Grade
Symptoms
0
No abnormality present
I
Short-term memory loss, reversal of sleep/wake cycle
II
Asterixis, apathy, inappropriate behavior, confusion, lethargy
III
Stupor, marked confusion, incoherent speech
IV
Comatose
Pathophysiology
Several pathophysiologic mechanisms appear to underlie the effects of FLF on the brain and may contribute to the development of seizures. The hallmark pathological feature of hepatic encephalopathy is astrocytic swelling. Astrocytes are rich in the enzyme glutamine synthetase, which catalyzes the conversion of ammonia to glutamine. When hepatic failure occurs, excessive ammonia is available for metabolism by glutamine synthetase. Intracellular glutamine accumulates, causing astrocytic swelling, breakdown of the blood-brain barrier (BBB), and cytotoxic edema [6].
Excessive glutamate, a major excitatory neurotransmitter, has been implicated in the development of seizures [7]. FLF models have demonstrated decreased GLT-1, a glutamate transport protein present on astrocytes [8] leading to reduced glutamate reuptake. Glycine, which activates excitatory n-methyl- d -aspartate (NMDA) receptors , has also been implicated. A similar animal model of FLF demonstrated decreased expression of Glyt-1, a glycine transport channel. Cerebral microdialysis in these animals revealed approximately three times the extracellular glycine concentration compared to controls [9].
Increased GABA-ergic tone may also play a role in both encephalopathy and, by inhibiting interneuron populations, the development of seizures. Flumazenil, a GABA antagonist, transiently improves neurological function in patients with hepatic encephalopathy, but randomized controlled trials have not demonstrated durable improvements in recovery or survival [10]. In astrocytic tissue samples, reuptake of GABA is inhibited by ammonia at concentrations associated with the development of hepatic encephalopathy [11]. Rabbit models of FLF have shown that the activity of the GABA-metabolizing enzyme GABA-transaminase is decreased, further supporting a role for excessive GABA activity [12].
Diagnosis
Seizures in the critically ill, particularly those in the medical or surgical intensive care unit, are typically nonconvulsive [13–15] and require continuous EEG (cEEG) monitoring in order to achieve sufficient sensitivity [15, 16]. In the early stages of hepatic encephalopathy, movement disorders such as asterixis/myoclonus may be difficult to distinguish from ictal movements such as clonus. cEEG, which includes video recording, is crucial to elucidate shaking or jerking spells observed in the ICU environment. Further complicating the clinical observation of seizures in patients with hepatic failure, patients with more severe hepatic encephalopathy (Grades III/IV) often require mechanical ventilation, analgosedation, and/or paralysis. These mask clinical seizure activity, and therefore the threshold for considering cEEG monitoring in patients with organ failure, including those with FLF, must remain low [17].
The background EEG of patients with liver failure is traditionally characterized by three stages correlating to the depth of coma [18]. Grade I/II encephalopathy is associated with θ frequency background slowing. As encephalopathy progresses, δ frequency slowing becomes more pronounced. Generalized, symmetric, and periodic waves described as “triphasic” in morphology appear—classically with a positive-polarity dominant second or third phase, a frontal predominance, and an anterior-posterior lag (Fig. 14.1). These were originally described in close association with liver failure [5], but subsequently these generalized periodic discharges with triphasic morphology have been reported across a variety of conditions including other organ failure syndromes and as the ictal pattern in some patients with seizures [19, 20]. The presence of generalized periodic discharges of any kind, including those with triphasic morphology, is highly associated with nonconvulsive seizures [21, 22], and others have observed that indeed seizures are more common in liver failure with brain dysfunction during this second stage. A third stage, consisting of a diffuse, asynchronous polymorphic δ pattern, is observed in the most severe stage of hepatic coma.


Fig. 14.1
Grade III encephalopathy including generalized periodic discharges with triphasic morphology. 41-year-old man with nonalcoholic steatohepatitis with cirrhosis and variceal bleeding. cEEG performed 4 days after transjugular intrahepatic portosystemic shunt procedure with increasing ammonia to 330 μg/dL and Grade III hepatic encephalopathy. The EEG background demonstrated predominantly delta frequency slowing with frequent, very brief runs of triphasic-appearing waveforms occurring at approximately regular intervals. These gradually resolved as mental status improved
The largest series to date retrospectively studied 118 of 526 patients admitted with a diagnosis of hepatic encephalopathy undergoing EEG. EEGs were typically “routine” and not continuous 24 h EEG. 18 patients (15%) were noted to have epileptiform abnormalities on EEG, and electrographic status epilepticus (SE) was observed in five patients (4%). Epileptiform activity was related to clinical deterioration or death, suggesting an association with poor prognosis [23]. More recently, medical and surgical ICU cohorts undergoing cEEG have not shown a relationship between acute hepatic failure and the presence of periodic discharges or seizures [13, 14].
After transplant, epileptiform abnormalities may increase in incidence during the first 7 days, with an incidence of seizures up to 42 % [24]. Mechanisms by which seizures occur posttransplant may be related to immunosuppressant medications or withdrawal from GABA-ergic metabolites, both pharmacologic and endogenous, that occur with a newly functioning liver [25].
Management
As with any seizures in the critically ill, initial steps in management include an assessment for readily reversible causes. Particularly in organ failure and endocrine crises, biochemical derangements such as hypoglycemia or hyponatremia are seen frequently and may act as a precipitant for seizures to develop. If these have been addressed, the choice of antiepileptic drug (AED) itself may be complicated: with the exception of levetiracetam, vigabatrin, pregabalin, topiramate, and gabapentin, almost all AEDs undergo some degree of hepatic conversion (Table 14.2). Further, patients with liver dysfunction exhibit several relevant physiologic changes that may also impact the decision about which AED to choose (Table 14.3).
Table 14.2
Pharmacology of Antiepileptic Drugs
Name | Routes of administration | Hepatic metabolisma | Renal eliminationb | Protein binding (%) | Dialyzable | Adverse effects | Serum concentration available | Special considerations |
---|---|---|---|---|---|---|---|---|
Phenytoin | IV, oral | ++++ | + | 80–95 | N | Arrhythmia, agranulocytosis, ataxia, hepatitis | Y | Nonlinear kinetics, weight-based dosing |
Carbamazepine | Oral | ++++ | + | 75–90 | Y | Dizziness, drowsiness, nausea, hyponatremia, anemia, agranulocytosis, skin reactions | Y | Autoinduction metabolism, major CYP3A4 substrate |
Oxcarbazepine | Oral | ++++ | ++ | 40 | No data | Hyponatremia, dizziness, drowsiness, nausea | Y | At steady-state concentrations the extended release product is not bioequivalent to the same daily dose of immediate release product |
Eslicarbazepine | Oral | ++ | +++ | <40 | Y (metabolites) | Hyponatremia, diplopia, blurred vision, hypertension, skin reactions | Y | |
Zonisamide | Oral | +++ | ++ | 40 | Y | Metabolic acidosis, increased BUN, nephrolithiasis, agranulocytosis, aplastic anemia | Y | Use not recommended with GFR < 50 mL/minute; monitor patients for suicidality |
Lamotrigine | Oral | ++++ | + | 55 | Y | Skin reactions, visual disturbance | Y | |
Lacosamide | IV, oral | +++ | ++ | <15 | Y | EKG changes, diplopia, blurred vision | Y | |
Ethosuximide | Oral | ++++ | + | 0–5 | Y | Blood dyscrasias, skin reactions, systemic lupus erythematosus | Y | |
Benzodiazepines | ||||||||
Diazepam | IV, oral | ++++ | + | 98 | N | Hypotension, amnesia, drowsiness, urinary incontinence, apnea | N | Active metabolite |
Midazolam | IV, oral | ++++ | + | 97 | N | Hypotension, drowsiness | N | |
Lorazepam | IV, oral | ++++ | + | 85–93 | N | Hypotension, sedation, dizziness | N | IV: Risk of propylene glycol toxicity |
Clonazepam | Oral | ++++ | + | 85 | N | Ataxia, drowsiness, edema | N | |
Clobazam | Oral | ++++ | + | 80–90 | N | Drowsiness, lethargy, sialorrhea, URTI, fever | N | |
Pentobarbital | IV | ++++ | + | 45–70 | N | Bradycardia, hypotension, hepatotoxicity | Y | |
Phenobarbital | IV, oral | ++++ | ++ | 20–40 | Y | Drowsiness, sedation, ataxia, cognitive and behavioral changes | Y | |
Primidone | Oral | ++++ | +++ | 30 | Y | Drowsiness, sedation, ataxia | Y | |
Tiagabine | Oral | ++++ | + | 96 | N | Dizziness, asthenia, lack of energy, somnolence | Y | |
Vigabatrin | Oral | + | ++++ | 0 | Y | Vision loss, anemia, somnolence, neuropathy, edema | N | |
Gabapentin | Oral | + | ++++ | <3 | Y | Dizziness, drowsiness, ataxia | Y | |
Pregabalin | Oral | + | ++++ | 0 | Y | Peripheral edema, dizziness, somnolence, weight gain | Y | |
Valproate | IV, oral | ++++ | + | 80–90 | N | Headache, drowsiness, dizziness, N/V, thrombocytopenia, alopecia, tremor | Y | Nonlinear kinetics, weight-based dosing |
Felbamate | Oral | +++ | ++ | 22–25 | No data | Somnolence, dizziness, anorexia, N/V | Y | |
Topiramate | Oral | + | +++ | 15–41 | Y | Paresthesias, drowsiness, dizziness, weight loss | Y | |
Levetiracetam | IV, oral | + | +++ | <10 | Y | Headache, behavioral problems, drowsiness, irritability | Y | |
Ketamine | IV | +++ | + | 60 | N | Hypertension, tachycardia, emergence reaction (hallucinations, excitement, etc.) | N | Weight-based dosing |
Propofol | IV | ++ | + | 97–99 | N | Hypotension, hyperlipidemia, propofol-related infusion syndrome | N | |
Perampanel | Oral | ++++ | + | 95–96 | No data | Dizziness, drowsiness, headache, hostility, falls | Y | |
Rufinamide | Oral | ++++ | + | 34 | N | Shortened QT interval, headache, drowsiness, dizziness | Y | Weight-based dosing; absorption increases with food |
Ezogabine | Oral | ++++ | ++ | 80 | No data | Urinary retention, retinal abnormalities, QT prolongation | Y | May falsely elevate serum/urine bilirubin |
Table 14.3
Metabolic changes associated with fulminant liver failure
Physiologic Alteration | Effect |
---|---|
Increased blood flow to liver (early) | Increased drug metabolism |
Hepatocyte death and P450 dysfunction | Increased circulating levels of hepatically metabolized antiepileptic drugs |
Decreased synthetic function | Hypoalbuminemia and increased free levels of protein-bound antiepileptic drugs |
Enhanced sensitivity to hepatotoxic side effects | Decreased tolerance of certain hepatically metabolized antiepileptic drugs |
Acute seizures and particularly SE should be treated as medical emergencies [26]. Benzodiazepines form the first line of therapy based on two randomized controlled trials for SE in the prehospital setting [27, 28]. Although benzodiazepines are extensively metabolized by the liver (Table 14.2), they are not considered hepatotoxic as a class and therefore are considered safe to use as first line in those with acute liver failure. Benzodiazepines with shorter t 1/2 such as lorazepam might avoid prolonged sedation and therefore may be preferred as an initial benzodiazepine. Further, lorazepam is metabolized by glucuronidation (along with older benzodiazepines oxazepam and temazepam), and therefore although liver failure may affect its overall clearance, there are no active metabolites formed that may prolong effects of the medication, as is the case with diazepam.
Several AEDs are known to have to direct hepatotoxic effects on the liver, particularly phenytoin and phenobarbital, which may rarely cause toxic or hypersensitivity hepatitides. Valproic acid is a short-chain branched carboxylic acid with multiple active metabolites, including 4-ene-valproic acid, which may exhibit hepatotoxicity. Through metabolites formed via the carnitine shuttle, hypocarnitinemia and hyperammonemia may develop even in those with normal liver function [29]. Valproic acid should be avoided in patients with hepatic failure where possible, and supplementation with l-carnitine should be considered if hyperammonemia or other signs of hepatic failure occur in the setting of valproic acid use [30].
Despite the association between liver failure with brain dysfunction and the development of seizures or epileptiform abnormalities on EEG, routine prophylaxis with AEDs is not currently recommended. Two studies have investigated the role of phenytoin for prophylactic use in hepatic failure [4, 31]. One study of 42 patients with Grade III/IV encephalopathy used the cerebral function monitor, a limited montage EEG-based signal processing method used in the OR or ICU setting. Electrographic seizures were documented in 15% of those randomized to phenytoin prophylaxis compared to 32% without phenytoin. Of the 25 that died, 19 were autopsied; significantly fewer in the prophylaxis group had cerebral edema [4]. However, in another study of 42 patients, no difference in clinical seizures, clinical manifestations of cerebral edema, or survival was observed when phenytoin was used prophylactically [31]. When cerebral edema and intracranial hypertension are present, prophylactic AEDs in general may serve to optimize patients who might otherwise not be operative candidates for liver transplantation. Given the potential for toxicity, larger studies are warranted before phenytoin is used as a primary agent; the authors prefer levetiracetam in those with hepatic failure. The authors recommend AEDs only for those patients with confirmed clinical or electrographic seizure activity, for those with EEG patterns highly associated with seizures (e.g., periodic discharges), or as prophylaxis in those in coma with global cerebral edema or other signs of elevated intracranial pressure.
Hepatic Conditions Associated with Seizures
Wilson’s Disease
Wilson’s disease (WD) is an autosomal recessive disorder of copper metabolism that results from abnormalities in the ATP7B gene. WD results in the deposition of copper in the brain, liver, and cornea (referred to as Kayser-Fleischer rings). Patients may manifest myriad symptoms ranging from primarily neuropsychiatric to purely hepatic [32]. Patients with WD develop chronic seizures at a rate of 6–8%, substantially more than the incidence of epilepsy in the general population. Nearly three-quarters will develop seizures upon initiation of chelation treatment or shortly thereafter [33, 34].
The pathophysiology of seizures in WD may be related to (a) a relative pyridoxine deficiency specifically during chelation with penicillamine, (b) direct toxicity from liberated copper, or (c) deafferentation of subcortical white matter tracts from the overlaying cortex caused by large copper deposits. Early literature suggested that rats treated with penicillamine chelation became pyridoxine deficient and were more likely to develop seizures [35]. Others have countered that neurotoxicity associated with the mobilization of copper during chelation contributes to the seizures seen during and just after treatment [33]. Cavitary lesions in subcortical white matter, caudate, or putamen may be seen on MRI or autopsy and may predict the development of refractory seizures [36–38]. Once therapy is complete, the majority, 84.5% of patients in one series, experience substantial improvement in seizure frequency [33]. The authors suggest pyridoxine supplementation in conjunction with AEDs in the treatment of seizures that occur in patients undergoing chelation therapy.
Reye’s Syndrome
Reye’s syndrome is a rare, rapidly progressive encephalopathy marked by liver dysfunction. Viruses, including influenza, coxsackie, measles, and varicella, are epidemiologically linked with Reye’s syndrome [39], which develops with co-exposure to salicylates. Though mostly a pediatric illness, adult cases have been reported [39, 40]. Clinically, Reye’s syndrome begins with confusion and somnolence and progresses to seizures and coma. Fatty infiltration of the abdominal viscera, hepatomegaly, and cerebral edema are observed, but icteric changes are mild or absent. Cases are extraordinarily rare, and while the pathogenesis is not well understood, electron microscopy studies of rat liver hepatocytes have demonstrated uncoupling of oxidative phosphorylation, swelling, and cell permeability when exposed to salicylates [41]. Outcomes of patients with Reye’s syndrome have been associated with the degree of background slowing on electroencephalography (EEG), with those demonstrating diffuse slowing ultimately succumbing to their illness [42, 43]. Seizures have been considered a poor prognostic marker and are observed in approximately half of patients [43], although a good outcome has been reported in a patient with Reye’s syndrome and SE [44].
Hepatic Porphyrias
The hepatic porphyrias (including acute intermittent porphyria, ALA dehydratase deficiency porphyria, variegate porphyria, and hereditary coproporphyria) are caused by various enzymatic deficiencies in the heme synthesis pathway, with resulting accumulation of porphyrin precursors. Reported incidence of seizures may be as high as 5 % in those who present with symptoms of an acute porphyric attack [45]. Proposed mechanisms leading to encephalopathy and seizures involve δ-aminolevulinic acid crossing the BBB and (1) affecting GABA, and possibly glutamate, receptors, (2) inducing oxidative stress/oxygen radical damage, and (3) inhibiting the neuronal Na+/K+ ATPase [46]. Treatment of seizures related to porphyria starts with hematin infusions which terminate further porphyrogenesis by inhibiting ALA synthetase, thus correcting the underlying pathology.
Several commonly used AEDs may induce, or worsen, porphyric attacks in patients with hepatic porphyrias, primarily by inducing cytochrome p450 isoenzyme metabolism and accelerating the generation of heme pathway byproducts.
AEDs that exacerbate porphyrias:
Phenobarbital
Phenytoin
Valproic acid
Carbamazepine
Lamotrigine
Topiramate
Tiagabine
Ethosuximide
Diazepam
These AEDs should be avoided or stopped immediately. Non-hepatically cleared AEDs such as, gabapentin [47] and levetiracetam [45] are preferred. Clonazepam has been used successfully [48] after failure of phenytoin and valproic acid, but refractory SE has been reported [49], in which case propofol may be used as a preferred continuous intravenous anesthetic agent instead of pentobarbital and perhaps even midazolam, which has been shown to induce prophyrogenesis even at relatively low concentrations [50, 51]. Interestingly, the GABA-ergic and antioxidant effects of melatonin may benefit patients with porphyrias: a small series found that patients with seizures had decreased urinary excretion of melatonin compared to those without seizures [52].
Renal Failure
Background
Renal failure, specifically acute kidney injury , is a common clinical problem encountered in the ICU. Renal failure is associated with increased length of hospital stay, resource utilization, and mortality [53]. Acute kidney injury occurs in up to 25% of those hospitalized in an ICU [54], though this estimation may be higher depending on the definition used; approximately 6% will require renal replacement therapy [55].
Current definitions of acute kidney injury exist from several groups (Table 14.4). All use serum creatinine and urine output as principle markers of renal compromise. As renal clearance decreases, azotemia develops, and while the absolute level of azotemia does not appear to correspond with changes in neurological functioning, the rapidity and degree of renal dysfunction correspond both to encephalopathy and increasing seizure risk. Encephalopathy may initially manifest as fatigue, forgetfulness, or decreased attention, but progresses to include bifrontal dysfunction and finally frank delirium with tremulousness or asterixis/myoclonus, hallucinations, and obtundation. Between 2 and 10% of those with renal failure requiring dialysis and up to one-third of patients with acute uremic encephalopathy are reported to have seizures or SE, most in later stages of encephalopathy [59, 60].
Table 14.4
Definitions of acute kidney injury (AKI)
Group | Definition | Reference |
---|---|---|
Kidney Disease: Improving Global Outcome (KDIGO) | 1. Increase in serum Cr of 0.3 mg/dL developing over <48 h or >50% over 7 days OR 2. Urine output of <0.5 mL/kg/h for >6 h | [56] |
Acute Kidney Injury Network (AKIN) | 1. Increase in serum Cr of 0.3 mg/dL or >50% developing over <48 h OR 2. Urine output of <0.5 mL/kg/h for >6 h | [57] |
Risk, Injury, and Failure (RIFLE) | 1. Increase in serum Cr of >50% developing over <7 days OR 2. Urine output of <0.5 mL/kg/h for >6 h | [58] |
Pathophysiology
Blood urea nitrogen (BUN) is commonly used to evaluate renal function, and its removal from serum is directly associated with improved patient mortality [61]. By itself, urea does not appear to be toxic despite its nominal association with uremic encephalopathy. However, its presence acts as a surrogate marker of failed renal clearance, with unmeasured metabolites more likely contributing to the neurologic findings seen in uremic encephalopathy.
Both excitatory and inhibitory neurotransmitters become disrupted when organic substances are not adequately filtered. In particular, guanidino compounds (GC) such as guanidinosuccinic acid have been reported to be increased in the brain and cerebrospinal fluid (CSF) [61–63]. By complex interactions with GABA, glycine, and NMDA receptors, GCs may contribute both to encephalopathy and the development of seizures. Methylguanidine has been shown to inhibit neuronal Na+/K+ ATPase as well and therefore may have a direct effect on neurotransmission or seizure propagation. Azotemia has been linked with dysfunction of the neuronal Na+/K+ ATPase, as well as the Na+/Ca++ exchange mechanism and the ATP-dependent calcium transport system [64, 65]. Experimental introduction of GCs directly into CSF has been shown to induce tonic-clonic activity in animal models in a dose-related fashion [66].
Evidence suggests total brain calcium is increased in renal failure [63]. This is likely the result of parathyroid hormone (PTH)-sensitive neuronal calcium transporters. Increased serum phosphate levels act to lower serum calcium in renal failure, stimulating PTH secretion (secondary hyperparathyroidism). Calcium excess is linked to neuronal hyperexcitability, and the relationship between calcium/hyperparathyroidism and neuronal dysfunction has been supported using canine models [67]. After induction of uremia, increases in EEG power and bursting (paroxysms of high-amplitude delta activity/total duration of the record) have been reported. In those with EEG changes, total brain calcium was noted to be significantly elevated. Interestingly, animals in whom parathyroidectomy was performed prior to induction of uremia lacked electroencephalographic abnormalities, suggesting a distinct role of parathyroid hormone in the pathogenesis of encephalopathy associated with renal failure.
In addition to the clearance of organic compounds and the regulation of calcium, the kidney is central to the homeostasis of vascular tension and volume management through maintenance of fluid and solute balance, in part through its endocrine function via the renin-angiotensin axis. Alternations in kidney function, and particularly in filtration rates, may manifest as malignant hypertension. Often severe, renal failure-related hypertension may be associated with cerebral vascular dysfunction, loss of cerebral autoregulation, and the reversible posterior leukoencephalopathy syndrome (PRES). In a series of 15 patients with PRES, nine had had a documented creatinine values >1.2 (range: 1.2–16.1), of whom seven developed clinical seizures [68].
Several medications may contribute to seizure development in patients with renal failure, many with common use in ICU care.
Selected common ICU drugs associated with seizures in renal failure:
Acyclovir and valacyclovir
Antihistamines (diphenhydramine, cimetidine)
Baclofen
Bupropion
Carbapenems (imepenem, ertapenem, and meropenem)
Cephalosporins (cefazolin, cefepime, ceftazidime, and ceftriaxone)
Clozapine
Cyclosporine
Isoniazid
Lithium
Meperidine
Metronidazole
Penicillins
Polymyxin B
Quinolones
Tramadol
Tranexamic acid
Tricyclics
Figure 14.2 demonstrates a case of a patient in renal failure, clozapine toxicity, and NCSE.


Fig. 14.2
Status epilepticus due to clozapine toxicity in renal failure. 58-year-old woman with schizoaffective disorder on home clozapine presented unresponsive with acute on chronic renal failure (Cr 1.9, BUN 32) in the setting of sepsis with hypoglycemia. Despite correction of hypoglycemia and appropriate antibiotics, she remained comatose. Clozapine levels were supratherapeutic at 1987 ng/mL. (a) Initial cEEG demonstrated nonconvulsive status epilepticus comprised of continuous high-amplitude 2–3 Hz generalized sharp-wave complexes. (b) Lorazepam was administered with improvement in both the background EEG and clinical exam. Levetiracetam was started, and clozapine was stopped with gradual improvement of mental status over several days
The cephalosporins (particularly cefepime), carbapenems, and penicillins have all been implicated in seizure development, possibly by competitively antagonizing GABA receptor sites [69–71]. Adequately adjusting doses of renally cleared antibiotics based on glomerular filtration rate should mitigate the risk for such complications; however, the expertise of a clinical pharmacist is required to ensure adequate antibiotic concentrations . Acyclovir and its prodrug valacyclovir may also induce seizures [72] during treatment for encephalitis. Neurotoxicity may be distinguished from symptoms of encephalitis by the absence of fever, headache, focal neurological signs, and a lack of EEG patterns suggestive of temporal encephalitis, namely, lateralized periodic discharges [73]. Patients taking baclofen who develop renal failure may develop encephalopathy and coma—even in low doses [74]. While seizures are uncommon, the encephalopathy is severe, and EEG may demonstrate generalized periodic discharges that are challenging to distinguish from ictal activity. If neurotoxicity is suspected, discontinuation of the medication and potentially hemodialysis may help reduce ongoing neurotoxicity, although responses may not be immediate due to central nervous system accumulation.
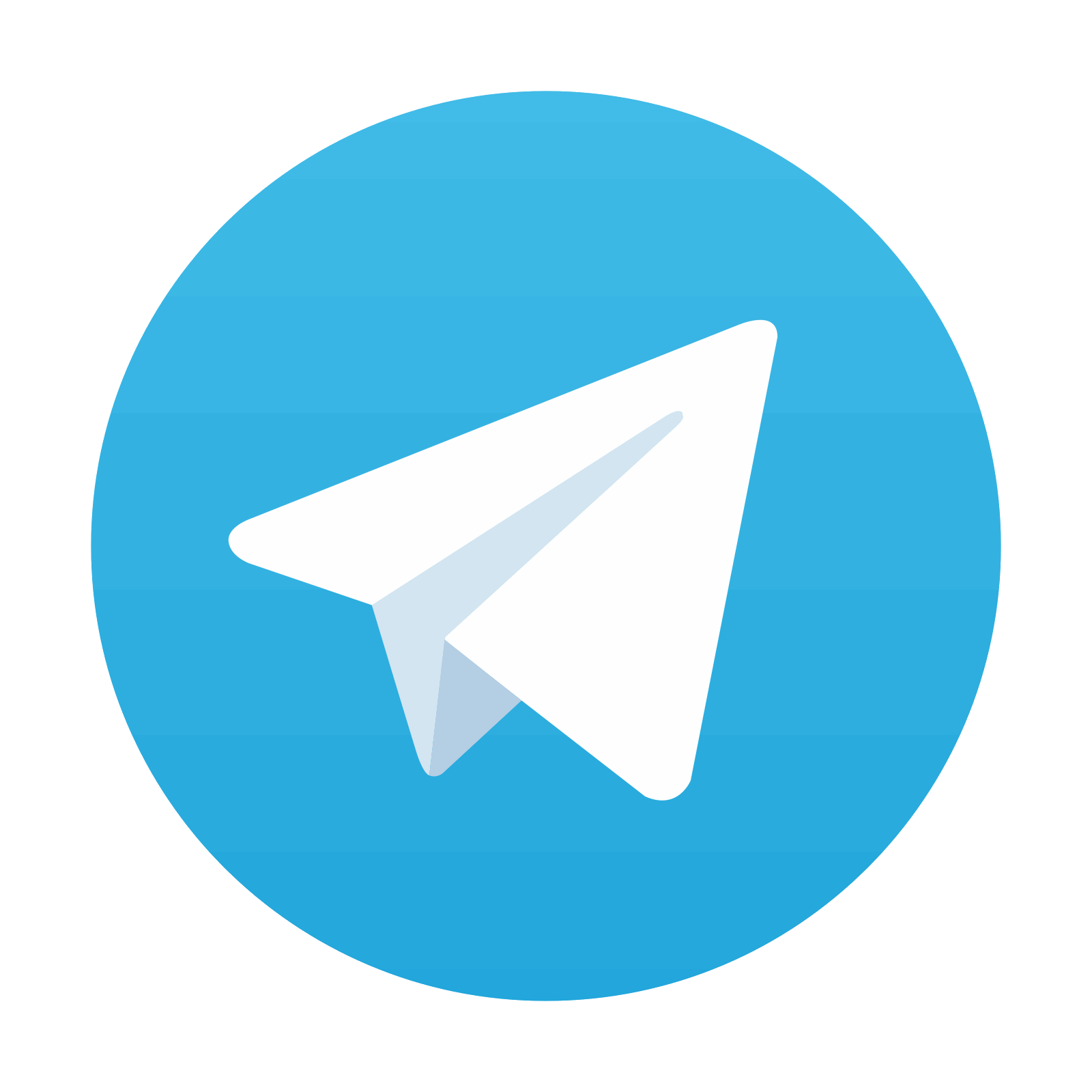
Stay updated, free articles. Join our Telegram channel

Full access? Get Clinical Tree
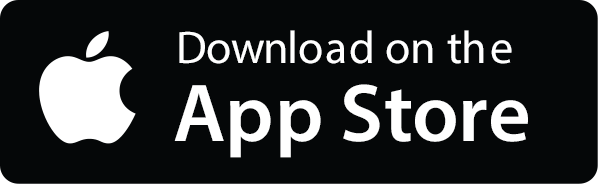
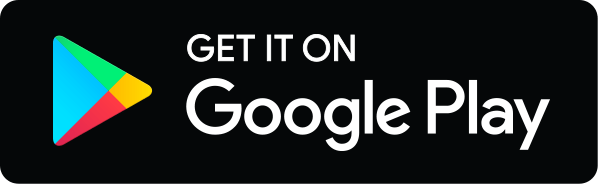