A 46-year-old woman is brought back to the neurologic intensive care unit (NeuroICU) after undergoing coiling of a right middle cerebral artery aneurysm that had ruptured the previous day. On examination, she requires mild sternal rub to remain awake and is able to follow simple, one-step commands. There are no cranial nerve abnormalities, but a mild left pronator drift is noted. Overall, examination is unchanged from presentation. While in the operating room, a left subclavian central line and radial arterial line is placed along with a right external ventricular drain. On posthemorrhage day 5 (postoperative day 4), she develops a fever with a temperature of 39°C.
Fever after brain injury independently worsens outcome. One of the important mechanisms is by exacerbating inflammatory cascades. Postinjury elevations in temperature have been shown to increase inflammatory processes, including elevations in proinflammatory cytokines, the increased accumulation of polymorphonuclear leukocytes in injured tissue.1 Vascular and inflammatory cascades appear to be extremely sensitive to mild elevations in temperature following central nervous system (CNS) injury.
Several studies also suggest that one of the key impacts of fever is an increase in neuronal excitotoxicity. Elevations in temperature have been reported to increase neurotransmitter release, accelerate free-radical production, increase intracellular glutamate concentrations, and potentiate the sensitivity of neurons to excitotoxic injury.1 In an experimental microdialysis study of focal ischemia, glutamate release was significantly higher in hyperthermic than normothermic rats, indicating the importance of focal brain temperature on neurotransmitter release.2 Other investigators have observed increases in cellular depolarization in the ischemic penumbra surrounding damaged neuronal tissue, increased neural intracellular acidosis, and inhibition of enzymatic protein kinases, which are responsible for synaptic transmission and cytoskeletal function in relation to elevations in core body temperature.3 At the molecular level, elevations in temperature have been shown to enhance the expression of heat-shock proteins, as well as receptor expression associated with glutamate neurotransmission.4 Fever affects cerebral hemodynamics, and cerebral blood flow (CBF) velocity and pulsatility index has been shown to increase in parallel with rising temperatures.5 Experimental mild hyperthermia has been shown to increase contusion areas and volumes and most severe loss of neuronal nuclear (NeuN)-positive cells in animal models with mild traumatic brain injury (TBI).6
Experimental and clinical studies have suggested that fever can also directly induce nervous system injury.7 In these circumstances, it is usually a temperature > 40°C for extended periods that produces abnormalities in the blood-brain barrier, as well as profound cardiovascular, metabolic, and hemodynamic dysfunction. The hypothalamus is a key center for thermoregulation, and damage to this structure can result in hyperthermia. Thus, mechanisms underlying hyperthermia-induced pathophysiology and secondary hyperthermia following CNS injury are similar.
A meta-analysis of all brain injury types found that fever was consistently associated with mortality and worse outcomes across multiple outcome measures of morbidity.8 However, several important questions regarding the impact of timing and duration of fever on the outcome remain unanswered. Is it the fever seen within the first 24 hours or within the first 10 days the most influential on outcome? Which is more important in influencing outcome: the number of febrile episodes or the overall burden of temperatures > 37°C in the first week? Both clinical and experimental evidence indicate that each type of injury may have an optimal time window for fever control.
The new onset of fever should trigger a careful diagnostic evaluation to find a source of infection. It is important to be familiar with the patient’s history, with particular attention being paid to predisposing causes of fever. For example, patients presenting in coma who require emergent endotracheal intubation are likely at a higher risk for developing pneumonia from aspiration. In addition to auscultation of the lung fields and careful abdominal examination, particular attention should be paid to any surgical wounds or skin ulcerations as well as for cerebrospinal fluid (CSF) leaks (otorrhea or rhinorrhea) in patients who have undergone a craniotomy. Review of chest radiographs should focus on any evidence of new infiltrates or effusions. Initial laboratory tests should focus on peripheral white blood cells (WBCs) and cultures of blood, urine, sputum, and CSF in patients with an external ventricular or lumbar drain. If the patient is endotracheally intubated or has a tracheotomy, obtain a sample of sputum for Gram stain either by blind suctioning or bronchoalveolar lavage. Central venous catheters that have been in place for > 96 hours should be removed, and the tip should be submitted for semiquantitative microbiology. In patients receiving antibiotics for > 3 days, a stool sample should be analyzed for the presence of Clostridium difficile toxin (Figure 21-1).
Endogenous pyrogens released by leukocytes in response to infection, drugs, blood products, or other stimuli cause fever by stimulating cerebral prostaglandin E synthesis and as a result raise the hypothalamic temperature set point.9 Antipyretic agents including acetaminophen, aspirin, and other nonsteroidal anti-inflammatory drugs (NSAIDs) are believed to block this process by inhibiting cyclooxygenase-mediated prostaglandin synthesis in the brain, resulting in a lowering of the hypothalamic set point. This activates the body’s two principal mechanisms for heat dissipation: vasodilation and sweating.9 The effectiveness of antipyretic agents is tightly linked to conditions where thermoregulation is intact. Therefore, they are more likely to be ineffective in brain-injured patients with impaired thermoregulatory mechanisms. Corticosteroids also have antipyretic properties but are not used clinically to treat fever because of their side effects.
Whether acetaminophen alone is more effective than placebo for treating fever in adult ICU patients is still unclear. The majority of studies have been conducted in the pediatric population, where weight-adjusted doses have been shown to be effective in reducing fever. In the adult neurocritical care population, acetaminophen has been most widely studied in an attempt to maintain normothermia in patients with acute stroke. Koennecke and Leistner10 showed that treatment with acetaminophen in a daily dose of 4000 mg resulted in a substantial reduction of the proportion of patients with body temperatures > 37.5°C (the amount of temperature reduction was not reported). Kasner et al11 observed a difference of 0.2°C in body temperature in favor of treatment with acetaminophen (approximately 4 g/d) compared with placebo in patients with hemorrhagic or ischemic stroke, although not statistically significant. Two recent phase II studies have demonstrated that perhaps a higher dose of acetaminophen (6000 mg/d) is more effective in maintaining normothermia and preventing fever.12 In a recent trial, intravenous (IV) acetaminophen was found as safe and effective in reducing endotoxin-induced fever as PO acetaminophen, but with an earlier onset of action.13 A phase III trial comparing IV paracetamol to placebo in the treatment of fever in critically ill adults with known or suspected infection is currently ongoing.14 However, IV acetaminophen, given in antipyretic doses, can cause a significant decrease in blood pressure in febrile critically ill patients, and this side effect should be considered when using it in the neurocritical care population.15
Ibuprofen has been widely studied in the pediatric population, with equivalent or superior efficacy compared with acetaminophen. However, only one randomized, controlled study has been conducted in adult patients with brain injury, which demonstrated that ibuprofen (2400 mg/d) was not shown to be better than acetaminophen or placebo in maintaining normothermia after ischemic stroke.12 A small randomized study of a continuous infusion of diclofenac sodium (0.04 mg/kg/h) was found to be effective in reducing the burden of fever and number of febrile events in critically injured patients with TBI and subarachnoid hemorrhage (SAH).16 Another trial has shown that IV ibuprofen (400 mg q4h) was effective in lowering temperature to normal and maintaining this over the first 24 hours of dosing compared with placebo in both critically ill and non-critically ill groups.17 There were no clinically significant differences in any safety parameter including renal function or bleeding throughout the 28-day follow-up period.
External cooling reduces body temperature by promoting heat loss without affecting the hypothalamic set point. Four modes of heat transfer constitute the basis of interventions to promote heat loss: (1) evaporation (eg, water sprays or sponge baths); (2) conduction (eg, ice packs, water-circulating cooling blankets, immersion); (3) convection (eg, fans, air-circulating cooling blankets); and (4) radiation (ie, exposure of the skin).18 In patients with temperature elevations caused by impaired thermoregulation, such as what occurs after brain injury, antipyretic agents are usually ineffective, and temperature reduction may only be achieved by external or internal cooling. However, external cooling can result in reflex shivering and vasoconstriction as the body attempts to generate heat and counteract the cooling process.
Few controlled studies have evaluated the efficacy of external cooling interventions for lowering body temperature in humans. Previous experimental studies have shown that the combination of evaporative and convection cooling, with water sprays or sponging and forced airflow, is more effective than conduction cooling or either method alone for reducing temperature in non–brain-injured patients with hyperthermia.19 In a study of febrile neurocritical care patients treated with acetaminophen, air-blanket cooling had a small benefit that did not reach statistical significance.20
Water-circulating cooling blankets, a form of conductive cooling, are the most commonly used treatment for acetaminophen-refractory fever in critically ill adults. However, there are few data regarding their efficacy. Two small controlled studies have evaluated external cooling in adult ICU patients. One study compared the use of acetaminophen alone with tepid water sponging or with a water-circulating cooling blanket in febrile neurologic patients and found no difference between the three treatments.21 Another study of febrile patients under sedation, analgesia, and mechanical ventilation found that ice-water sponging was superior to two IV NSAIDs22 in a nonrandomized crossover study. A large observational study found no difference in the mean cooling rate in febrile ICU patients treated with or without water-circulating cooling blankets.23 A feature commonly found with water-circulating blankets is the wide fluctuations in temperature that occur, with temperature overshoot being very common.
Recent engineering advancements have introduced a new set of surface-cooling blankets that are much more efficient at achieving and maintaining normothermia (Figure 21-2). Each system works by utilizing tightly wrapped pads that circulate cold water to promote conductive heat loss. In a randomized controlled trial, 53 neurologically injured patients who had a fever (temperature ≥ 38.3°C) for at least 2 hours after the administration of 650 mg of acetaminophen were randomized to treatment with an advanced cooling blanket system or a conventional water-circulating cooling blanket. Despite having a slightly higher baseline mean temperature, patients treated with the advanced system experienced a 75% reduction in fever burden and became normothermic faster than the control group, despite a significantly higher rate of shivering.24
Figure 21-2.
Food and Drug Administration-approved temperature-modulation devices. A. Gaymar surface-cooling device using wrap-around pads. (Used with permission from Stryker Corporation, Kalamazoo, MI). B. Cincinnati Sub-Zero surface-cooling device using a cool blanket. (Used with permission from Cincinnati Sub-Zero Products LLC, a Gentherm Company). C. Medivance Arctic Sun, surface temperature modulation using conductive gel pads. (Used with permission. Copyright © 2017 C.R. Bard, Inc. Bard is a registered trademark of C.R. Bard, Inc.). D. ZOLL STx™ surface pad system for cooling and warming using thigh and vest pads. (Used with permission from ZOLL Medical Corporation). E. ZOLL Thermogard XP® temperature management system using balloon catheters. (Used with permission from ZOLL Medical Corporation).



Over the past few years, a number of intravascular devices have become available to lower body temperature (Figure 21-2). They all work to control fever by directly lowering the blood temperature with cooled saline, which circulates through balloons or channels around an intravascular catheter. Although there is no direct contact with the blood, the cold saline solution extracts heat from the blood, thus lowering body temperature.19
Infusion of 4°C normal saline is an appealing option because it is inexpensive and easy to administer in the critical care setting. The use of cold saline boluses has recently been studied and advocated for the induction of hypothermia in patients after cardiac arrest.25 A small case series also found the rapid infusion of large-volume cold saline to be a safe and effective method to achieve normothermia in select brain-injured patients.26 In addition to its rapid onset, the large volume of infusion can help offset the fluid imbalance that may be observed during the induction of hypothermia. Therefore, the administration of large-volume cold saline could be considered during the induction phase of fever control. Preclinical and preliminary clinical data suggest that intraarterial cold infusions may induce highly selective and rapid decrease in brain temperatures without significantly affecting body core temperatures, thus potentially diminishing systemic side effects of therapeutic temperature management.27 A transnasal evaporative cooling device that uses nasal catheters to spray an inert coolant (perfluorohexane) at a high flow rate into the nasal cavity has recently become available commercially. The device was shown to be effective in reducing brain temperatures by 1.2°C to 1.4°C in neurocritical patients.28,29 However, in a small trial, brain cooling was faster with cold infusions than with use of this device.30 More recently, a prototype of an esophageal cooling device was found effective in inducing hypothermia in a large animal model.31
The nurse administers 1000 mg of acetaminophen through the patient’s nasogastric tube. Cultures from blood, urine, sputum, and CSF are sent to the laboratory. All the lines are inspected and are noted not to appear infected. A decision is made to initiate aggressive fever control to maintain normothermia with an advanced temperature-modulating device.
Fever after SAH occurs in up to 70% of all patients in the first 10 days after SAH. In addition to disease severity and the amount of SAH present, the presence of intraventricular hemorrhage is a strong risk factor for the development of fever.32 Experimental models have demonstrated that the presence of even the smallest amount of blood within the CSF can induce fever.33
Clinically, the febrile response has been implicated in the development of cerebrovascular vasospasm after SAH.34 Fever can also exacerbate ischemic injury, worsens cerebral edema, and increase intracranial pressure (ICP).3,34 Numerous recent studies have found that after controlling for baseline predictors of poor outcome, fever is associated with an increased risk of death or severe disability, loss of independence in instrumental activities of daily living (IADL Scale), and cognitive impairment.8 In a case-control study, lowering fever burden over 14 days with therapeutic normothermia was independently associated with an improvement in 12-month outcomes.36 This study also found a higher rate of arrhythmias and hyperglycemia in the therapeutic normothermia group.
Therapeutic hypothermia for the first 12 to 24 hours after cardiac arrest has been adopted as a standard of care for survivors of cardiac arrest for more than a decade.37 However, the ongoing importance of controlling temperature and preventing fever after the initial 24 hours has not been well studied. Previously published studies indicated that the occurrence of fever in the first 72 hours after cardiac arrest was very common and was independently associated with poor outcome.38,39 In a more recent study, fever was present in 42% patients with cardiac arrest, with a postarrest median onset of 15 hours in patients not receiving therapeutic hypothermia and 36 hours in those who did.40 Fever was associated with worse outcomes in all patients, but with decreased survival only in those who did not receive therapeutic hypothermia. Other clinical evidence supports worsened neurologic outcomes with rebound pyrexia after therapeutic hypothermia post-cardiac arrest.41,42 A recent landmark trial found similar benefits of a targeted temperature of 36°C compared with currently popular targeted temperature goals of 33°C in survivors of out-of-hospital cardiac arrest.43 An active control of fever during the first 3 days was attempted in both groups with improved overall outcomes, thus supporting ongoing maintenance of fever control (therapeutic normothermia) after therapeutic hypothermia in survivors of cardiac arrest.
The incidence of fever in patients with spinal cord injury (SCI) is about 60% in acute care, with the most common cause being respiratory and urinary tract infections.44 However, similar to brain-injured patients, this population also has a high incidence of thermoregulatory problems, deep venous thrombosis, and fever of unknown etiology.45 Although less common, “quad fever” with an extreme elevation in body core temperature > 40.8°C from thermal dysregulation have been described with cervical or higher thoracic cord injuries.46 Experimental studies have shown that inducing hyperthermia immediately after cord injury is associated with increased tissue damage and worse outcomes in behavioral and histopathologic measures compared with normothermic conditions.1 Although robust clinical data are still lacking, few experimental studies and clinical case-series support use of therapeutic normothermia in selected cases.47,48
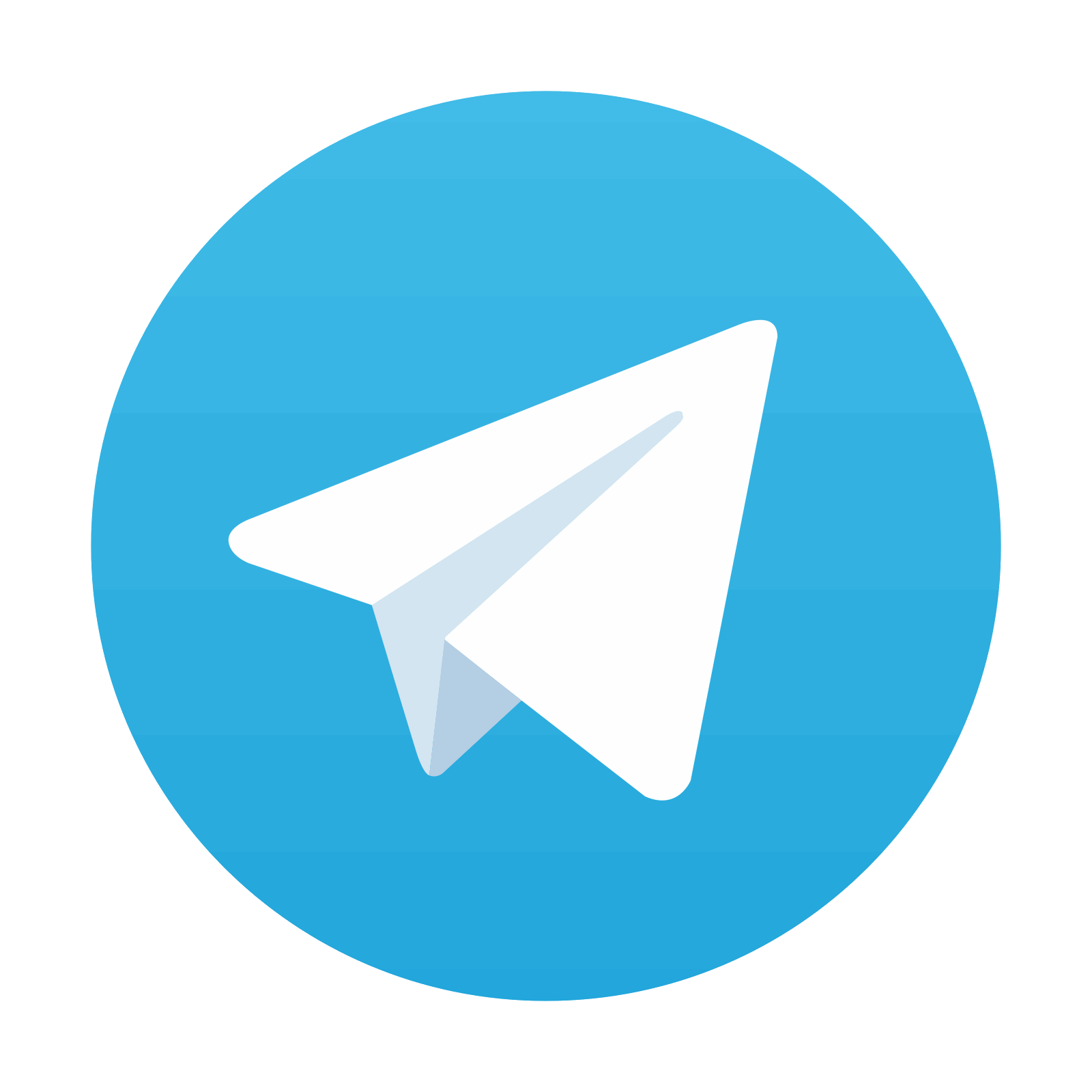
Stay updated, free articles. Join our Telegram channel

Full access? Get Clinical Tree
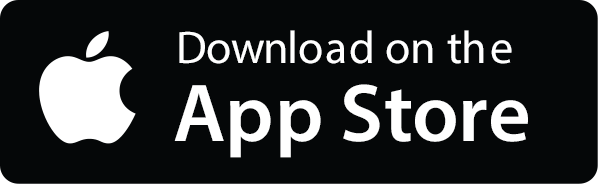
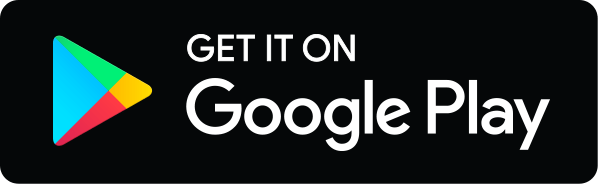