Patients choose a number from 1 to 7 that shows their degree of agreement with every statement, where 1 indicates strongly disagree and 7 indicates strongly agree
• My motivation is lower when I am fatigued
• Exercise brings on my fatigue
• I am easily fatigued
• Fatigue interferes with my physical functioning
• Fatigue causes frequent problems for me
• My fatigue prevents sustained physical functioning
• Fatigue interferes with carrying out certain duties and responsibilities
• Fatigue is among my three most disabling symptoms
• Fatigue interferes with my work, family, or social life
This chapter focuses on the causes and management of fatigue in primary sleep, neurological, and general medical and psychiatric disorders, with a brief overview of the proposed mechanism (the neurophysiological basis) of fatigue.
Identifying the Sites of Fatigue
Classification of fatigue as being of peripheral or central origin has aided the development of neurophysiological models [9]. Both peripheral and central fatigue can be demonstrated using models that measure the force generated by muscle during maximum voluntary contraction (MVC) (Fig. 42.1).


Fig. 42.1
Peripheral fatigue can be studied by measuring the maximum voluntary contraction (MVC) of muscle as recorded by a force transducer. Note the normal linear decrease in force generated is attributable to peripheral fatigue. Superimposed tetanic stimulation (denoted by the arrows) is used to detect the presence of central fatigue. Absence of significant twitch amplitude (A) suggests the absence of central activation failure (CAF), whereas a large twitch amplitude (B) suggests the presence of CAF. A pre-MVC tetanic contraction (C) and post-MVC tetanic contraction (D) are compared. The lower amplitude and slower relaxation phase of the post-MVC contraction is suggestive of the development of peripheral fatigue. (Reprinted with permission from Bhat and Chokroverty [44] and modified with permission from Zwarts et al. [11]
Peripheral fatigue occurs as a result of failure of the motor unit (the anterior horn cell, the motor axon, the neuromuscular junction, and the muscle fibers innervated by this). The recording and comparison of force generated by electrical stimulation of muscle before and after exercise helps identify peripheral fatigue. Typically, after a pre-exercise electrical stimulation, the subject performs a maximum voluntary contraction (MVC) for a pre-determined period of time, generally 30–60 s, which is measured with a force transducer. A subsequent post-exercise electrical stimulation is then performed, and the force generated is compared to the pre-exercise tracing. Lower amplitudes of the waveform and slower relaxation phases as compared with pre-exercise findings are characteristic of peripheral fatigue [10, 11]. On the other hand, central fatigue is generated in the central nervous system (CNS), namely the brain, spinal cord and descending central motor pathways, or at unidentified “pre-motor cortex” sites. This is assessed by measuring central activation failure (CAF), which suggests suboptimal CNS output to the motor unit. CAF can be measured by analyzing the force generated during MVC and providing superimposed electrical stimulation. The resulting twitch interpolation is then analyzed; the absence of significant change in twitch amplitude suggests full voluntary contraction and no central fatigue, whereas a large superimposed twitch amplitude suggests significant CAF. Several studies using transcranial magnetic stimulation (TMS) in patients with CNS lesions support the concept of a supraspinal site of fatigue in these conditions [12–14], and some authors have proposed a focal reduction in cortical excitability following a fatiguing motor task as the basis for such central fatigue [15, 16].
The localization of the seat of central fatigue remains elusive, and many diverse areas influencing motor output, such as the prefrontal cortex, multiple subcortical areas including the hypothalamus, the brainstem, the cerebellum, and neuronal systems subserving arousal and attention (i.e., ascending reticular activating system and the limbic system) have been investigated in this regard. Neuroimaging has not been able to identify a single anatomical substrate correlating with fatigue [17–20]. Functional magnetic resonance imaging (fMRI) studies in patients with fatigue have shown greater motor task-related activation of the contralateral cingulate area and more diffuse cervical cord recruitment [21–23] compared to controls, whereas positron emission tomography (PET) scans in patients with fatigue have demonstrated cerebral hypometabolism [24]. The clinical significance of these early findings remains unclear, and most patients with fatigue likely have involvement of multiple central and peripheral areas.
Cognitive or mental fatigue, defined as a failure to endure sustained mental tasks, a deficit of self-motivation, and overall debilitation not attributable to motor weakness, may occur with or without concomitant physical fatigue and is more challenging to identify, measure, and localize than physical fatigue. While a recent report suggested that the posterior hypothalamus may be involved in cognitive fatigue [25], this finding has yet to be replicated by other studies. Similarly, while several authors have proposed various cognitive and motor-task processing parameters to measure cognitive fatigue, large-scale validation studies are lacking [26–28].
Fatigue in Specific Conditions
Chronic Fatigue Syndrome (see also Chapter 47)
Chronic fatigue syndrome (CFS), also known as myalgic encephalomyelitis, is a poorly understood, ill-defined and yet potentially debilitating condition characterized by severe chronic fatigue lasting a minimum of six months and usually accompanied by myriad nonspecific systemic symptoms. Its full impact is difficult to estimate, with prevalence reports varying greatly from 0.002 to 11.3 % in primary care practices [29]. Most reports indicate that it is more common in women than in men, minority groups, and lower socioeconomic and educational classes, and is often comorbid with psychiatric and personality disorders [30, 31]. Our understanding about this condition is constantly evolving, and at present there is no uniform agreement about the criteria for diagnosis of CFS; while the Center for Disease Control (CDC) diagnostic criteria (Table 42.2) are generally widely accepted, they are limited by being highly dependent on patient self-reporting. The US Institute of Medicine recently recommended that the disorder be renamed “systemic exercise intolerance disease” and has proposed new diagnostic criteria, including three core symptoms that must be present: Impaired day-to-day functioning because of fatigue, malaise after exertion (physical, cognitive, or emotional) and unrefreshing sleep, and at least one of the following two additional symptoms: cognitive impairment or orthostatic intolerance [32]. This proposal awaits acceptance by the medical community at large.
Table 42.2
Center for Disease Control (CDC) criteria for the diagnosis of chronic fatigue syndrome (CFS)
In order to be diagnosed with chronic fatigue syndrome, a patient must satisfy two criteria: |
1. Have severe chronic fatigue for at least six months or longer with other known medical conditions (whose manifestation includes fatigue) excluded by clinical diagnosis and 2. Concurrently have four or more of the following symptoms: • post-exertional malaise • impaired memory or concentration • unrefreshing sleep • muscle pain • multi-joint pain without redness or swelling • tender cervical or axillary lymph nodes • sore throat • headache |
Attempts to identify the etiology of CFS have been futile. A post-viral etiology has long been proposed, and many patients complain of relatively acute onset of fatiguing symptomatology after a flu-like illness, but no organism has been consistently identified [33]. A few early studies provided some evidence for both central and peripheral immunological dysfunction in CFS [34–36], but more research is necessary to determine the clinical significance of these preliminary results [37]. The anatomical site of dysfunction in CFS is similarly uncertain. Numerous neurophysiological studies have failed to establish a peripheral cause of fatigue in CFS [38]. While studies have shown that there is a delay in information processing speed in patients and central dysfunction with CFS [39], MRI and single-photon emission computerized tomography studies have not identified consistent structural or functional markers [40].
Nearly half of the patients with CFS have a coexistent primary sleep disorder (mainly obstructive sleep apnea syndrome [OSA], as well as chronic insomnia and periodic leg movements during sleep [PLMS]), and 45 % have a coexistent psychiatric disorder (mostly mood and anxiety disorders) [41]. The diagnosis of either a sleep or psychiatric disorder, however, does not rule out coexistent CFS [42]. While sleep complaints such as unrefreshing sleep, EDS, and insomnia are common in patients with CFS, objective sleep architectural abnormalities are usually not demonstrable on polysomnography [43].
The optimal treatment of CFS is unclear. Immunological treatments (e.g., high-dose glucocorticoids and intravenous immunoglobulin [IVIG] therapy) have not been found to be beneficial [44]. Similarly, evidence for benefit from stimulants, antidepressants, and wakefulness-promoting agents (e.g., modafinil) is mixed [45]. Several studies, however, have consistently shown the superiority of nonpharmacological interventions in CFS, such as cognitive behavioral therapy (CBT) and low impact, graded aerobic exercise [46].
Fatigue in Primary Sleep Disorders
One of the unique challenges in treating patients with primary sleep disorders is distinguishing between fatigue and EDS [47]. Both symptoms are common bedfellows; nevertheless, as stated before, the distinction is important, because the two symptoms warrant different investigations and management strategies. The practitioner must help the patient to distinguish between fatigue and EDS based on historical clues. Several validated questionnaires, however, are available, aiding the distinction, such as the Epworth Sleepiness Scale (ESS) for EDS and the FSS for fatigue.
Most patients with untreated primary sleep disorders experience fatigue. Younger age, female gender, and a high number of awakenings and arousals have been shown to be predictive characteristics for fatigue [48]. Fatigue occurs in up to 42 % of patients OSA [49] and improves with continuous positive airway pressure (CPAP) treatment [50]. While fatigue in patients with OSA is related to the presence of depressive symptoms [51], it has not been found to correlate with the severity of OSA or with objective measures of EDS (such as multiple sleep latency testing [MSLT]), demonstrating once again that sleepiness and fatigue are distinct symptoms [52]. Similarly, although fatigue occurs in up to 63 % of patients with narcolepsy [53], it does not correlate with the degree of EDS; however, both symptoms respond to treatment with modafinil [54]. Both restless legs syndrome (RLS, recently renamed Willis-Ekbom disease [WED]) and PLMS have been found to correlate with sleep disruption and fatigue [55]. Circadian rhythm disorders, especially shift work disorder, are associated with both EDS and fatigue, independent of mood disorders [56], possibly related to lower morning serum cortisol levels [57]. Insomnia, either as a primary disorder or as a symptom of another sleep disorder or medical condition, is commonly associated with fatigue. Nonpharmacological interventions, such as CBT and exercise programs, improve sleep quality as well as fatigue-related symptoms [58].
Fatigue in Neurological Disorders
Fatigue in Peripheral Nervous System (PNS) Disorders
Fatigue in Neuromuscular Junction Disorders
Neuromuscular junctional disorders are characterized by the failure of transmission across the neuromuscular junction and may be presynaptic (e.g., Lambert-Eaton myasthenic syndrome) or postsynaptic (e.g., myasthenia gravis). This results in muscle fatigue of peripheral origin. However, patients with myasthenia gravis experience additional cognitive fatigue following completion of demanding cognitive work, suggesting a component of central fatigue [59]. Supplementation of Vitamin D may benefit fatigue in patients with myasthenia gravis showing Vitamin D deficiency [60].
Fatigue in Neuropathies and Myopathies
Chronic, persistent fatigue occurs in many patients with acquired immune-mediated neuropathies (e.g., Guillain–Barre syndrome, chronic inflammatory demyelinating polyradiculoneuropathy, and monoclonal gammopathies). Some authors have suggested that fatigue in these patients is related to axonal loss [61], but neurophysiological studies of the peripheral nerves are often normal, suggesting that central mechanisms are at play [62]. Medically supervised home exercise programs may be beneficial in these patients [63]. Modafinil has shown some benefit in alleviating fatigue in patients with hereditary motor sensory neuropathy type 1 (also known as Charcot–Marie–Tooth Disease-1), the most common inherited neuropathy [64]. Metabolic myopathies are characterized by the occurrence of “exercise intolerance,” cramping, painful contractions, and myalgias after exercise, while being asymptomatic at rest. This exercise intolerance may occur in the absence of motor weakness or abnormal physical findings, and may be perceived as fatigue by patients. Genetic testing is often required for diagnosis. Similarly, patients with inherited channelopathies (hypokalemic and hyperkalemic periodic paralysis, myotonia congenita, paramyotonia congenita, and Andersen–Tawil syndrome) present with paroxysmal attacks of periodic generalized weakness as well as muscle fatigability brought on by exposure to cold, carbohydrate-rich meals, exercise or rest, depending on the exact disorder. Except for the hypokalemic periodic paralysis, these patients also have myotonia on EMG and can be diagnosed by genetic testing. Muscle weakness in these disorders may last from a few minutes to a few hours during each attack, with a normal neurological examination finding including normal strength testing in between the attacks. The symptoms are often treated with acetazolamide, although the exact mechanism of action of this agent in these conditions is unknown [44]. Fatigue has also been described in a large number of patients with a variety of inherited myopathies such as facioscapulohumeral dystrophy and myotonic dystrophy [65]. Both fatigue and excessive daytime sleepiness are common with myotonic dystrophy type 1 (DM1), most likely due to prominent sleep dysfunction that accompanies sleep-disordered breathing, numerous microarousals, and PLMS. DM1 patients have short sleep latencies on MSLT as well as sleep onset REM periods (SOREMPs), suggesting a narcoleptic phenotype [66].
Fatigue in CNS Disorders
Fatigue in Anterior Horn Cell Disorders
Fatigue is very common in disorders of the motor unit and pyramidal cells. In patients with amyotrophic lateral sclerosis (ALS), both central and peripheral fatigue seem to be present. Patients with ALS show evidence of CAF on MVC studies, as well as less intramuscular phosphocreatine depletion and less fatigue of stimulated tetanic force during exercise compared to controls, suggesting that central fatigue plays a major role in this condition [67]. Peripheral fatigue has been demonstrated in muscles that show no evidence of denervation-related injury, suggesting that motor weakness and fatigue in ALS are distinct entities. Maladaptation of cortical processes related to degeneration of inhibitory GABAergic intracortical circuits is a feature of ALS that significantly correlates with the development of fatigue and weakness [68]. Sleep disruption is a contributory factor as well [69].
Fatigue occurs in over three quarters of patients with post-polio syndrome (PPS); young patients with PPS with shorter polio duration, more pain, and higher body mass index tend to be more fatigued and have a lower quality of life [70]. Both central and peripheral causes seem to be at play, with neuromuscular junction failure as well as defects of attention and information processing having been demonstrated [71, 72]. Neuroimaging studies have also shown that more than half of the patients with PPS-related fatigue have CNS white matter lesions [73]. EDS (causing secondary fatigue) may also result from sleep fragmentation, PLMS, OSA, hypoventilation, or a combination of these factors [74]. Treatment is unsatisfactory; amantadine, modafinil, and IVIG have all been studied, but no consistent benefit has been found [75]. The role of CBT and exercise programs is currently being investigated [76].
Fatigue in Multiple Sclerosis
Fatigue is a cardinal feature of multiple sclerosis (MS), reported by over 80 % of patients [47], but its etiology remains a matter of debate. Hypothalamic dysfunction, hypothalmo-pituitary axis hyperactivity, elevated adrenocortoctrophic hormone levels, lower waking cortisol levels and dopamine imbalance, and increased circulating proinflammatory cytokines have been all been implicated in causing fatigue in MS [77–81]. While certain agents used to treat MS, in particular interferons, may themselves worsen fatigue, glatiramer acetate and natalizumab have shown some efficacy in alleviating MS-related fatigue [82, 83]. The role of sleep dysfunction in MS patients with fatigue is being increasingly recognized. Insomnia, OSA, RLS, and PLMS are all very common in patients with MS and, when present, are associated with fatigue. Treating comorbid sleep disorders in MS patients improves fatigue [84]. Management of fatigue in MS has proven to be challenging. Pharmacological agents such as modafinil and amantadine have been tried with varying success. As with fatigue in general, MS-related fatigue improves with nonpharmacological measures (e.g., exercise programs and CBT) [85].
Fatigue in Parkinson’s Disease
A majority of patients with Parkinson’s disease (PD) complain of sleep disturbances and fatigue, which is associated with several motor and nonmotor symptoms [86]. Positron emission tomography (PET) scan findings in PD suggested that fatigue in this disorder is associated with reduced serotonergic function in the basal ganglia and limbic structures, and probably also dopaminergic dysfunction in the insular cortex [87]. Sleep dysfunction is common in PD (including insomnia, circadian rhythm abnormalities, RLS/PLMS, nocturnal bradykinesia and discomfort, sleep fragmentation, comorbid sleep apnea, and REM behavior disorder), and both sleep disorder and depression appear to be independent predictors of PD-related fatigue and EDS [88]. Fatigue may respond to treatment of comorbid mood disorders, levodopa therapy [89] as well as nonpharmacological interventions such as (e.g., CBT and exercise programs) [90].
Post-stroke Fatigue
Post-stroke fatigue affects more than half of the patients who suffer strokes and is associated with poor long-term functional outcome and physical health [91]. While post-stroke fatigue is often accompanied by depression, deficits in processing speed and memory appear to be independent of depressive symptoms [92]. It has been proposed that post-stroke fatigue is related to decreased motor cortex excitability [16], and serum glucose and homocysteine levels may play a role [93]. No neuroimaging findings predict post-stroke fatigue, and it does not appear to correlate with degree of neurological deficit. Younger patients with infratentorial infarctions and concomitant post-stroke depression appear to be most at risk for developing post-stroke fatigue [94]. There are no guidelines for treating post-stroke fatigue. Antidepressants, though commonly prescribed to post-stroke patients, have not been conclusively proven to be beneficial, and the role of CBT, exercise, and medications like modafinil and amantadine remains uncertain in this setting [95, 96].
Fatigue in Traumatic Brain Injury
Traumatic brain injury (TBI) results in a variety of long-term consequences, including sleep complaints such as insomnia and daytime sleepiness, cognitive impairment, mood disorders and fatigue, with the degree of initial injury being a poor predictor of the severity of post-TBI impairment [97, 98]. Fatigue, in particular, is present in nearly half of all patients post-TBI, does not appear to significantly improve with time, and seems to be independent of depression or EDS [99]. The natural history of fatigue in TBI remains unclear, but CBT, lifestyle modification, pharmacologic treatments with modafinil and melatonin, and light therapy have been suggested as possible interventional strategies [100].
Fatigue in General Medical Conditions
Chronic fatigue occurs in a wide variety of medical conditions such as anemia, autoimmune disorders (e.g., systemic lupus erythematosus [SLE], sarcoidosis), chronic infectious diseases such as HIV and Lyme’s disease, cardiopulmonary disorders (chronic obstructive pulmonary disease [COPD], congestive heart failure [CHF], interstitial lung disease), rheumatological disorders like fibromyalgia, and cancer. Endocrinological disorders such as hypothyroidism and hypothalamo-pituitary axis dysfunction are other causes of chronic fatigue. In addition, it may be a side effect of a wide variety of medications, most commonly beta-blockers, anxiolytics (e.g., benzodiazepines and barbiturates), antiepileptics (valproic acid, carbamazepine, and levetiracetam), antipsychotics, dopaminergics, proton pump inhibitors, chemotherapeutic agents, and beta interferons.
Fatigue in Cardiopulmonary Disease
Fatigue in COPD
The prevalence of daily fatigue in COPD has been reported as high as 80 %, and nearly half of COPD patients describe it as their most disabling symptom, affecting cognitive, physical, and psychosocial well-being and quality of life. It worsens with underlying disease severity and predicts higher hospitalization rates [101, 102]. The etiology of fatigue in COPD is incompletely understood, but is most likely multifactorial. Exercise intolerance in patients with COPD has been shown to be predictive of fatigue [103]. In addition, coexistent mood disorders and sleep disorders such as RLS [104] and sleep-disordered breathing (in particular the combination of COPD and OSA, known as the “overlap syndrome,” a common cause of severe nocturnal hypoxemia, sleep disruption, and subsequent EDS) increase the likelihood of fatigue. Severe COPD is also associated with complaints of insomnia and nonrestorative sleep [105]. Treatment of fatigue in COPD is closely linked to the improvement of pulmonary status and comorbid sleep and mood dysfunction. Multidimensional programs that include pharmacotherapy to improve lung function, pulmonary rehabilitation, and CBT for insomnia have shown promising results [106].
Fatigue in Heart Failure
Fatigue is an important symptom in patients with heart failure (HF) including approximating 70–90 % of all patients which adds to the overall debilitation caused by orthopnea, peripheral edema, nocturnal cough, and exertional dyspnea. Fatigue is indicative of a poor prognosis in HF, but a consistent relationship between fatigue and degree of cardiac dysfunction has not been demonstrated [107, 108]. The proposed causes of fatigue in HF include impaired peripheral perfusion during exercise, reduced oxidative capacity of skeletal muscle, impaired muscle strength, and possibly reflex mechanisms associated with alterations in the metabolism of skeletal muscle. However, fatigue may persist despite optimization of cardiac output [109]. Comorbid sleep disorders contribute to fatigue in HF, with sleep-disordered breathing (including primary central apneas and Cheyne–Stokes respirations), nocturia, and insomnia being the most common. In addition, pharmacotherapy of HF, especially with beta-blockers, has been linked to fatigue. Fatigue and depression occur independently in HF [110]. Nonpharmacological interventions such as individualized exercise training programs for all patients with stable CHF may combat fatigue [111].
Fatigue in Chronic Renal Failure
The debilitating fatigue experienced by patients with chronic renal failure and end-stage renal disease (ESRD) is likely due to multiple chronic metabolic and physical derangements, such as anemia, cachexia, and abnormal calcium and phosphate metabolism. Nearly half of the patients on hemodialysis complain of major fatigue, often associated with depression and poor sleep quality [112]. Primary sleep disorders such as OSA and RLS/PLMS may lead to both insomnia and EDS, worsening fatigue [113]. Optimal treatment of fatigue in ESRD patients on hemodialysis is unclear, but may include strengthening of social support, exercise programs, erythropoietin, and l-carnitine supplementation [114].
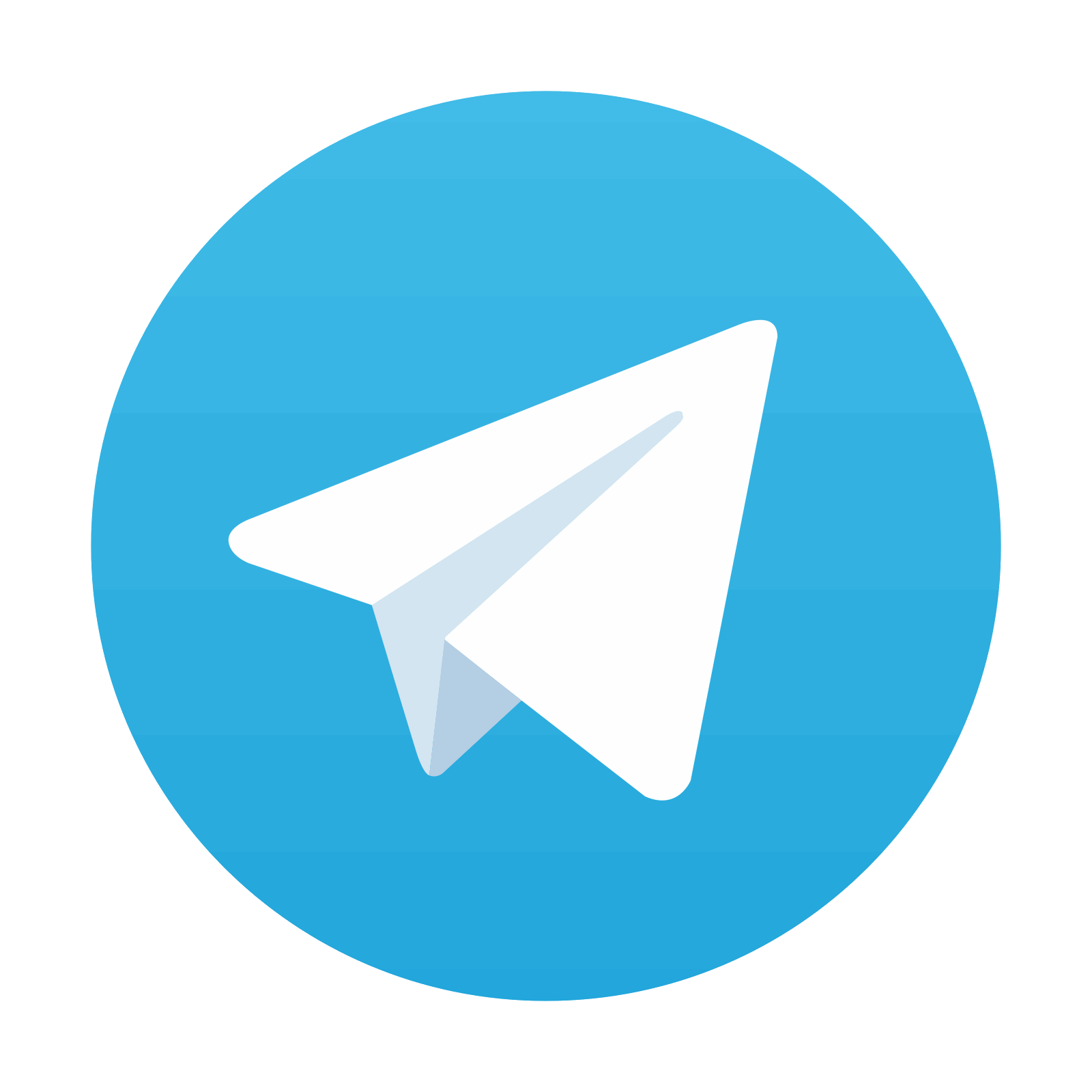
Stay updated, free articles. Join our Telegram channel

Full access? Get Clinical Tree
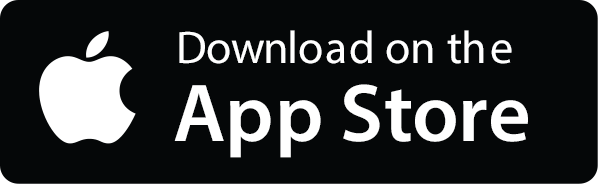
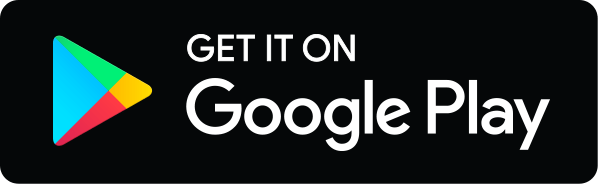