Fig. 19.1
Behavioral paradigm and recording of inter-laminar activity in PFC. Behavioral task and Prefrontal columnar recording. (a) Diagram of NHP brain with PFC recording locations in areas 46, 8, 6. (b) Behavioral paradigm showing sequence of events in DMS task. (1) ‘Focus ring’ presentation and response to initiate the trial commencing with (2) presentation of the ‘sample target’ image, followed by (3) ‘sample response’ by cursor movement into the image which initiated (4) a variable ‘delay’ period of 1–90 s prior to (5) the presentation of the ‘match’ target (sample image) accompanied by 1–6 non-match (distracter) images on the same screen. Cursor movement into correct (match target) image for 0.5 s was rewarded by juice reward (0.5 ml) via a sipper tube next to the animal’s mouth. Placement of the cursor into a non-match image for 0.5 s caused the screen to blank without reward delivery. Intertrial interval (ITI): 10.0 s. (c) Histologic section of DLPFC brain showing relative location of supra-granular layer 2/3 (L2/3, blue) and infra-granular layer 5 (L5, red) with tract used for placement of conformal multielectrode recording array (MEA) probe (W3). (d) Correlated inter-laminar activity within two adjacent prefrontal minicolumns (1&2) during DMS task performance. Center panel illustrates conformal MEA positioned for simultaneous inter-laminar columnar recording from adjacent minicolumns 1 and 2 (40 μm separation) from L2/3 and L5 cell pairs (blue and red extracellular spike waveforms). Associated cell activity is shown as individual trial rasters and average PEHs obtained from both cell pairs recorded simultaneously from L2/3 (blue) and L5 (red) within the respective minicolumns (1&2). Rasters and PEHs depict ±2.0 s relative to onset (0.0 s) of Match phase screen on each trial (Fig. 19.1b) within a single DMS session. In the middle of the raster-PEH displays are shown cross-correlation histograms (CCHs) for the same cell pair minicolumns which show display increased inter-laminar synchronization during target selection (green) in the match phase (0.0 + 2, 0 s, post) relative to similar but reduced correlations between the same cell pairs constructed 2.0 s prior to (black, pre) Match phase onset (−2.0 to 0.0 s in PEHs) (Compiled from Opris et al. 2012a, b)
The DMS task required hand-tracking movements by NHPs to place a cursor into different positions on a screen to obtain a juice reward for selection of the correct (sample) image, the location of which varied randomly in one of seven spatial positions on the screen on each trial (Hampson et al. 2004b). Key variables in the task were the number of images (2–7) presented on the screen in the match phase of the trial, the duration of the interposed delay (1.0–90.0 s), as well as the random placement of the sample image in the match phase (after the delay interval) that differed from the position responded to in the sample phase. In addition, to incorporating key cognitive features such as attention, short-term memory, cognitive workload and reward expectancy, subjects were also executing a ‘decision process’ in the match phase (Fig. 19.1b) which involved ‘target selection’, i.e. a process that was related directly to columnar related neuron firing in the PFC (Hampson et al. 2011).
A demonstration of this type of columnar processing is shown in Fig. 19.1d for two adjacent PFC minicolumns recorded simultaneously with the MEA during the match phase of the task. Raster/PEHs for the two simultaneously recorded cell pairs (L2/3 and L5) at the indicated locations on the conformal MEA array (Fig. 19.1d minicolumns 1&3) positioned via pad separation dimensions of 1300u are shown in Fig. 19.1c. There were significant increases in overall mean firing rates at the onset of the match phase (‘M’ onset = 0.0 s; pre vs. post, p < 0.001) over the time (from 0.0 to + 2.0 s) in which arm movements involved in target selection were initiated (Hampson et al. 2011, 2012b; Opris et al. 2012a; Porrino et al. 2013). In addition, the L2/3 neurons shown in Fig. 19.1d (upper raster/PEHs) exhibited significantly higher overall mean firing rates (p < 0.001) than the L5 neurons (lower raster/PEHs) recorded in minicolumns 1 and 2 on the MEA during the same phase of the task. Most important was the determination that the firing of cell pairs recorded in the same vertical positions on the MEA reflected columnar-based interlaminar communication via cross-correlations between cell pairs (minicolumns 1 and 2, Fig. 19.1d) which showed increased firing synchrony at the time of target presentation and response selection in the match phase.
The normalized CCHs in Fig. 19.1d depict differences in the correlation of L2/3 and L5 firing of the same cell pairs with respect to (1) the ‘pre’ match phase onset period (M = 0.0) baseline (−2.0–0.0 s) versus after presentation of images on the screen in the ‘post’-match phase (0.0 s to +2.0 s) onset period (Fig. 19.1b). The specificity of the cross-correlations between both L2/3 and L5 cell pairs (minicolumns 1 and 2) is shown in Fig. 19.1d by the significant increase in CCHs (p < 0.001) during performance of the Match response (green, Post-Match) relative to the ‘pre’ match phase period when the animal was waiting for the Delay interval to time out prior to image presentation (black, Pre-Match). Results revealed reduced correlated firing between cell pairs within single minicolumns on error trials with inappropriate target selection (Opris et al. 2011, 2012a, b). This was a direct demonstration of task-specific, real-time columnar processing in prefrontal cortex indicating the role of inter-laminar microcircuits in executive control of decision-making in primate brain.
19.3 Derivation by MIMO Model of PFC Columnar Processing
19.3.1 Application of the MIMO Model to PFC Columnar Processing
In prior studies (Berger et al. 2011; Hampson et al. 2012b, Hampson et al. 2013; Opris et al. 2013), it has been shown that a multi-input multi-output model (MIMO) nonlinear dynamic model applied to spatiotemporal patterns of multiple recordings from synaptically connected neurons is capable of extracting patterns of firing related to successful task performance of this same task shown in Fig. 19.1a. It was shown that the MIMO model could be used to facilitate and recover performance when administered as patterns of electrical pulses to the same recording locations from which output signals were detected on correct trials (Hampson et al. 2012a, 2013; Berger et al. 2011). The MIMO model applied to minicolumnar data recorded by the conformal MEA probes in the animals performing the DMS task shown in Fig. 19.1 as a modeling strategy for the nonlinear dynamics underlying spike-train transformations between L2/3 and L5. This was then used to predict L5 output firing patterns from input patterns of L2/3 neural activity, as a representation of multicolumnar firing patterns (Berger et al. 2005, 2011, 2012; Hampson et al. 2012a; Song et al. 2007, 2009). In this application, the identification of spatio-temporal pattern transformation from the PFC layer 2/3 to layer 5 in MEA identified columns was formulated by the MIMO model and analyses included extraction of the first-, second- and third-order temporal firing within at least two defined minicolumns on MEAs inserted repetitively on multiple recording sessions in order to extract relevant patterns of minicolumnar activity related to successful image selection during the match phase of the task.
The same MIMO model was applied in this study to multicolumnar PFC L2/3 and L5 cell pairs recorded during the Match phase of the DMS task. Spatiotemporal patterns associated with trials of correct performance were constructed from 2–4 L2/3 and L5 confirmed MEA cell pairs recorded in five different NHPs (n = 5) performing the same task. MIMO model derived L5 output firing associated with L2/3 input firing, was used to predict performance on individual trials. Figure 19.2 shows the relationship of L5 firing to task performance with respect to the firing patterns extracted by the MIMO model. Performance of the task shown in Fig. 19.2a was differential with respect to the number of distracter images with an increase in image number associated with poorer performance. However if performance on trials was segregated and the type of MIMO output associated with performance identified, a clear distinction between the types of patterns present on successful (strong code) vs. poorer (weak code) performed trials for each number of images was clearly present as shown in Fig. 19.2b, c. The MIMO model clearly can derive appropriate firing patterns but what is more important detection of patterns that are less appropriate for successful performance can be predicted by monitoring the associated L2/3 input patterns for wrong code trials online that provides time for modifying the L5 firing pattern on the same trial.


Fig. 19.2
Prefrontal cortical module code strength vs. DMS performance. (a) Behavioral performance, quantified as percent correct, segregated according to normal average performance over all trials (blue), above average performance on trials in which spatiotemporal strong code patterns (red) were detected, or trials in which performance was below average and different “weak code” (green) patterns detected. Heat maps show the time course of PFC layer 2/3 cell firing (n = 20 cells) on MIMO model derived strong code (b), vs. weak code (c) trials, shown in (a) prior (−2.0 to 0 s) to and following (0 to +2.0 s) Match Phase presentation (MP)
19.3.2 Facilitation of PFC Columnar Processing by MIMO Microstimulation
Prior investigations applying MIMO model-derived stimulation patterns to hippocampus in the rodent provided the means to enhance performance and overcome deficits induced by pharmacological treatments (Berger et al. 2011). In this manner, predictions of the L5 output related to the successful performance were monitored online during the task to define when successful or non-successful trials were about to be completed prior to target selection in the Match phase as shown in Fig. 19.3. This online monitoring by the MIMO model provided the basis for interposing the correct (strong code) L5 firing pattern as electrical pulses delivered to the same L5 recording pads of the MEAs at the same time as normal occurrence after Match phase onset and completion of target selection (Fig. 19.3). Stimulation pulses consisted of biphasic constant current square waves, 0.5 ms per phase, adjusted in intensity (10–50 μA) to produce local field potentials monitored on adjacent L5 recoding pads on the same MEA. Model-derived stimulation was applied on 50 % of trials in each session to compare stimulated and non-stimulated trials in terms of correct performance. Effective stimulation patterns that were determined to facilitate performance under normal conditions were also applied at the same time during the trial, irrespective of prior L2/3 activity, when employed for the recovery of function as a neural prosthesis as described below (Fig. 19.5). In this manner, it was demonstrated that the MIMO model served as a prostheses for recovering decision making that required appropriate PFC columnar processing related to successful target selection in the DMS task.


Fig. 19.3
Columnar microstimulation delivered via MIMO model. Online application of MIMO model for calculating Match phase response codes from L2/3 recordings and delivering electrical stimulation pulses to L5 recording pads that mimic prior associated L5 strong codes during the same trial. Schematic at left shows prefrontal cortical (PFC L2/3) recording and the NHP MIMO model with feedback stimulation applied to PFC L5. Neural recordings from layer 2/3 are analyzed to predict layer 5 neural activity from multilevel temporal analyses, which is used to generate stimulation patterns applied to layer 5 recording sites after assessment of L2/3 patterns on the same trial. MIMO model coefficients applicable to PFC recordings distinguish different features of the DMS task. This has provided the means to test the specificity of the MIMO codes recorded in L2/3 that occur on different types of trials (with different cognitive load) when applied as stimulation patterns to L5
The effects of MIMO generated electrical stimulation patterns averaged over all NHPs and trials (Fig. 19.4) were highly significant (p < 0.001, ANOVA). It is clear that MIMO stimulation facilitated performance above normal levels but was not consistent for the same types of trials in all animals (Fig. 19.4a, individual NHPs); however, Fig. 19.4b shows that the overall effect of MIMO stimulation was to significantly facilitate performance in all animals across trials with increased number of images (p < 0.001). Figure 19.4c shows an additional facilitatory effect of the MIMO-derived stimulation on trials with longer delays (30–60 s, 61–90 s) where performance was less impaired on stimulated versus nonstimulated trials (p < 0.001). Finally, the facilitatory effects of MIMO stimulation on target selection and execution were strongly supported by a significant decrease (F(4,401) = 9.14, p < 0.01) in the latency to make the match response (Fig. 19.4d) as a function of trial difficulty (number of images) on MIMO stimulation versus nonstimulation trials. The controls associated with these results are found in Hampson et al. (2012b).


Fig. 19.4
Facilitation of DMS performance by MIMO microstimulation. Facilitation of DMS performance by MIMO stimulation (a). MIMO stimulation applied to prefrontal cortex in five different NHPs (indicated by number) performing the DMS task under normal conditions. MIMO model utilized L2/3 input to predict L5 output patterns delivered as electrical stimulation (Fig. 19.3) during target image selection in the Match phase of the task (Fig. 19.1 Behavioral graphs for each animal show mean DMS performance (±SEM) as a function of trial complexity indicated by number of images (2–7) for 3–5 sessions consisting of 120 trials each). Performance is shown on normal trials in which stimulation was not delivered (No Stim) and trials in the same session in which the MIMO model stimulation was delivered to PFC L5 as shown in Fig. 19.3. Dashed line indicates performance that could be achieved by random ‘chance’ selection at each degree of match difficulty related to the number of images to select from. Inset: average performance summed across trials, animals and sessions for Control (nonStim) vs. MIMO Stim trials. **F(5,239) > 42.16, p < 0.001 increase compared to no stimulation. (b) Mean DMS performance as a function of images across all animals shown in (a) for stimulation versus no stimulation trials. **F(1,239) > 18.34, p < 0.001 increase compared to no stimulation. (c) Effect of MIMO stimulation on DMS trials with differing delays as a function of number of images. Same results shown in (b) sorted by duration of trial delay prior to match phase onset (Fig. 19.1a) for no stimulation versus stimulation trials. *F(1,239) > 8.22, p < 0.01; **F(1,239) > 13.40, p < 0.001 increase compared to no stimulation. (d) Effect of MIMO stimulation on mean Match response (MR) latency to respond to the match image on stimulation versus no stimulation trials. Mean (±SEM) latencies (in seconds) across animals and sessions as a function of number of images presented in the match phase. *F(1,239) = 9.51, p < 0.01; **F(1,239) > 21.29, p < 0.001 versus no stimulation (With permission Hampson et al. 2012a)
19.4 Drug Induced Disruption of Modularity and Cognition
19.4.1 Pharmacologic Agents Disrupt Microanatomic Processing and Cognitive Performance
Extensive prior investigation of features that affect cognitive processing in DMS tasks have shown that Match phase activation of PFC is altered by the modulation of dopamine influences on task-related PFC cell firing by cocaine and other agents that alter dopamine uptake (Hampson et al. 2011; Porrino et al. 2013; Arnsten and Robbins 2009). To determine similar actions on PFC columnar activity in this task firing was assessed in the same minicolumns before and after systemic injection of cocaine (0.40 mg kg−1) midway through the DMS session. Figure 19.5a shows raster/PEHs for a PFC inter-laminar cell pair (L2/3 upper, L5 lower) recorded: (1) in the first 60 trials of a DMS session (IV Saline control) followed by, (2) the un-signaled administration (IV) of cocaine on trial 61 in the same session of 120 trials. It is clear that a significant reduction in match phase firing occurred in L2/3 (p < 0.001) and L5 (p < 0.001) in the cocaine versus control half of sessions (Fig. 19.5a), and that these changes in firing resembled closely the type of reduced firing that occurs on error trials (Opris et al. 2012a). The generality of this effect on match phase firing over a large population of cell pairs (n = 30) is shown in Fig. 19.5b as a significant decrease in the L2/3 cell activity (p < 0.001) relative to the saline half of the session. The reduction in L5 average firing rates in the cocaine half of the session approached but did not reach significance (p > 0.10) which perhaps reflects decreased dopamine sensitive processes (Gulledge and Jaffe 1998) relative to L2/3 cells as has been indicated in prior studies of dopamine receptor actions in these PFC layers in NHPs (Bordelon-Glausier et al. 2008; Arnsten and Robbins 2009). However, the more specific columnar firing indicator, CCHs for the single cell pair in Fig. 19.5a and for all cell pairs in Fig. 19.5b (n = 30), showed mark clear decreases in correlated firing (p < 0.001) after cocaine administration (Fig. 19.5c, d). The significance of this change with respect to cocaine’s effect on correlated firing of cells in L2/3 and L5 is shown in Fig. 19.5e as a scatter plot of correlation coefficients for control and cocaine halves of the same behavioral sessions. The lack of points along the diagonal line in the scatter plot indicates a decrease in synchronized firing in cell pairs that showed high correlations in the first half of the session, after cocaine administration in the second half of the same session. Finally, it is an important coincidence that the alterations in columnar processing produced by cocaine also influenced task performance in a manner consistent with cognitive demand as shown in Fig. 19.5f by the reduction in mean selection accuracy during the cocaine half of the session as a function of trial difficulty on trials with increased numbers of images (p < 0.001). These data are supported by other findings showing impairment in cognitive function by agents which alter activity in PFC (Arnsten 2000; Arnsten and Robbins 2009; Wang et al. 2011).


Fig. 19.5
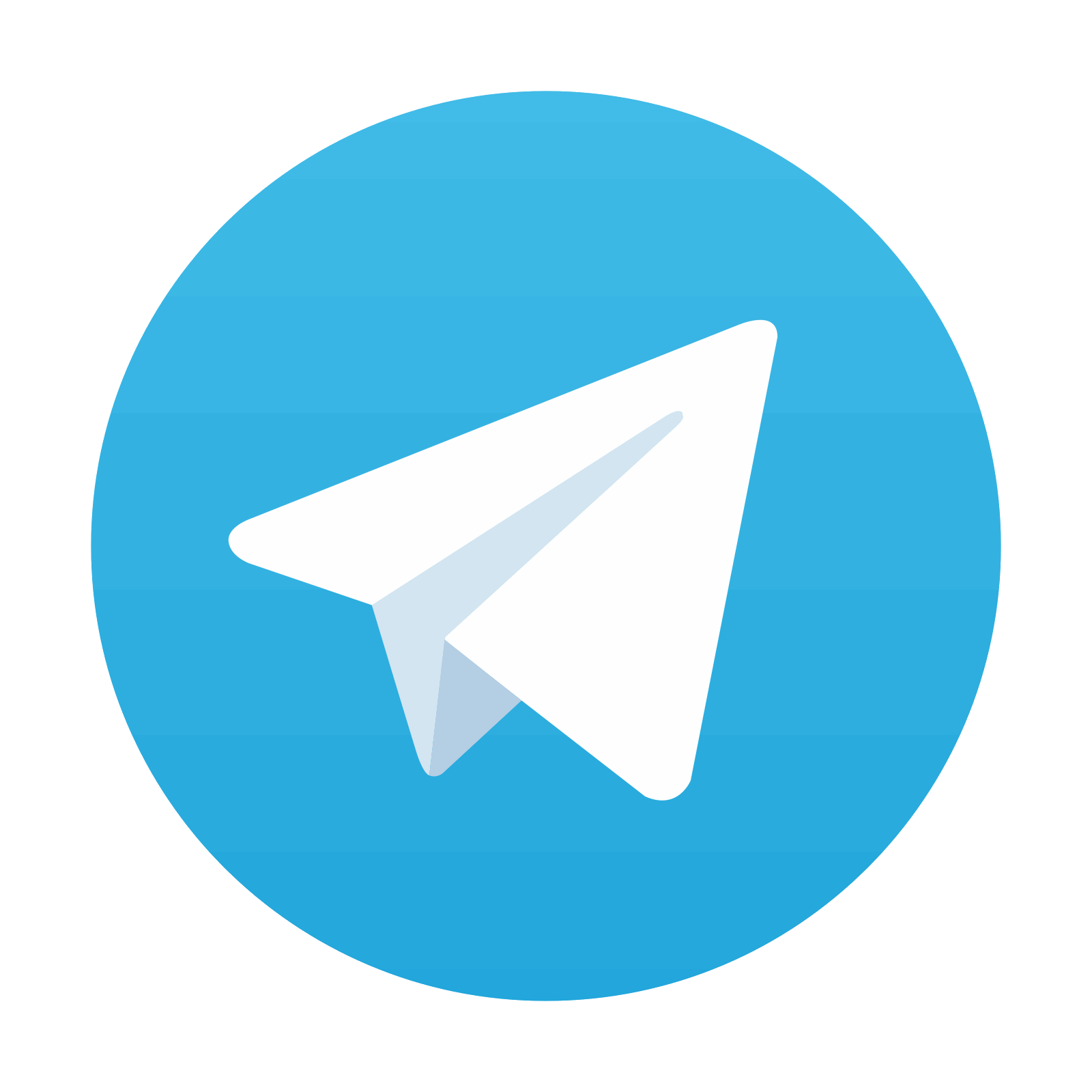
Disruption of cortical modularity and cognition. Pharmacological interruption of DMS-dependent inter-laminar processing. Effects of administration cocaine (0.40 mg kg−1 IV) at midsession on MEA L2/3-L5 cell pair firing during performance of DMS task. (a): Rasters and PEHs show Match phase L2/3 and L5 inter-laminar activity during the initial control (saline, blue) portion of the session and after cocaine administration (cocaine, red) midway through the same session. **F(1,958) > 19.72, p < 0.001 versus Control (saline). (b) Average PEHs for control (upper) versus cocaine trials (lower) summed across animals over all inter-laminar L2/3 (blue) & L5 (red) PFC cell pairs (n = 30) recorded in the same sessions with cocaine administered at the midpoint (trial 62) of the session. Blue (control) and red (cocaine) histograms show mean frequency distributions of associated MR latencies relative to Match phase onset (M, 0.0 s) for the same trials. *F(1,958) = 13.43, p < 0.001. (c) Cross-correlograms (CCHs) of firing between the L2/3 and L5 cell pair shown in (a) constructed from control trials (n = 62) in the first half of the session (blue–left), and after cocaine administration during the second half of the same session (red–right). **F(1,401) = 17.22, p < 0.001 versus Control. (d) Mean cross-correlation histograms (CCHs) for the same inter-laminar cell pairs (n = 30) shown in (b) constructed from trials in the control (blue) versus cocaine (red) halves of the session. **F(1,401) = 11.22, p < 0.001, versus Control. (e) Scatter plot of normalized cross-correlation coefficients from cell pairs shown in (d) for control (horizontal axis) and cocaine (vertical axis) halves of the same DMS sessions. Distribution of coefficients along the diagonal line would represent no change in correlation coefficients between the two halves of the session, whereas the demonstrated asymmetry of coefficient distribution reflects a significant change in inter-laminar correlated cell firing after cocaine administration. (f) Reduction in DMS (% correct) performance for all animals on trials with varying number of images (Fig. 19.4) for control versus cocaine segments of the same sessions (n = 19). **F(1,239) > 16.01, p < 0.001 Cocaine versus Control (With permission Hampson et al. 2012a)
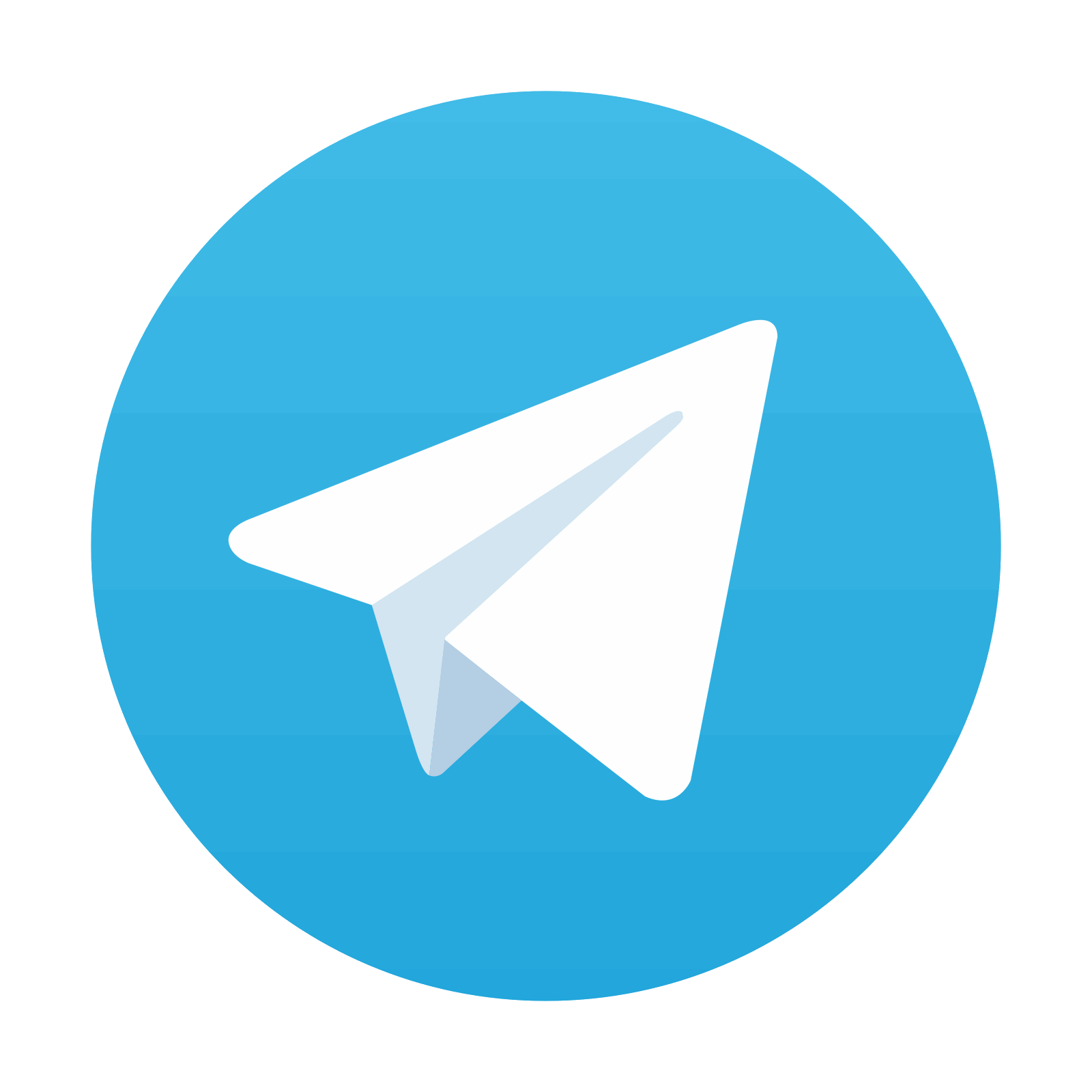
Stay updated, free articles. Join our Telegram channel

Full access? Get Clinical Tree
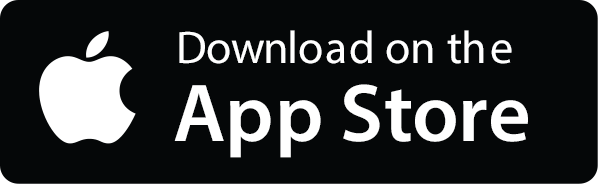
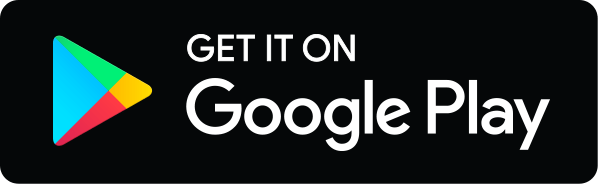
