Fig. 1
Activation of SFKs results in BBB disruption and brain edema formation in the acute stage, but leads to BBB self-repair and brain edema resolution in the recovery stage after acute brain injury, such as ICH, TBI, and ischemic stroke
Tissue Specificity, Structure, Activity, and Functions of SFKs
Several SFK family members (c-Src, Fyn, Yes, Yrk) are ubiquitously expressed, whereas others (Lyn, Fgr, Hck, Blk, Lck) are generally found in brain and hematopoietic cells [47, 67–72]. In adult mice, Fyn and c-Src mRNA expression is highest in hippocampal neurons [73, 74]. Importantly, one tissue can express multiple SFK members, for example, Src, Fyn, Yes, and Lck have been examined in brain [47, 67–72], and the different SFK family members are often found to compensate for one another [75].
Structurally, SFK family members share a conserved domain structure consisting of consecutive SH3 (polyproline type II helix for protein-protein interaction), SH2 (phosphotyrosine recognition), and SH1 (tyrosine kinase catalytic activity) [43]. They also contain a membrane-targeting region at their N-terminus that is followed by a unique domain of 50–70 residues, which is divergent among family members [43]. Although still incompletely clear, Src activity is regulated by tyrosine phosphorylation at two sites (one is at Tyr416 in the SH1 domain, the other at Tyr527 in the short C-terminal tail), but with opposing effects. Whereas phosphorylation at Tyr416 activates Src, phosphorylation at Tyr527 results in inactivation [72, 76].
Under normal physiological conditions, SFKs are implicated in the regulation of embryonic development, cell growth, cellular differentiation, and inflammatory responses [74, 77–79]. SFKs can initiate negative feedback to prevent their sustained activation via recruitment of inhibitory factor C-terminal Src (Csk) [80]. The feedback loop consists of SFK activation leading to phosphorylation of Csk binding protein (Cbp), and the phosphorylated Cbp targets Csk to SFKs and promotes inhibitory Csk phosphorylation of SFKs [81].
Because of mutations in SFKs or Csk, aberrant activation of SFKs can occur in cancers, and the abnormal SFK signaling contributes to many aspects of tumor development, including proliferation, survival, adhesion, migration, and invasion, as well as metastasis [82–84]. Thus, it is likely that targeting SFKs may be a promising therapeutic approach for cancer, as SFK antagonists have been tested and well tolerated in cancer clinical trials [85–88].
We and others have demonstrated a new function of SFKs in acute brain injury, that is, transient activation of SFKs associated with BBB disruption, brain edema, and spatial memory deficits after experimental ICH (intracerebroventricular fresh blood or thrombin model), TBI (lateral fluid percussion (LFP) model), and stroke (middle cerebral artery occlusion (MCAO) model) [18, 19, 63–66].
SFK Activation, Excitotoxicity, BBB Disruption, and Brain Edema
After acute brain injury (i.e., ICH, TBI, ischemic stroke), there occurs a transient increase of glucose utilization and local cerebral blood flow [53, 89, 90], presumably because of the actions of glutamate in blood at the time of brain injury. This was supported by findings that glucose hypermetabolism could be blocked by antagonists of NMDA and AMPA receptors [53, 90]. However, glutamate alone could not explain the hypermetabolism, inasmuch as glutamate injected directly into brain does not produce hypermetabolism [53]. This suggests that acute brain injury affects NMDA receptors in some way to make them more sensitive to glutamate so as to mediate brain injury and/or hypermetabolism.
A large number of studies have revealed that the molecules released after acute brain injury (e.g., adenosine, thrombin, cytokines) can activate SFKs [10–32], and SFKs directly bind NMDA receptors and modulate their activity [45–50]. Our data show that either an NMDA receptor inhibitor (MK801) or an SFK inhibitor (PP2) is able to prevent brain edema and improve behavioral outcomes after intracerebroventricular injection of thrombin in rats [19]. Therefore, it is plausible that SFKs and NMDA receptors are coupling to mediate calcium overload, glucose hypermetabolism, and brain edema after acute brain injury.
SFK Activation, Mitogenic Signaling, Brain Edema Formation, and Resolution
SFKs can be activated by many trans-membrane receptors, such as adhesion receptors, tyrosine kinase receptors, G protein-coupled receptors, cytokine receptors, and others [44]. This unique feature of SFKs makes them a point of convergence for many toxic molecules that are released after brain injury [10–40]. Most of these molecules are abruptly released and reach peak concentrations within a couple of hours to a day after brain injury. In the acute stage, overactivated SFK mitogenic signaling causes neurons to enter the cell cycle and die, and damages astroctyes and endothelial cells via MAPKs or CdKs [14, 19, 20, 51–62]. The disruption of BBB components increases BBB permeability, resulting in brain edema after acute brain injury.
Within about a day after acute brain injury, the molecules resolve gradually, and the disease progresses to a recovery stage of brain injury. The restored moderate SFK/mitogenic signaling leads to birth of new endothelial cells, astrocytes, and other cells that mediate BBB self-repair and brain edema resolution. Recent studies suggest that a number of stem cells exist throughout the mammalian brain, and some of these are associated with vascular niches [91]. Such stem cells could serve as a source of newborn endothelial cells, astrocytes, and other cells of the neurovascular unit that would play a major role in reestablishing the BBB after brain injury [92].
Although SFK inhibitors prevent toxicity signaling at the acute phase after ICH, they also block cellular proliferation of stem cells to delay and prolong BBB self-repair [55, 56, 93, 94]. This may provide at least a partial explanation for the findings that (1) acute single administration of SFK inhibitors (PP2, 1mg/kg, i.p. (intraperitoneally) immediately after ICH) can attenuate the BBB disruption and brain edema induced by intracerebroventricular injection of thrombin [20, 52] and (2) that delayed and chronic administration of SFK inhibitor (PP2, 1 mg/kg, i.p. daily, days 2 through 6) prevents thrombin-induced BBB repair and brain edema resolution in rats [20, 52].
Additionally, SFKs also activate hypoxia-inducible factors (HIFs) that can increase BBB permeability for brain edema formation or promote angiogenesis for brain edema resolution after brain injury through expression of aquaporins (AQPs), matrix metalloproteinases (MMPs), vascular endothelial growth factor (VEGF), BBB proteins (i.e., occluding), and others [95–97]. Interactions and cross-talk with these and other molecules and pathways add complexity to timing and development of appropriate treatment strategies involving the SFKs.
Future Directions
Future studies need to address exactly which specific SFK members found in brain (e.g., Src, Fyn, Lck, and Yrk) mediate edema after acute brain injury. In view of findings that SFKs also play critical roles in brain edema resolution, the therapeutic time window of SFK inhibition should be studied for treating edema following acute brain injury while avoiding the possible side effects caused by chronic inhibition of SFKs. A nanoparticle-based siRNA transfection system can be used for knockdown of individual SFK genes, as it allows transient knockdown of target genes, high efficiency of in vivo siRNA delivery, high specificity for gene targets, low cytotoxicity [98, 99], and is approved by the FDA for human use [100–103].
Disclosure
The authors acknowledge the support of AHA Beginning Grant-in-Aid 12BGIA12060381 (DZL) and National Institutes of Health grant RO1NS089901 (DZL).
References
1.
Marmarou A (2003) Pathophysiology of traumatic brain edema: current concepts. Acta Neurochir Suppl 86:7–10PubMed
2.
Thiex R, Tsirka SE (2007) Brain edema after intracerebral hemorrhage: mechanisms, treatment options, management strategies, and operative indications. Neurosurg Focus 22, E6PubMed
3.
Kasner SE, Demchuk AM, Berrouschot J, Schmutzhard E, Harms L et al (2001) Predictors of fatal brain edema in massive hemispheric ischemic stroke. Stroke 32:2117–2123PubMed
4.
Donkin JJ, Vink R (2010) Mechanisms of cerebral edema in traumatic brain injury: therapeutic developments. Curr Opin Neurol 23:293–299PubMed
5.
Rosenberg GA (1999) Ischemic brain edema. Prog Cardiovasc Dis 42:209–216PubMed
6.
Iencean SM (2003) Brain edema – a new classification. Med Hypotheses 61:106–109PubMed
7.
Sadaka F, Veremakis C (2012) Therapeutic hypothermia for the management of intracranial hypertension in severe traumatic brain injury: a systematic review. Brain Inj 26:899–908PubMed
8.
Pitfield AF, Carroll AB, Kissoon N (2012) Emergency management of increased intracranial pressure. Pediatr Emerg Care 28:200–204; quiz 205–207PubMed
9.
Sandsmark DK, Sheth KN (2014) Management of increased intracranial pressure. Curr Treat Options Neurol 16:272PubMed
10.
Prins M, Greco T, Alexander D, Giza CC (2013) The pathophysiology of traumatic brain injury at a glance. Dis Model Mech 6:1307–1315PubMedCentralPubMed
11.
Namjoshi DR, Good C, Cheng WH, Panenka W, Richards D et al (2013) Towards clinical management of traumatic brain injury: a review of models and mechanisms from a biomechanical perspective. Dis Model Mech 6:1325–1338PubMedCentralPubMed
12.
Gaetz M (2004) The neurophysiology of brain injury. Clin Neurophysiol 115:4–18PubMed
13.
Perel P, Roberts I, Bouamra O, Woodford M, Mooney J et al (2009) Intracranial bleeding in patients with traumatic brain injury: a prognostic study. BMC Emerg Med 9:15PubMedCentralPubMed
14.
Liu D, Sharp FR, Van KC, Ander BP, Ghiasvand R et al (2014) Inhibition of Src family kinases protects hippocampal neurons and improves cognitive function after traumatic brain injury. J Neurotrauma 31:1268–1276PubMedCentral
15.
NINDS (2013) Traumatic brain injury: hope through research. http://www.nindsnihgov/disorders/tbi/detail_tbihtm
16.
Ray SK, Dixon CE, Banik NL (2002) Molecular mechanisms in the pathogenesis of traumatic brain injury. Histol Histopathol 17:1137–1152PubMed
17.
Xi G, Reiser G, Keep RF (2003) The role of thrombin and thrombin receptors in ischemic, hemorrhagic and traumatic brain injury: deleterious or protective? J Neurochem 84:3–9PubMed
18.
Sharp F, Liu DZ, Zhan X, Ander BP (2008) Intracerebral hemorrhage injury mechanisms: glutamate neurotoxicity, thrombin, and Src. Acta Neurochir Suppl 105:43–46PubMed
19.
Liu DZ, Cheng XY, Ander BP, Xu H, Davis RR et al (2008) Src kinase inhibition decreases thrombin-induced injury and cell cycle re-entry in striatal neurons. Neurobiol Dis 30:201–211PubMedCentralPubMed
20.
Liu DZ, Ander BP, Xu H, Shen Y, Kaur P et al (2010) Blood-brain barrier breakdown and repair by Src after thrombin-induced injury. Ann Neurol 67:526–533PubMedCentralPubMed
21.
Keep RF, Hua Y, Xi G (2012) Intracerebral haemorrhage: mechanisms of injury and therapeutic targets. Lancet Neurol 11:720–731PubMed
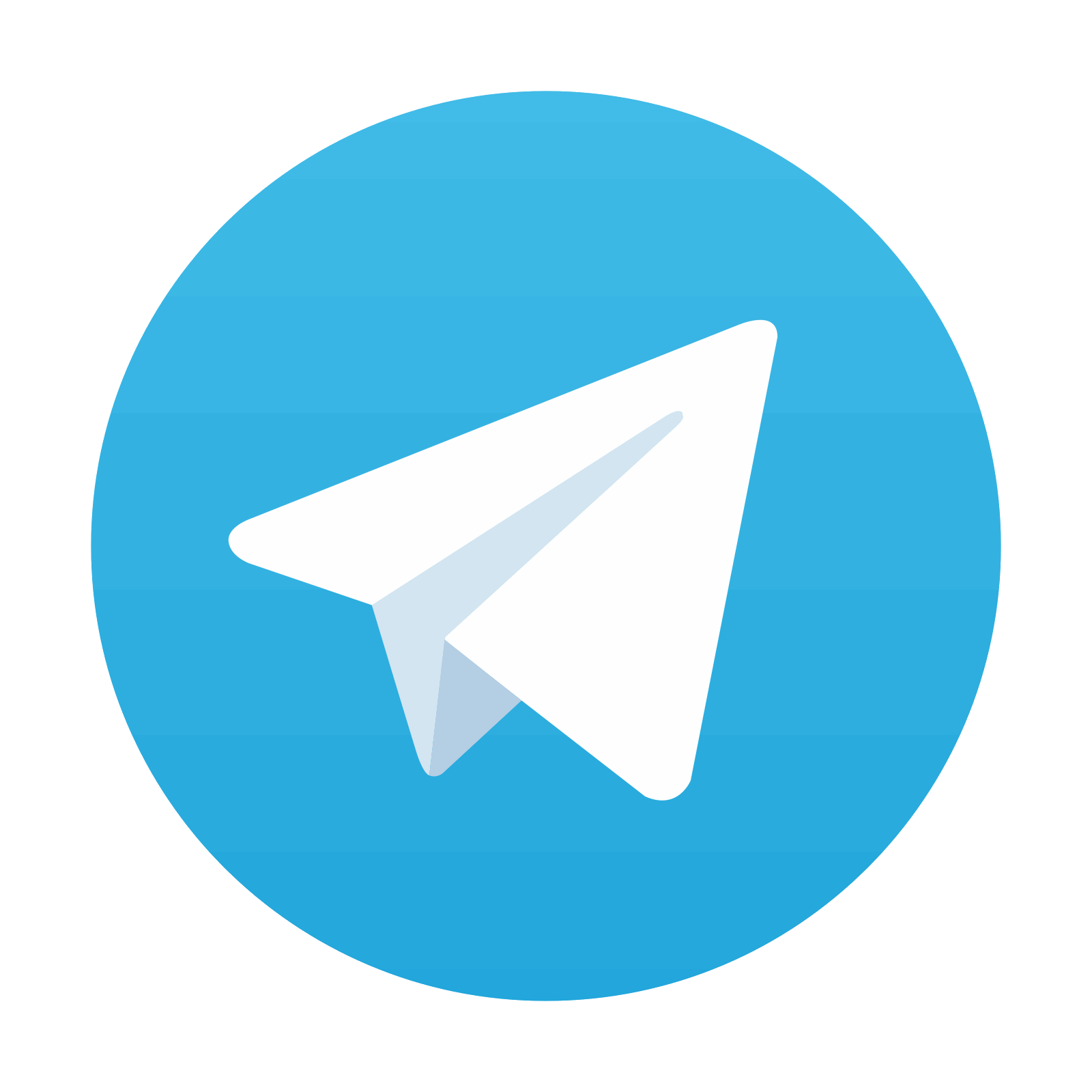
Stay updated, free articles. Join our Telegram channel

Full access? Get Clinical Tree
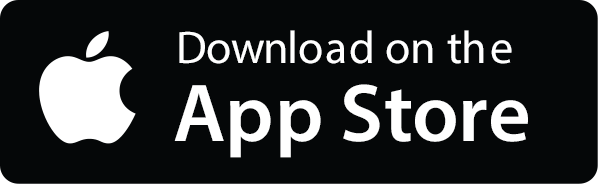
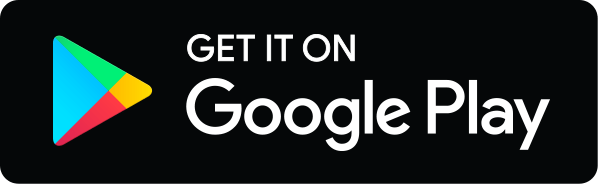