhi 25 μg i.m. (Typhim Vi®)
16M
Fatigue, mental confusion, increased reaction time, increased anxiety, decreased mood
S. typhi 25 μg i.m. (Typhim Vi®)
59M
Decreased mood (more decreased when combined with stress, and less decreased in optimists), increased fatigue in combination with stress
Eisenberger et al. (2010b)
E. coli 0.8 ng/kg i.v.
39X
Feelings of social disconnection and depressed mood
Grigoleit et al. (2010)
E. coli 0.4 ng/kg i.v.
24M
No effect on memory
Van den Boogaard et al. (2010)
E. coli 2 ng/kg i.v.
15M
No effect on memory, slight EEG changes indicating increased attention
Hannestad et al. (2011)
E. coli 0.8 ng/kg i.v.
11X
Social anhedonia, lassitude, and depressed mood, reduced by pre-treatment with the antidepressant citalopram
Grigoleit et al. (2011)
E. coli 0.4 or 0.8 ng/kg i.v.
34M
Dose-dependent mood decrease, dose-dependently impaired emotional memory acquisition and improved reaction speed, no effect on non-emotional memory
Kullmann et al. (2014)
E. coli 0.4 ng/kg i.v.
18M
Decreased mood, no effect on social cognition performance
Table 4.2
Neuroimaging studies employing LPS injection in humans
Study | Dose of LPS and imaging method | N | LPS effects on neural activity |
---|---|---|---|
S. typhi 25 μg i.m. (Typhim Vi®) fMRI (1.5T) | 16M | Reduced substantia nigra activity. sACC activity and connectivity to other regions upon presentation of emotional faces predict inflammation-associated mood changes. Enhanced neural activity within brainstem, thalamus, amygdala, cingulate, and insula during Stroop color word task with additional recruitment of dlPFC and MCC in response to demanding incongruent trials | |
E. coli 0.8 ng/kg i.v. fMRI (3T) | 39X | Significant reduction in ventral striatum activity to a monetary reward cue, increased activity in the amygdala in response to socially threatening images, no differences in neural response to social exclusion | |
E. coli 0.8 ng/kg i.v. PET (18F-FDG) | |||
E. coli 0.4 ng/kg i.v. fMRI (1.5T) 9X | 18M Hannestad et al. (2012b) | Enhanced orbitofrontal cortex activity in response to emotional visual stimuli. Increased responses in the fusiform gyrus, temporo-parietal junction, superior temporal gyrus, and precuneus during a social cognition task (Reading the Mind in the Eyes) Higher NGM in the insula, trend towards higher NGM in cingulate, no effect on amygdaloid NGM |
The first studies employing endotoxin to determine inflammatory effects on neuropsychological functions in humans focused on sleep. In one of the earliest endotoxin studies in humans, Pollmacher and colleagues reported that the intravenous administration of 0.4 ng LPS/kg of body weight reduced the amount of rapid eye movement (REM) sleep and promoted non-REM sleep during the night (Pollmacher et al. 1993). Subsequent studies reported similar results for daytime sleep as well as dose-dependent effects with significant sleep disruption at high doses and increases in non-REM sleep at low doses of endotoxin (Hermann et al. 1998; Korth et al. 1996; Mullington et al. 2000). In 2001, Reichenberg and colleagues published a highly recognized study in which they demonstrated for the first time that the mild immune response elicited by LPS at a dose of 0.8 ng/kg affects memory performance in humans independently from physical symptoms of sickness (Reichenberg et al. 2001). In addition to a decrease in verbal and non-verbal memory (with acquisition and retrieval within the course of the immune reaction), they also observed a decrease in mood and an increase in state anxiety. All of these changes correlated with those in cytokine secretion, predominantly that of tumor necrosis factor alpha (TNF-α). Cytokine-associated, symptom-independent mood worsening was also observed after the more subtle typhoid vaccination (Strike et al. 2004; Wright et al. 2005) and is among the most consistent and most striking observations in studies employing TLR-4 activation in humans (Dellagioia and Hannestad 2010; Kullmann et al. 2011; Schedlowski et al. 2014; see also Box 4.2).
Box 4.2 Inflammation and Depressive Disorder
Based on the notion that sickness behavior resembles depressive symptomatology (e.g., lowered mood, fatigue, decrease in activity, social withdrawal, anhedonia, altered sleep patterns) it has been frequently discussed as to what extent both phenomena are connected to each other or whether they even may represent two sides of the same coin (Dantzer 2001; Dantzer et al. 2008; Miller et al. 2009). Prolonged pro-inflammatory cytokine release (like in chronic inflammation) is suspected of mediating negative effects on mental health, especially when released in high concentrations (as seen during sepsis or auto-immune disease), or in an inappropriate compartment of the body (e.g., after damage of the blood–brain barrier). Indeed, a number of studies suggest an increased incidence for depressive disorders in persons with chronically increased levels of pro-inflammatory cytokines (Dowlati et al. 2010). In addition, a number of patients receiving therapeutic administration of certain cytokines suffer from neuropsychological disturbances like cognitive impairments or emotional disorder (Capuron and Miller 2004; Spath-Schwalbe et al. 1998). The exact mechanism linking increased levels of cytokines with the etiology of depression is not well defined, however, it is believed that alterations in tryptophan metabolism and its effect on serotonin availability may play a crucial role (Dantzer et al. 2008) as well as up-regulation of the expression and activity of the serotonin transporter (SERT) (Zhu et al. 2006). This is supported by a study demonstrating that the selective serotonin reuptake inhibitor citalopram attenuated endotoxin-induced lassitude (Hannestad et al. 2011). Considering data from fMRI studies, the dopaminergic mesolimbic system is likely to be involved (see Table 4.2 and discussion). Another important system potentially connecting depression and inflammation bi-directionally is the HPA axis. The HPA axis responds to inflammation and the immune system responds to HPA axis activity. However, dysregulation of the HPA axis is known to accompany and promote depression (Pariante and Lightman 2008). Consequently, there is evidence that inflammation can contribute to depression via activation of the HPA axis or through cytokine-induced glucocorticoid-receptor resistance (Holsboer 2000; Pace et al. 2007). Finally, there is a number of depression risk genes also known to be involved in inflammatory processes (Raison and Miller 2013a) leading some authors to the assumption that “depression confers a critical compensatory advantage in immune protection that has offset notable disadvantages of depression for evolutionary fitness” (Anders et al. 2013).
Of note, depressive disorders are not the only psychiatric disorders linked with inflammation. The pathogeneses of schizophrenia, neurodegenerative disease, and age-related cognitive decline are all known to have an inflammatory component (Akiyama et al. 2000; Drexhage et al. 2010; Giovanoli et al. 2013; Weaver et al. 2002).
The Fine Print: What to Keep in Mind When Performing an Endotoxin Study
However, subsequent studies on the impact of inflammation on memory and cognitive functions turned out to be less consistent. In a study performed in our laboratory, we were not able to reproduce the results of Reichenberg et al., as there were no observable differences in memory performance or executive functions despite a profound increase in temperature, heart rate, and levels of pro-inflammatory cytokines (Grigoleit et al. 2010). In contrast, a study by Cohen et al., based on the same sample as the Reichenberg study, reported an enhancement of working memory (Cohen et al. 2003), whereas other studies found no such effect (Brydon et al. 2008; Grigoleit et al. 2010; Krabbe et al. 2005; van den Boogaard et al. 2010). However, our laboratory demonstrated that the dose of the employed immune stimulus may have a profound and quite selective impact on the appearance of cognitive changes. In a placebo-controlled crossover study, we compared cytokine, neuroendocrine, vital, mood, and cognitive responses to a dose of either 0.4 or 0.8 ng of E. coli endotoxin per kg of body weight (see figure Box 4.1). Whereas the physiological and neuroendocrine response was the same between both conditions, there were profound differences in mood worsening, anxiety, and cognitive test results. While accuracy and psychomotor speed in a working memory paradigm did not change in response to the lower dose, we observed a significantly improved reaction time after applying the higher dose (Grigoleit et al. 2011). In addition, the source and method of application of the immune stimulus might account for differences between studies; for example, Reichenberg et al. employed 0.8 ng LPS from S. abortus equi which resulted in a mean temperature increase of 0.5 °C without alterations in heart rate, whereas in our studies, 0.4 ng/kg E. coli LPS caused a rise in temperature of nearly 1 °C and profound increases in heart rate. In Table 4.1, most of the existing studies testing cognitive performance after endotoxin challenge in humans are listed together with the employed source and dosage of endotoxin. However, even the exact serotype and batch of an endotoxin might account for differences in the results, as well as the injection time point, the exact time between injection, type of employed tests, or the sex of the subjects. Most studies recruited only male volunteers, and to our knowledge there is only one study directly addressing sex differences in the neural response to endotoxin in humans (Eisenberger et al. 2009).
The dose of endotoxin is also of importance, as the effects are not linear. Mullington et al. were the first to report a non-linear response in the amount of non-REM sleep in response to different doses of endotoxin, which follow a bell-shaped curve with the greatest amount of non-REM sleep at an intermediate LPS dosage (Mullington et al. 2000; Pollmacher et al. 2002). In one of our studies, we observed an increase in reaction time after administration of a higher LPS dose, whereas in the same study, negative effects on long-term acquisition were solely visible after applying a lower dose (Grigoleit et al. 2011). Interestingly, in this study the physiological and neuroendocrine responses were not significantly different between doses, whereas the decrease in mood and self-reported alertness were far more pronounced in response to the higher dose. The non-linear effect of endotoxin may be related to its source, as we observed the same profound increase in heart rate both after applying 0.4 or 0.8 ng of E. coli LPS/kg of body weight, whereas Mullington et al. observed only a slight increase after administration of 0.4 but a marked rise after 0.8 ng of S. abortus equi LPS. Similar differences between the two studies are observable with IL-6 levels, cortisol, and body temperature. This observation highlights the importance of standardized protocols to receive comparable data and the difficulty of matching data from different studies.
Social Aspects of Sickness Behavior and the Illuminated Brain
Since the effects of sub-septic LPS doses on memory resulted in subtle effects, scientists began to ask more specific questions based on the following three observations:
1.
Mood worsening and fatigue were the most constant and robust responses to an LPS injection.
2.
The sickness response is believed to represent an adaptive and evolutionarily meaningful reaction, so most observations one would expect should be either beneficial or inevitable.
3.
Humans are highly social beings. When inflammation induces changes in rodents’ social behavior, it should, a fortiori, do so in humans but not necessarily in the same way and direction.
Two publications based on animal studies supported this change in orientation. First, a review by Cunningham and Sanderson raised the question whether prior experiments in rodents suggesting memory impairments after immune activation might in fact rather be representing changes in the animals’ motivational state (Cunningham and Sanderson 2008). Second, a study by Willette et al. studied rhesus monkeys injected with low doses of LPS which showed a marked increase in social behavior (Willette et al. 2007), contrary to social withdrawal observed in sick rodents. Social withdrawal is a key feature of sickness behavior and is often assumed to be present in sick humans and other animals.
In 2009, two studies by Brydon et al. investigated the role of psychological stress and personality traits on the outcome of an acute immune challenge. They found that typhoid vaccination elicited a larger rise in participants’ IL-6 serum levels when it was combined with a stressful task, and the stress response to the tasks was more pronounced in the vaccinated than in the stress-only group (Brydon et al. 2009a). Furthermore, in the combined vaccine/stress group, the authors found an inverse relationship between subjects’ measures of optimism and IL-6 response and a positive correlation between optimism and antibody production after vaccination (Brydon et al. 2009b). This not only exemplifies the bi-directional brain-immune interactions in humans but also highlights a relationship that can only be investigated in a human experimental model, which underlines the importance and potential advantages of this model over animal models. Another example of a human-only study is a report in which participants showed an increase in feelings of social disconnection, which also was associated with the induction of depressed mood after receiving LPS (Eisenberger et al. 2010b). We recently investigated the effect of acute inflammation on social cognitive performance with two theory of mind (ToM) tests, which showed no effect (Kullmann et al. 2014; Grigoleit et al., unpublished).
One of the most intriguing examples for an adaptive change in social interaction in response to sickness and inflammation was recently published by Olsson et al. (2014). In this study, the authors found that the body odor of participants who received endotoxin was more aversive to blinded raters than that of control subjects, providing a potential non-verbal way of communicating sickness from one individual to another to avoid contagion.
The ability to create a well-controlled, reproducible immune response in a homogenous sample of subjects also allows for the use of imaging techniques to observe changes in brain activation and thereby identify to the neural origins of sickness and sickness-related behavior. Presently, there are data from one PET and three fMRI studies available, which are listed in Table 4.2. Using fMRI, it is possible to image and map differences in the level of blood oxygenation (BOLD signal) within the brain and thereby to draw conclusions about the degree of neural activity in respective brain regions. Brydon, Harrison, and colleagues were the first to publish an fMRI study in humans receiving endotoxin. They found that administration of the inflammatory stimulus (typhoid vaccine) had no effect on general neurovascular coupling upon visual stimulation, which was confirmed by findings from our group (Kullmann et al. 2012) and is important for the interpretation of specific changes in the BOLD response. In subjects who had received vaccination, higher activation in the left substantia nigra was observed which corresponded to the right hand which was used to press the response button during performance of the word-color Stroop task. This increase correlated with higher circulating IL-6 levels and prolonged response times in the task, which led the authors to conclude that IL-6-dependent modulations in the dopaminergic midbrain system may play a key role in transmitting the motor deficits of inflammation (Brydon et al. 2008). In a further analysis of the same sample, the authors defined the Stroop task as a model for a set of high-demand cognitive processes typically compromised by sickness. During presentation of congruent as well as incongruent stimuli, they found that typhoid vaccination enhanced neural activity within the brainstem, thalamus, amygdala, and bilateral mid and anterior insula. Moreover, there was a significant correlation between insular activation and the amount of self-reported fatigue, which was only present in the vaccine group (Harrison et al. 2009b). This observation is supported by data from animal studies that also suggest a key role of the insula, amygdala, and various brain stem regions in afferent immune-to-brain communication (Doenlen et al. 2011; Engler et al. 2011; Serrats et al. 2010). In another study, Harrison et al. explored the neural mechanisms underlying inflammation-associated mood changes. Activity in the subgenual anterior cingulate cortex (sACC) in response to emotional face stimuli and its connectivity to several other brain regions correlated with changes in total mood 3 h after vaccine injection (Harrison et al. 2009a). Since the sACC and its functional connectivity to other regions are recognized for their role in the pathophysiology of major depressive disorder (MDD), this observation might contribute to the cytokine hypothesis of depression (see Box 4.2). Another fMRI study by Eisenberger, Inagaki, and colleagues dealt with the social aspects of sickness behavior using an i.v. injection of 0.8 ng E. coli endotoxin/kg of body weight as an inflammatory stimulus. Employing an online ball-tossing game as a model of social exclusion to explore differences in the neural processing of social pain in an inflammatory context, the authors did not find meaningful differences in neural responses between LPS and placebo conditions (Eisenberger et al. 2009). However, in the endotoxin group there was an association between increases in IL-6 and social pain-related neural activity in the dorsal anterior cingulate cortex and anterior insula, which was only present in female subjects. Based upon the observation that a decrease in reward-seeking behavior (anhedonia) is a key feature of sickness behavior in rodents, Eisenberger et al. examined the neural response to anticipation of monetary rewards and found a significant reduction in corresponding ventral striatum activity 2 h after endotoxin exposure (Eisenberger et al. 2010a). The ventral striatum, which contains the dopaminergic nucleus accumbens, is considered an important reward center and as such known to be involved in the etiology of depression (Nestler and Carlezon 2006). In their third paper on this study, the authors focused on the neural response to fearful stimuli (Inagaki et al. 2012). Previous studies have shown that experimental immune responses increase general state anxiety in humans and that the amygdala, a key structure for fear and anxiety processing, responds to systemic inflammation in rodents (Reichenberg et al. 2001; Grigoleit et al. 2011; Engler et al. 2011). Inagaki et al. presented a set of socially and non-socially threatening and non-threatening visual cues to their subjects and found that endotoxin led to greater amygdala activity in response to socially threatening (fear faces) versus all other types of images. This not only verifies an important immune-neural pathway but also highlights the specificity with which the brain reacts to inflammatory stimuli. A similar approach, however, using only non-socially threatening versus neutral images, was performed at our laboratory. Intravenous injection of 0.4 ng LPS/kg enhanced activation of the inferior orbitofrontal cortex, and (albeit at a lower statistical threshold) superior and medial prefrontal regions during aversive visual stimulation. These activations, which were not associated with changes in mood or anxiety, might reflect stronger engagement in emotion regulation, reappraisal, cognitive control of emotionally salient stimuli, and recognition of other individuals’ emotions (Kullmann et al. 2012). In a subsequent report on this study, we investigated the effect of systemic inflammation on theory of mind (ToM) abilities and corresponding neural activity. Subjects were asked to detect facial expressions from pairs of eyes shown to them on a computer screen. Compared to a control condition (gender detection), all subjects showed enhanced activity in a set of regions typically engaged in ToM and face recognition with significantly higher activations within left fusiform gyrus, right temporo-parietal junction, right superior temporal gyrus, and right precuneus in the LPS compared to the placebo condition. In contrast, there was no effect on subjects’ performance in this task, so that enhanced neural activity was interpreted as a compensatory mechanism to maintain social cognition abilities despite decreased overall brain performance or motivation (Kullmann et al. 2014). A different approach to imaging brain activity during inflammation was taken by Hannestad et al. who combined the human endotoxemia model with PET. Intravenous injection of the radioactive glucose analog fludeoxyglucose (18F-FDG) allows for the tracing and mapping of changes in local glucose metabolic rates, which indicate changes in energy consumption and neural activity in the respective region. In contrast to fMRI, PET records changes over a larger time frame of several minutes and independently of an experimental stimulus. Hence, it more closely represents a general engagement or tonus of a certain region than its recruitment in a specific task. Hannestad and colleagues found significantly increased normalized glucose metabolism (NGM) in the insula and a trend towards decreased NGM in the cingulate cortex without a general effect on global cerebral metabolic rate of glucose (CMRGlu), with secondary analyses suggesting the main effect occurred in the right anterior insula and right anterior cingulate (Hannestad et al. 2012b). Contrary to the authors’ expectation, there was no effect on amygdala NGM. Unfortunately, there were no measures of anxiety employed in this study; however, in another study using a similar design of endotoxin application, the same authors did not find effects on anxiety (Hannestad et al. 2011). A great advantage of PET is that it is not restricted to parameters related to energy consumption like glucose metabolism or blood oxygenation to image changes in the brain. The density and engagement of neurotransmitter receptors or surface antigens might be explored as long as a sufficient tracer is available. Recently, the authors of the aforementioned studies demonstrated engagement of microglia in the baboon in response to peripheral endotoxin application using 11C-PBR28 as a ligand for translocator protein, a marker of microglial activation (Hannestad et al. 2012a).
Beyond Sickness: Other Uses of Human Endotoxemia in PNI Research
One of the strengths of the presented model is its versatility. Beyond exploring the effects of an inflammatory response on cognition, social behavior, and corresponding neural correlates, it was also used in other experiments addressing important questions in the field of PNI. In a study performed in our laboratory, we attempted to pair immunogenic effects of endotoxemia to a gustatory stimulus via classical conditioning, which had already been successfully utilized to condition immunosuppression in humans (Wirth et al. 2011). Although we failed to induce a conditioned inflammatory response, we observed a significant conditioned aversion against the conditioned stimulus solely in the endotoxin treated group, highlighting a potential evolutionary strategy to prevent the organism from pathogen-contaminated food (Grigoleit et al. 2012). In another study, we were able to demonstrate inflammation-induced release of salivary α-amylase, an enzyme which was recently suggested as a novel marker of sympathetic activity (Grigoleit et al. 2013). A few recent studies demonstrated that systemic inflammation dose-dependently increases visceral and somatic pain sensitivity (Benson et al. 2012; Hutchinson et al. 2013; Wegner et al. 2014). Exploring the interaction of sickness behavior and depression (see Box 4.2) Hannestad, DellaGioia, and colleagues investigated the effect of antidepressant treatment on endotoxin-induced fatigue and other depressant symptoms. Whereas pre-treatment with the selective serotonin-reuptake inhibitor citalopram significantly reduced endotoxin-induced lassitude and depressive symptomatology, pre-treatment with buprion, a norepinephrine and dopamine reuptake-inhibitor failed to display such an effect (Dellagioia et al. 2012; Hannestad et al. 2011). Although the latter study suffers from relatively low statistical power and is therefore considered preliminary, these reports demonstrate for the first time a direct connection between sickness behavior and depression with an involvement of the serotonergic system in humans. In addition, these findings may support the recently discussed possibility to adopt human endotoxemia as an experimental model of depression (DellaGioia and Hannestad 2010).
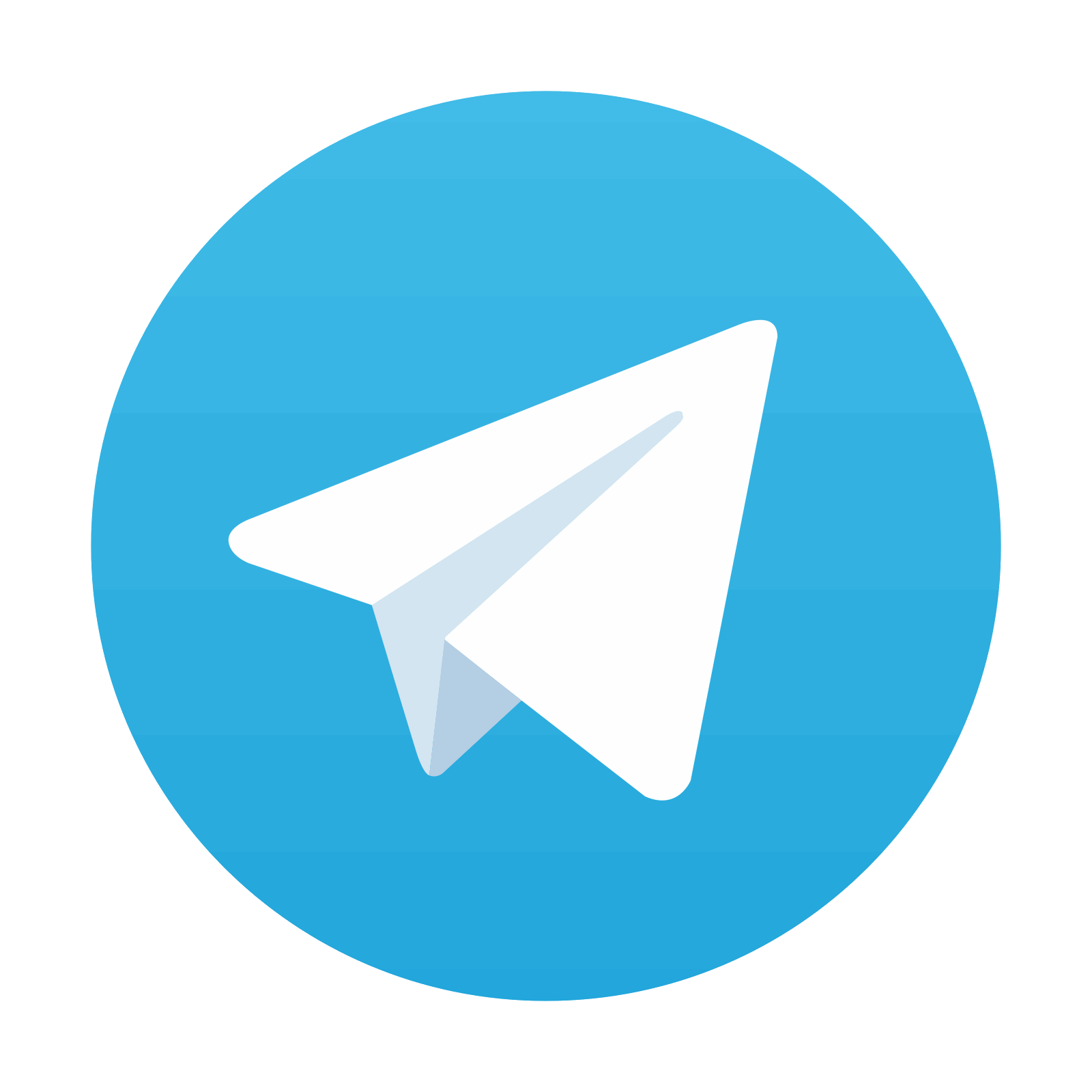
Stay updated, free articles. Join our Telegram channel

Full access? Get Clinical Tree
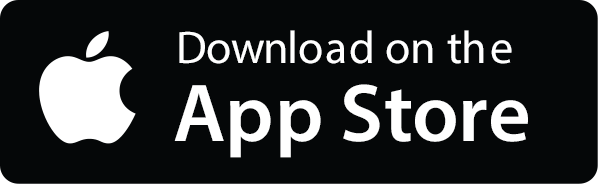
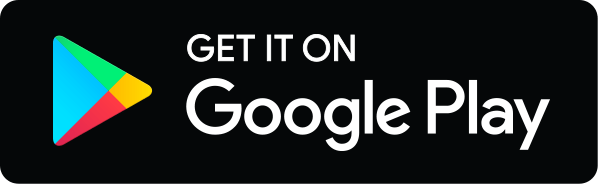