© Springer International Publishing AG 2018
Nivedita Agarwal and John D. Port (eds.)Neuroimaging: Anatomy Meets Functionhttps://doi.org/10.1007/978-3-319-57427-1_1111. Functional Anatomy of the Cerebellum and Brainstem
(1)
S. Maria del Carmine Hospital, Azienda Provinciale per i Servizi Sanitari, Rovereto (TN), Italy
(2)
Center for Mind/Brain Sciences (CIMeC), University of Trento, Rovereto (TN), Italy
(3)
Department of Radiology, Section of Neuroradiology, University of Utah, Salt Lake City (UT), USA
In this chapter, we will briefly review the functional anatomy of the cerebellum and brainstem, cross-referencing Chaps. 7 and 8 with references to relevant figures. We will describe the major functions of each structure and identify specific deficits that can localize disease to a specific brain region. As the longitudinally oriented white matter tracts span more than one part of the brainstem, they are discussed separately at the end of the chapter.
Where possible, important syndromes have been outlined. These descriptions are not intended to be comprehensive, but rather to present a sample of function and pathology. For more comprehensive descriptions, the reader is referred to standard texts of neurology.
11.1 Cerebellum
On axial and coronal images (Figs. 7.1, 7.14), a midline portion or the vermis can be recognized from the more lateral cerebellar hemispheres. In addition, the primary fissure separates a more cranial anterior lobe from the caudal posterior lobe. The posterolateral fissure separates the posterior lobe from the flocculonodular lobe.
11.1.1 Motor Functions
As detailed in Chap. 7 (Table 7.1), the vermis (archicerebellum or spinocerebellum) receives input primarily from the spinal cord. It is important in the control of muscle tone and axial limb movements, maintaining posture of the antigravitational muscles. Asymmetric homotopic representation of the sensorimotor cortex is represented in the vermis and the paravermis. The flocculonodular lobe (paleocerebellum, vestibulocerebellum) is heavily interconnected with the vestibular nuclei and brainstem nuclei for the important head and eye movement coordination.
11.1.2 Non-motor Functions
The cerebellar hemispheres (neocerebellum) receive input from the brain through the pontine nuclei. These areas are responsible for the non-motor functions of the cerebellum such as cognition, language and emotion processing, and modulation. fMRI studies have identified specific cerebellar regions that process cognitive and emotional information. In addition to a precise homotopic mapping of the cognitive functions, a functional asymmetric representation of executive function is also maintained in the cerebellum. For instance, language is lateralized to the right posterior hemisphere. Visuospatial functions appear to be lateralized more to the left cerebellar hemisphere.
11.1.3 Functional subdivisions
Anatomical subdivision predominantly considers cerebellar nomenclature of Ito [1] and Larsell [2], as detailed in Chap. 7 and seen in Figs. 7.1–7.3.
Lobule I: specific function is unknown. Lesions in the lingula of primates disrupt vestibulocerebellar and spinocerebellar integration, resulting in axial muscle disequilibrium [3]. A malformed thick lingula may represent an endophenotype to increased risk of alcohol and substance use by creating enhanced tolerance to these drugs [4].
Lobules II–V (anterior lobe): the primary sensorimotor region of the cerebellum is located in this lobe and in the adjacent part of lobule VI [5]. The anterior lobe also receives input from the prefrontal cortex (area 46) for skilled motor functions. Sensorimotor homunculi have been precisely mapped to this lobe together with the paravermian area [6]. Right-handed finger tapping, flexion-extension, and pronosupination tasks will activate right lobules IV, V, and VIII.
Lobules VI and VII: receive contralateral input mostly from association areas such as prefrontal cortex, posterior parietal, superior and middle temporal areas, cingulate gyrus, and retrosplenial cortex [7, 8]. Thus, most cognitive tasks such as verb generation, mental rotation, and 2-back tasks for memory can activate both VI and VII lobules. Lobule VI also represents the language motor articulatory apparatus (Urban 2003). Lobules VI and VII are also considered the “oculomotor vermis” involved in controlling eye movements.
Lobule VIII: secondary sensorimotor region of the cerebellum [9].
Lobule IX: essential for visual guidance of movement. Recent functional connectivity magnetic resonance imaging (fcMRI) data indicate that it contributes to the default mode network [10].
Lobule X: gaze stability, smooth pursuit, and vestibulo-ocular reflex. These require active inhibition of unwanted saccades. Eyeblink conditioned response requires memory of eye movement partly stored in the vestibulocerebellar regions.
Higher level cognitive functions mainly are subserved mainly by the cerebellar hemispheres (Crus I and Crus II) and partly by some lobules in the vermis such as lobule VI, Lobule VIIA and VIIB). Language areas are predominantly represented in the right hemisphere regions of lobule VI, Crus I, and Crus II. Working memory tasks activate bilateral regions of lobule VI and Crus I and parts of VIII with cognitive areas situated laterally. Affective and emotional functions limbic cerebellum such as response to pain, panic, and air hunger activate most midline vermis, lobule VI, and Crus I [9].
Oculomotor regions of the cerebellum include lobules VI, VII, and IX together with the fastigial nucleus, Crus I and Crus II, flocculonodular lobe, and the uvula. These areas help maintain steady gaze. They are involved in the control of saccades, horizontal smooth pursuit, and the vestibulo-ocular reflex (eyes move conjugately in the opposite direction to horizontal head motion). While specific cerebellar regions have not yet been identified, brainstem-cerebellar circuits underlie conditioned and unconditioned eye blinking. Eye blinking has also been linked to cerebellar mechanisms of learning through reciprocate connections with the amygdala and other limbic areas [11].
Three pairs of pontine nuclei are present in the deep white matter of the cerebellum (Fig. 7.9). The dentate nuclei are involved in speech control, activate strongly with increasing complexity of sensorimotor tasks such as discrimination tasks, motor grip control, and are only weakly involved during simple motor tasks [12]. Multiple non-motor tasks also involve the dentate such as visuospatial tasks and verbal working memory. The fastigial nuclei are important in programming eye saccades. The interpositus (emboliform and globose) nuclei are involved in eye blinking responses and critical to learning and memory of conditioned responses [13]. Limb movement coordination is also modulated by these nuclei, and lesions may lead to limb ataxia.
Three pairs of peduncles supply input and output to and from the cerebellum (Figs. 7.12, 8.2–8.4). The inferior cerebellar peduncles (restiform bodies) are composed of fibers that receive and transmit signals to and from the spinal cord and brainstem nuclei, specifically, sensory proprioceptive signals from the spinocerebellar tracts and motor vestibular-ocular signals from the brainstem nuclei. The middle cerebellar peduncles (brachium pontis) are composed of fibers that carry input from the contralateral cerebral hemisphere, mainly relayed through the pontine nuclei. The superior cerebellar peduncles (brachium conjunctivum) are composed of fibers that carry primarily output signals to the contralateral cerebral hemisphere relayed through the red nucleus.
11.1.4 Cerebellar Pathology
Motor deficits of the cerebellum include primarily sensorimotor and oculomotor deficits. Sensorimotor deficits involve disorders of timing, lack of control of corticomotor excitability, deficits in sensorimotor synchronization, and making motor predictions that impair coordination of movement (dysmetria, dysdiadochokinesia). These result in typical “cerebellar-like” disequilibrium with lack of control of axial muscles, gait ataxia, and impaired coordination (dysmetria) of the extremities [11]. Ataxic dysarthria, often seen in patients with cerebellar stroke that damage the muscles of the phonatory apparatus, is pathologic speech characterized by slow, monotonous, scanned, indistinct, jerky, explosive, and slurred production due to alterations in phonation and articulation of consonants and vowels. Oculomotor deficits involve loss of the vestibulo-ocular reflex in comatose patients with brainstem damage resulting in “doll’s eyes” (eyes do not move to the opposite direction when the head is turned sideways). Lesions in the flocculus can cause gaze-evoked nystagmus. Lesions in the nodulus can result in periodic alternating nystagmus. Stroke and tumors in the cerebellum can cause ipsilateral reduction of the eyeblink-conditioning reflex. Similarly, cerebellar cortical degeneration and other diseases involving reduction of eyeblink conditioned response should point to a cerebellar involvement.
Non-motor deficits of the cerebellum, forming constellations of symptoms, are grouped into syndromes. Lesions in the dentato-thalamocortical pathway and emotional/affective loops lead to “dysmetria” of thought, with impairments of the cerebellar modulation of intellect and emotion [14]. Several levels of language deficits may occur in different neurodevelopmental, neurodegenerative and vascular diseases leading to ataxic dysarthria, disprosody and speech apraxia [15]. Neurodevelopmental abnormalities such as autism and schizophrenia are associated with cerebellum maldevelopment [16].
Cerebellar mutism syndrome (CMS) is characterized by the inability to produce and process speech without apraxia or aphasia typical of cerebral cortical lesions. Severe dysarthria and/or anarthria, unrelated to damage to muscles of the phonatory apparatus, leads to loss of verbal fluency and modulation of speech [17]. Lesions in the posterior midline and paramedian vermis, dentate nuclei, and superior cerebellar peduncle can result in CMS [18]. CMS is mostly reversible but may result in a more chronic complex cognitive and affective dysfunction.
Cognitive cerebellar affective syndrome (CCAS) is chronic changes in cognition and affect due to damage to midline structures of the posterior vermis and parts of the posterior lobe. CCAS is characterized by symptoms including: (a) impairment of executive functions such as deficient planning, verbal fluency, abstract reasoning, and working memory, (b) impaired visuospatial memory, (c) personality changes with blunting of affect and disinhibited and inappropriate behavior, and (d) la nguage deficits including agrammatism, mild anomia, and dysprosodia [19]. This is not accompanied by “cortical” symptoms such as aphasia, agnosia, and apraxia.
Rostral vermis syndrome (anterior lobe syndrome) is characterized by selective atrophy of the anterior vermis. The posterior or caudal vermis and most of the cerebellar hemispheres appear normal. The most common symptoms are wide-based stance and hesitating gait, gait ataxia and little or no involvement of the arms, speech, and ocular motility (arm incoordination, nystagmus, and dysarthria). This syndrome is best exemplified in patients with chronic alcoholic degeneration.
Caudal vermis syndrome occurs with damage to the flocculonodular lobe. It is characterized by axial disequilibrium and staggering gait, markedly impaired tandem gait, little or no limb ataxia, and spontaneous nystagmus and head rotation to the side. This syndrome is more commonly seen in pediatric populations with midline tumors such as medulloblastoma.
Cerebellar hemispheric syndrome is a severe constellation of symptoms including severely affected coordination of ipsilateral limbs as well as fine tremor arising only during voluntary movement. This syndrome is more commonly seen with cerebellar neoplasms, infarcts, and hemorrhage.
Pancerebellar syndrome presents with bilateral signs of cerebellar dysfunction characterized by various degree of signs and symptoms including incoordination of limbs, nystagmus, and dysarthria.
11.2 Brainstem
The brainstem develops from caudal two of the three embryological vesicles: the more rostral mesencephalon (that develops into the midbrain) and the rhombencephalon. The rhombencephalon further subdivides into the metencephalon (that develops into the pons and cerebellum) and the myelencephalon (that develops into the medulla oblongata). All nuclei and tracts respect a columnar organization along the rostral-caudal axis of the brainstem. Thus, it is important to remember that some cranial nerve nuclei and most white matter tracts run through the entire length of the brainstem. It is a combination of symptoms that helps to localize pathology in one of the three parts of the brainstem.
In the developing central nervous system, a basal plate and an alar plate are formed delimited by the sulcus limitans. The alar plate is the dorsal part of the neural tube, whereas the basal plate is the ventral portion of it. The alar plate continues caudally into the sensory dorsal part of the spinal cord, and the basal plate continues to form the motor part of the spinal cord. In the brainstem the alar plates move out laterally and contain the general somatic and general and special visceral afferents of the cranial nerves. Ascending sensory tracts are also seen more laterally. The basal plate contains the motor axons and contains the general somatic and general and special visceral efferents. The motor descending fibers are also mostly found in the medial part of the brainstem. The brainstem contains most of the encephalic reticular centers. The brainstem reticular nuclei are divided into three longitudinal columns: median, central (or medial), and lateral. Median nuclei are the raphe nuclei and are cholinergic, locus coeruleus contains noradrenergic fibers and the periaqueductal gray matter (PAG), and the reticular nuclei are mostly serotoninergic.
In this chapter, the contents of each brainstem section have been further subdivided into gray matter and white matter structures for easy reference. The functions of the cranial nerves have been detailed separately in Chaps. 12–23 and will not be further discussed in this chapter. The function of the white matter tracts will be discussed at the end of the chapter.
11.2.1 Midbrain Function
Superior colliculi (SC): The SC receive their primary input from retinal visual pathways and the frontal eye fields. Their most important function is controlling eye movement in “egocentric” (body specific) space allowing for voluntary saccadic movements. The SC also receive sensory input from other modalities such as auditory and somatosensory, helping to orienting head and eyes toward objects that are voluntarily seen or heard [20].
Inferior colliculus (IC): Tonotopic synapses in the auditory system. The IC receives input bilaterally from the dorsal cochlear nuclei. There is a certain degree of asymmetry in the input, with ipsilateral fibers being more numerous. Auditory information is then transmitted to the ipsilateral medial geniculate body. The IC also receives and sends information through multiple parallel loop connections to the ipsilateral auditory cortex. The IC represents the main multisensorial hub and allows for the startle response. It is important in the spatial localization of sound [21]. Contralateral nonpulsatile tinnitus, diminished capability to localize sounds, and presbycusis all have been associated with IC pathology.
Overview of Main Structures in the Midbrain
Major landmarks
Upper boundary of the midbrain is the plane of the mammillary bodies and superior colliculi; lower boundary is the plane of the inferior colliculi and pons.
Major Nuclei
Cranial Nerve
Motor
Sensory
Mesencephalic trigeminal nuclei (V) (Chap. 16)
Superior colliculi
Inferior colliculi
Red nuclei
Substantia nigra
Periaqueductal gray matter
Ventral tegmental area
Reticular activating system
Median centralis superior (median raphe nucleus)
Nucleus raphe dorsalis (dorsal raphe nucleus)
Major Tracts
Motor tracts
Cerebral peduncles
Tectospinal tract
Rubrospinal tract
Medial longitudinal fasciculus
Sensory tracts
Medial lemniscus
Spinothalamic tract
Lateral lemniscus
Central tegmental tract
Pretectal nuclei: A group of nuclei just anterior to the superior colliculi in the midbrain and has extensive connections with the thalamus, Edinger-Westphal nucleus, pons, and inferior olive to name a few. They receive direct input from the retinal ganglion cells and are important in the classic pupillary light reflex. They allow eyes to follow a moving object (smooth pursuit) and allow for the accommodation reflex (through the CN III). They also participate in REM sleep [22].
Edinger-Westphal nuclei: Parasympathetic center for motor innervation of intraocular muscles, constriction of pupil, and accommodation reflex [23].
Red nucleus (RN): The RN is involved in motor coordination. Major inputs are from the contralateral cerebellar hemisphere and ipsilateral motor cortex. Major outputs are to the inferior olive (in the medulla), the cerebellum (via the central tegmental tract, forming the “triangle of Guillian and Mollaret”), and the spinal cord (rubrospinal tract) [24]. The rubrospinal tract is more important in primates and may be vestigial in humans.
Substantia nigra (SN): The substantia is anatomically divided into a more medial pars compacta (SNpc) and a more lateral pars reticulata (SNpr). The SNpc sends dopaminergic output to striatum, initiating movement by disinhibiting thalamocortical pathways. The SNpc is also important in habit learning, goal-directed behavior, reward, pleasure, addictive behavior, and sleep-wake circadian cycle [25]. The SNpr sends GABAergic signals to the basal ganglia and other brain structures including the superior colliculus, modulating saccadic eye movement. Subthalamic nuclei may enhance spontaneous firing of SNpr neurons and are an important site for deep brain stimulation in patients suffering from tremor, rigidity, and bradykinesia [26].
Ventral tegmental area (VTA): The VTA is a group of gray matter nuclei in the ventral part of the mesencephalic tegmentum that give rise to dopaminergic mesolimbic (nucleus accumbens, SNpc, hippocampus, amygdala, olfactory cortex) and mesocortical (prefrontal cortex) reward mechanism circuitry.
Periaqueductal gray matter (PAG): The PAG contains opioid receptors. Spinothalamic tracts carrying pain and temperature information reach PAG. Together with the activation of the raphe nuclei, neurotransmitters and neuromodulators such as encephalin, substance P, and serotonin are released, activating neurons in the VPL of the thalamus reducing pain input. It is one of the sites of deep brain stimulation in chronic neuropathic pain. The PAG is also part of neuronal circuits underlying the expression of positive symptoms [27] in schizophrenia and manic depression. PAG activity may also be involved in maternal behavior through activation of oxytocin and vasopressin activity of the hypothalamus and the orbitofrontal cortex [28].
Reticular activating system (RAS): The RAS is an ill-defined group of different interconnected nuclei in the brainstem. The RAS includes raphe nuclei in the median part of the brainstem as well as the gigantocellular and parvocellular nuclei in the more lateral part of the brainstem. The RAS regulates cardiovascular and respiratory function, organizes eye movement, regulates sleep-wake cycle, and determines level of consciousness/arousal [29, 30]. It also helps maintain balance and posture.
The raphe nuclei are serotoninergic nuclei within the RAS that project to the medial forebrain and hypothalamic nuclei. They are involved in different types of reward systems and sleep-wake cycle. Dysfunction of the raphe nuclei (in particular dorsal raphe nucleus) is implicated in depression and obsessive-compulsive disorder and can cause narcolepsy and cataplexy [31].
11.2.2 Midbrain Pathology
Lesions in the midbrain cause several different symptoms including paralysis of vertical gaze, diplopia (double vision due to oculomotor and trochlear nerve weakness), rubral tremor, loss of conjugate movements in the horizontal gaze, altered consciousness, and akinetic mutism. While these symptoms can occur in solitary, more often they occur as constellations of symptoms that can be grouped into syndromes.
Benedikt syndrome: Involves damage to the RN + oculomotor nucleus. Presents with ipsilateral complete third cranial nerve palsy and contralateral involuntary motor movements such as hemiathetosis, hemichorea, or intention tremor.
Claude’s syndrome: Involves damage to the RN + brachium conjunctivum + oculomotor nucleus. Presents with ipsilateral complete third cranial nerve palsy and contralateral cerebellar signs (asynergia, ataxia, dysmetria).
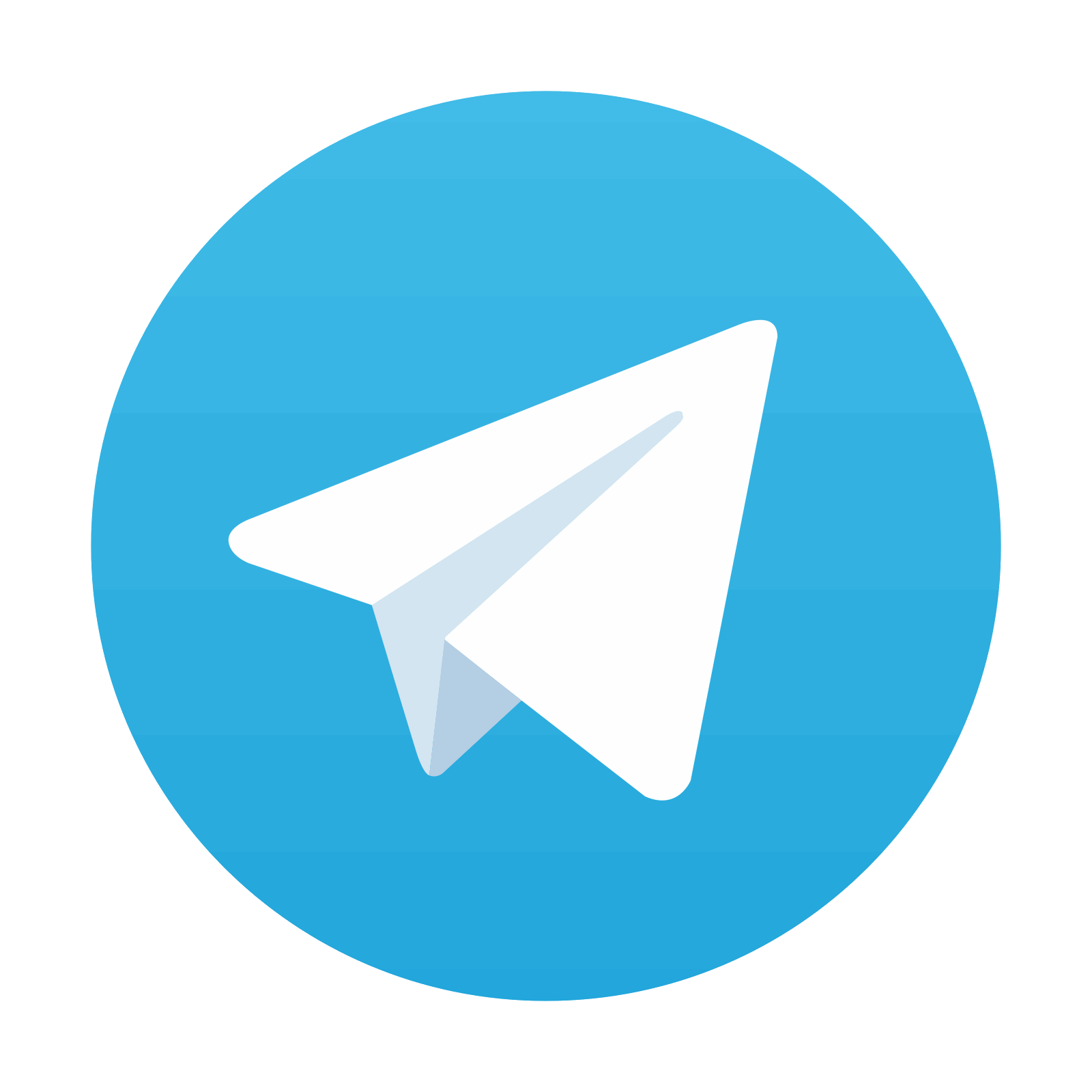
Stay updated, free articles. Join our Telegram channel

Full access? Get Clinical Tree
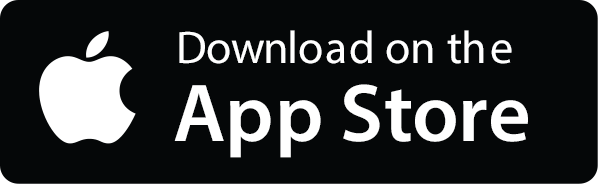
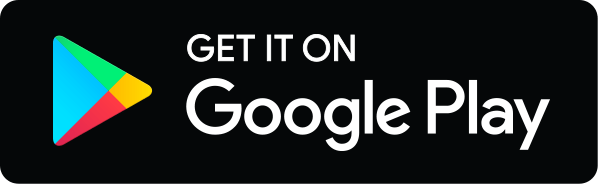