Flavor: Interaction Between the Chemical Senses
Summary
Both chemical senses, smell and taste, contribute enormously to flavor perception, but it has been shown that visual and trigeminal inputs also influence olfactory and gustatory perception. In terms of olfaction, orthonasal and retronasal perception differ: Retronasal thresholds are higher and perceived intensity of identical stimuli is reduced, suggesting different central processing, which has been confirmed on functional magnetic resonance imaging studies. Application of congruent olfactory and gustatory stimuli has been shown to produce superadditive central responses compared with unimodal stimulation alone. Moreover, simultaneous application of congruent odorants can increase the perceived intensity of tastants. Trigeminal and olfactory stimuli, both perceived intranasally, interact; and the trigeminal threshold is increased in patients with anosmia. Visual stimuli, if present as congruent visual cues while presenting odorants, can also enhance odorant processing. These interactions explain the complaints of patients suffering from an isolated olfactory loss who nevertheless complain about changes in the perception of trigeminal and gustatory stimuli as well.
Introduction
The chemical senses of taste and smell interact with each other during eating and drinking to produce flavor perceptions. In everyday life, it is very rare to sense taste without also sensing smell. This contrasts with how taste and smell are generally studied in the laboratory as independent modalities. It is also recognized that other factors influence flavor perception.1 This is illustrated by imagining a dinner in a nice restaurant with music and candlelight: Not only is the flavor of the food perceived, but also the visual and acoustic sensory inputs. These additional sensations contribute not only to the atmosphere, but also to the perception of flavor. However, although there is general agreement that many modalities contribute to flavor perception, there continues to be significant debate over just how many modalities should be included. Here, the core flavor modalities are defined as those sensations that arise, or appear to arise, from the mouth.2 These sensations include olfaction, gustation, and oral somatosensation. The goal of this chapter is to provide an overview of chemosensory integration in healthy subjects, and then in subjects with known chemosensory deficits.
Flavor Perception
Olfactory and gustatory inputs are the main contributors to flavor perception, alongside trigeminal inputs such as temperature and texture of the given food.3 Before looking at further details, one has to keep in mind that odorants are perceived in two ways: Orthonasally, before they enter the mouth; and retronasally, when odorants traverse the nasopharynx. To understand interactions in flavor perception and the important role of retronasal odorant perception in flavor perception, the main differences between orthonasal and retronasal perception will be briefly discussed.
Orthonasal versus Retronasal Olfactory Perception
The olfactory components of food are first sensed orthonasally, before the food is placed inside the mouth, and then retronasally, when the food enters the mouth ( Fig. 19.1 ). In both cases the odor molecules bind to receptors in the olfactory epithelium.4 In 1982, Rozin noted that olfaction is the only dual sense modality, in that it provides information about objects distal and proximal to the observer.5 He therefore suggested that olfaction should be considered as two distinct senses. It is nowadays known that odorants of the same concentration are perceived less intensely when applied retroansally.6 Moreover, retronasal thresholds are higher than orthonasal thresholds even in odorants identical in hedonics and concentration, thus supporting the idea that orthonasal and retronasal odorants are processed differently.7,8 Using functional magnetic resonance imaging (fMRI), Small and colleagues showed that the brain responds differently to the same food odor sensed orthonasally than retronasally.9 In a subsequent study using olfactory event-related potentials (OERPs), Hummel and Heilmann10 were able to demonstrate that OERP responses were larger when an odor unrelated to food was presented in an unusual site (i.e., retronasally) than when the same odor was presented at an orthonasal site. The result was the other way around for a food-related odor. Odorants were presented using an olfactometer and a tube close to the epipharynx, mimicking molecules coming from the epipharynx (see Fig. 19.2 ). In patients with endonasal polyps, retronasal function was found to be significantly better than orthonasal olfactory function compared with controls.11 Retronasal olfactory function was also found to be better than orthonasal function in patients with olfactory disorders because of other reasons, further supporting the different processing of orthonasal and retronasal stimuli.12 Performing an interesting experiment involving mechanical blocking of the anterior part of the olfactory cleft, Pfaar et al were able to demonstrate that orthonasal and retronasal perception differ.13 Further findings regarding orthonasal and retronasal olfaction will be discussed below within the flavor perception section.

Interaction Between Olfactory–Gustatory and Gustatory–Olfactory Systems
Almost two decades ago in monkeys, single-cell recording techniques identified single cells responding to both taste and smell in the orbitofrontal cortex,14 as well as in the insula/operculum.15 Interestingly, these bimodal cells in the orbitofrontal cortex of the macaque were selective for congruent stimuli: For example, a cell responding optimally to a sweet taste such as glucose also responded optimally to sweet-like odors rather than to odors related to salty food. Also in primates, bimodal cells have been identified that react to both gustatory and visual stimuli. As such techniques are not applicable in humans, it took the implementation of functional imaging techniques to gain further insight into multimodal processing in humans. Initial functional imaging work focused on the identification of primary and secondary olfactory and gustatory cortices. The piriform cortex and medial orbitofrontal cortex,16 caudal orbitofrontal cortex,17 and the anterior insula/frontal operculum18 have been identified. In all these studies, however, the brain’s reaction to isolated olfactory or gustatory stimuli was recorded. Using positron emission tomography, Small et al19 were the first to examine the brain’s reaction to simultaneous olfactory and gustatory stimuli. They were able to show that the evoked cerebral blood flow in primary gustatory and secondary gustatory and olfactory cortices decreased during simultaneous presentation of olfactory and gustatory stimuli compared with independent presentation of these stimuli alone. This decrease in response was later interpreted to reflect competition between the taste and smell systems, arising from the fact that the odors were delivered orthonasally by asking subjects to sniff, rather than retronasally, as would naturally occur during bimodal taste and odor stimulation during eating or drinking.8 In this study, olfactory or gustatory stimuli were not only applied “alone” but also in a “matched” and “mismatched” flavor condition (for example, sour taste and grapefruit smell as a matched condition; sweet taste and soy sauce odorant as a “mismatched” condition). In the “mismatched” condition, an increase in blood flow was observed in the left amygdaloid region and in the left and right basal forebrain. These results have been interpreted as confirmation of the function of the amygdala and basal forebrain in processing novel or unpleasant stimuli.19

This study was followed by others using fMRI techniques. Cerf-Ducastel et al20 tested the interaction of gustatory and lingual somatosensory perception at a cortical level in humans. Stimulating subjects with either pure gustatory stimuli or stimuli containing both gustatory and somatosensory qualities, they were able to demonstrate that gustatory and somatosensory lingual representation fields overlap hugely, as similar cortical areas (namely the insula, the Rolandic operculum [base of the pre- and post-central gyri], the frontal operculum, and the temporal operculum) were activated by both kinds of stimuli. Moreover, in 2001, Cerf-Ducastel and Murphy21 applied aqueous solutions of odorant intraorally, thus mimicking retronasal odorant perception. The observed activation pattern included all the brain regions usually activated when odorants were applied orthonasally, such as the piriform and orbitofrontal cortex, the hippocampal region, the amygdala, the insular lobe, the cingulate gyrus, and the cerebellum. In this study, however, no comparison with orthonasally applied odorants was made. A region in the lateral anterior part of the orbitofrontal cortex was identified in 2003 by de Araujo et al as the region activated by combined olfactory and taste stimuli, but not by unimodal stimuli alone.22 Odorants in this study were delivered in aqueous form, again to mimic retronasal stimulation in combination with taste stimuli, just as they were in another study by Small et al in 2004.23 Using familiar/congruent taste odor pairs and unfamiliar/incongruent taste odor pairs, as well as unimodal stimuli, the authors were able to show superadditive responses in the anterior cingulate cortex, the insula, the orbitofrontal cortex, and the posterior parietal cortex following stimulation with the congruent pair compared with unimodal stimulation alone.
In summary, these initial studies using intraorally applied aqueous stimuli in combination with taste stimuli to examine flavor perception were able to identify the orbitofrontal cortex, the insula, and the cingulate cortex as the main areas where odorants and tastants integrate. Moreover, it became obvious that the experimental set-up (namely the route of delivery of the stimuli) was of great relevance.
The form of stimulation used in these studies (namely, the application of odorants in aqueous form), however, is not always comparable with food consumption and flavor perception in our everyday life. Small et al9 therefore conducted a study examining brain activation in response to identical orthonasal and retronasal stimuli applied in gaseous form with an olfactometer. As shown in Fig. 19.2 , stimuli were applied twice, orthonasally and then retronasally. Using a food odor (chocolate) and other, nonfood odors, Small et al were able to show that brain activation differed depending on the route of delivery of the food odor but not of the nonfood odors.9 These findings prove that food odorants perceived retronasally—which happens during food intake normally—are processed in different central networks from nonfood odors perceived retronasally. In combination with gustatory stimuli, retronasal olfactory stimulation, but not orthonasal olfactory stimulation, has been shown to accelerate swallowing activity.24 This is not surprising considering that retronasal olfactory stimulation activates a region of the Rolandic operculum at the base of the central sulcus,9 and that this area corresponds to a primary representation of the oral cavity.25 Looking at interactions the other way around, Bult et al26 were able to show that a simultaneous odor stimulus was able to influence perceived oral texture (in this case, thickness and creaminess) of food, but only when it was presented retronasally. The effect was most pronounced when odor presentation coincided with swallowing.
Simultaneous odor perception (if the odorants are perceptually similar) can enhance the perceived intensity of tastants; however, blocking the nose severely diminishes this effect.27 This phenomenon was shown by Sakai et al, who were able to demonstrate taste enhancement caused by simultaneously presented odors and, moreover, confirm that the effect observed was really due to simultaneous olfactory perception and not to taste stimulation.28 It has also been shown that the pure presence of an oral stimulus increases odor intensity, irrespective of whether the odorant is perceived orthonasally or retronasally.29 In a psychological experiment, Dalton et al were able to demonstrate that subthreshold concentrations of olfactory and gustatory stimuli integrate.30
However, there is more recent evidence that taste stimulation influences odor intensity perception31 and is important for localizing retronasally sensed odorants to the oral cavity.32 Interestingly, the localization effect occurs only for congruent taste-odor pairs. A sweet but not salty taste sensation causes subjects to report a vanilla odor appearing to come from the mouth, whereas a salty but not sweet taste sensation produces the appearance of a soy sauce odor.
In summary, all these data confirm that (1) odorants are processed differently when they are perceived orthonasally versus retronasally; and (2) they interact and influence taste perception differently.
Clinical Implications
So far, there has been a limited number of studies explicitly examining patients with isolated lesions. However, as previously mentioned, it has been shown that in patients with mainly orthonasal mechanical obstructions, such as polyps or chronic rhinosinusitis, retronasal olfactory perception is better than orthonasal olfactory perception.11 However, it has also been shown that in cases of olfactory loss for other reasons, retronasal olfactory perception can remain, at least partly.12 In cases of reduced orthonasal airflow (e.g., post-laryngectomy patients), retronasal olfaction is reduced as well.33 The exact underlying patho-physiology that might explain why orthonasal and retronasal olfactory perception differ is currently unknown.
There are also studies examining the effect of gustatory stimulation on olfactory perception. In a study by Welge-Luessen et al, it was shown that congruent paired stimuli, such as a sweet tastant in combination with a sweet odorant, modulate OERPs of the odorant and enlarge them, while a concomitant sour tastant enlarges the event-related potentials (ERPs) of simultaneously applied gaseous carbon dioxide.34 Olfactory stimulation, however, was only performed in an orthonasal way. Applying odorants not only orthonasally but also retronasally, it has been shown that food-like retronasally applied odors are processed significantly faster, resulting in shorter OERP latencies, in congruent odor–taste situations (food-like odor vanillin, plus sweet taste) than in incongruent smell–taste combinations (food-like odor vanillin, plus sour taste). Incongruent simultaneous gustatory stimulation applied during orthonasal olfaction seemed to induce conflict priming, also resulting in faster processing.35
To examine the influence of the vagal nerve on gustatory and olfactory processing, a small group of patients with vagal nerve stimulators (VNSs) was examined. It has long been suggested that interruption of a laryngeal–vagal feedback mechanism36 might contribute to olfactory dysfunction after laryngectomy,37 which might be considered to be consistent with findings from the early 1980s.38 On the other hand, it has also been shown that part of the hyposmia in post-laryngectomy patients is due to reduced airflow.39,40 Examining olfactory function in subjects with vagal stimulators, it was shown that vagal nerve stimulation prolonged P2 latencies of OERPs. In patients receiving a therapeutic benefit from VNSs in terms of seizure control, larger amplitudes during the “on” period than during the “off” period were observed.41 Even though the number of studies examining vagal–olfactory interaction is limited, there seems to be growing evidence that vagal–olfactory interaction does occur. It has also been shown that patients with olfactory disorders exhibit higher gustatory thresholds.42
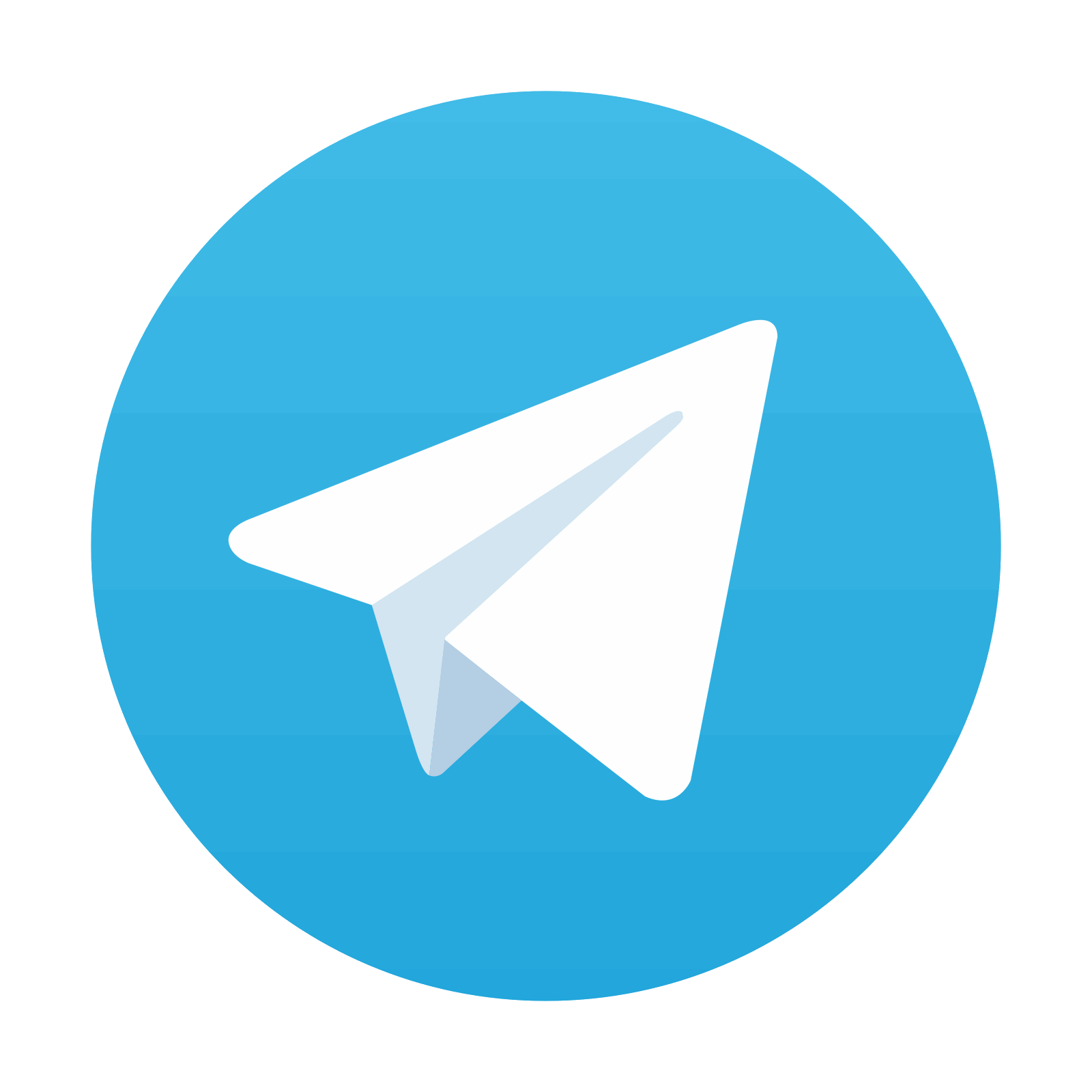
Stay updated, free articles. Join our Telegram channel

Full access? Get Clinical Tree
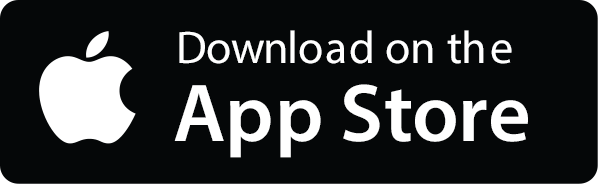
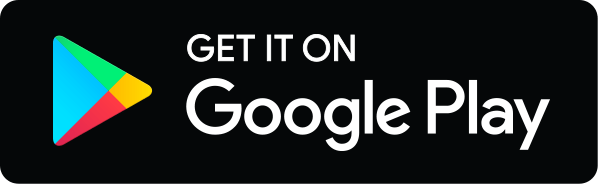
