Functional Anatomy of the Olfactory System I: From the Nasal Cavity to the Olfactory Bulb
Structural Considerations
The nasal air passageways are delineated in the anterior plane by the nasal valve, in the lateral plane by the superior, middle, and inferior conchae (turbinates), and in the medial plane by the nasal septum. As seen in Figs. 2.1 and 2.2 , these structures define the organizational borders for the nasal air passages, which provide the conduit through which air and odorant molecules are delivered to the airspace above the olfactory receptors.1
The olfactory receptors are located high in the nose along the septum and on the superior concha. Because of their location, and because the airspaces defined by the middle and inferior conchae are much larger than those leading up to the olfactory receptor region, during a sniff only ~10% of the inspired air is directed toward the airspace in contact with the olfactory receptors.2,3
The septum and conchae produce multiple and convoluted (sometimes turbulent) flow paths for the inspired air ( Fig. 2.3 ). By affecting the rate at which odorant molecules are delivered to the olfactory receptor sheet, the structure of the nose itself may be one of the mechanisms involved with odor detection and discrimination. In addition, some investigators have suggested that the relatively low air volume, coupled with the convoluted air flow path leading to the receptors, may help protect the olfactory processing system from particulate pollutants.4
The nasal air passageways, which can have a dramatic effect on olfactory ability, are delineated by the superior, middle, and inferior turbinates. Alterations in these nasal air passageways often have an effect on odorant perception.
Nasal Air Conditioning, Conduction Problems and the Nasal Cycle
The nose serves important functions beyond odorant identification. These nonolfactory nasal functions together will be referred to as “nasal air conditioning.” During the process of air conditioning, the inspired air is brought to the temperature and humidity of the lungs. In addition, a filtration process occurs in which particles in the inspired air, ranging from combustion products to airborne bacteria, are trapped in the mucus and removed. Some aspects of nasal air conditioning are briefly discussed below because these nonolfactory physiological processes can have a profound effect on the ability to detect odorants.5
The major function of the nose is to provide the lower airways with clean, humidified, and warm air.
The epithelial cells lining the structures of the internal nose have a very rich blood supply and are covered by watery mucus that is continually flowing into the back of the throat. By regulating the amount of blood in the dense capillary beds servicing the structures of the internal nose, the size of the airspace defined by these organizational structures can be changed quickly and dramatically. Because of the speed and the amount of change that can occur around the inferior concha, these areas of the nose are called “swell spaces.”1
If olfactory complaints are due to the problem of odorant molecules not getting to the head space above the olfactory receptors (a conduction problem), breathing warm, humidified air can sometimes temporarily restore some lost olfactory ability by increasing the size of the nasal air passageways. Given the impact the swell spaces can have on nasal patency, questions about how olfactory ability changes as the ambient temperature or humidity change should be included as part of a routine history for patients with olfactory complaints.



Conduction problems can happen as a result of structural blockages or as a result of temporary changes in the nasal air passageways due to conditions such as allergic rhinitis.6 Structural blockages can occur as a result of conditions such as polyposis or as a result of an alteration in the nasal passageways, such as a severely deviated nasal system or facial trauma. With conduction problems, restoring the patency of the nasal passageways often restores olfactory function.1
Inflammatory processes may produce conduction problems. However, inflammatory processes may also produce olfactory loss in the presence of open nasal passages.
When the cause of a complaint is an access problem, olfactory ability can sometimes be restored even after a considerable period of time. For example, when, after many years, patients who had under gone larynectomy were able to move air through their noses by connecting a tube from the stoma to the mouth, olfactory ability was restored to normal levels for most patients.7
As a word of caution, in rats with conduction problems like those accompanying allergic rhinitis, an increase in the size and number of Bowman glands and the infiltration of several kinds of white blood cell have been observed in addition to the blocked nasal passages. If observations from animal models can be applied to humans, restoring nasal airflow may not immediately resolve the olfactory complaint as the increase in gland activity could contribute to olfactory problems.8
The degree of openness of the nasal cavity at some level contributes to the feeling of “comfortable breathing.” In addition, the stimulation of cold receptors and other trigeminal receptors and the presence of a dry mucosa can all contribute to the sensation of stuffiness. Total nasal resistance may also contribute, although there are some data to support the hypothesis that the obstruction on the more open side of the nose is primarily responsible for the presence or absence of nasal complaints.9,10
To add to the complexity of the relationship between nasal anatomy and olfactory ability, a pattern has been observed in most human noses in which congestion on one side of the nose is accompanied by decongestion on the other ( Fig. 2.4 ). This pattern is called the nasal cycle. The periodicity of the cycle is between 50 minutes and 7 hours. There has even been a correlation reported between handedness and the nostril which is open for a larger percent of a 24-hour period, such that left-handed individuals are more likely to have a more open left nostril and vice versa for right handers. The relationship among single-nostril olfactory ability, binasal olfactory ability, and the nasal cycle has not yet been well described.11

Humidification and the Partitioning of Odorant Molecules in the Nasal Mucus
The humidification of incoming air is possible as the respiratory and olfactory mucus layers are mostly composed of water. Thus, when the inspired air is dry, water evaporates from the mucus into the inspired air. The watery mucus can also sorb odorants as they make their way through the nose toward the olfactory receptors. As described in detail below, this sorption is especially noteworthy for water-soluble odorants and it can significantly reduce the number of odorant molecules delivered to the headspace adjacent to the olfactory receptors.12
Using a combination of experimental and modeling techniques, odorant solubility in human nasal and olfactory mucosa has been determined for some odorants. Mucosal odorant solubility is odorant specific and usually follows the trend that odorants with lower water solubility are more soluble in the mucosa than would be predicted from their water solubility alone. Additionally there is a odorant-independent increase in the effective diffusive resistance of the olfactory mucosa compared with that of water alone.13
In addition to supplying water for humidification purposes, the nasal mucus serves as a trap for particulate matter, including smoke and dust particles, and even airborne bacteria. The respiratory and olfactory cells lining the nasal passageways contain cilia, which beat in a slow wavelike action and so move the mucus through the nose to the back of the throat. The flow of mucus toward the back of the throat is one of the mechanisms by which water-soluble odorant molecules sorbed to the mucus bathing the olfactory receptors are cleared. Molecules sorbed in the mucus can also be cleared from the olfactory mucosa through odorant uptake by the circulatory system.1
Ciliopathies, diseases in which the structure and function of cilia are significantly altered, are often accompanied by olfactory problems. This observation underscores the important role cilia play in olfactory transduction.14
Inherent and Imposed Patterns
When odorant molecules are delivered to the headspace above the mucus-coated olfactory receptors, they bind to receptor sites on the cilia of olfactory receptor neurons (ORNs). This produces a change in the membrane potential at the tip of the olfactory receptor cell that, in turn, creates an electronic signal that flows along the axon of the olfactory neuron to the olfactory bulb. The olfactory bulb is composed of several different types of cell, although the axons of the olfactory receptor cells first make contact with glomerular cells.1
Like-tuned receptor cells (there are ~400 different functioning receptor cell types in the human olfactory mucosa) send their signals to specific glomerular cells. With a few exceptions, like-tuned olfactory sensory neurons are segregated to a limited part of the olfactory epithelium (OE) and are thought to be confined to one of the four nonoverlapping zones ( Fig. 2.5 ).15–17
The physiochemical properties of incoming odorants and the distribution of like-tuned olfactory receptors are apparently both important in the peripheral coding of olfactory information.
Most odorants stimulate more than one type of receptor cell and all produce a response pattern across the olfactory mucosa that is unique for that particular odorant. The axons for like-tuned olfactory neurons innervate a limited number of glomeruli which are usually found in a specific locus in the medial glomerular layer and another specific locus in the lateral glomerular layer. As a result, the mucosal activity pattern is maintained, and perhaps even sharpened, in the glomerular cell layer of the olfactory bulb.15–17 There is also recent evidence from OE recordings that is consistent with the hypothesis that there is a zonal expression of ORNs in humans.18
ORNs are expressed in certain zones of the olfactory epithelium.
There is an increasing body of evidence to suggest that the mucosa and bulb patterns play a key role in odorant detection and discrimination.19,20 For example, using voltage-sensitive dyes, Youngentob and his coworkers demonstrated that odorant-induced activity patterns in the mucosa were sharpened as animals learned an odorant identification task ( Figs. 2.5 and 2.6 ).21


In summary, as receptor cells of similar sensitivity are grouped in particular locations along the olfactory receptor sheet, different smells produce different patterns of electrical activity in both the mucosa and the olfactory bulb. These patterns are thought to be one of the ways in which the brain can identify a particular smell. The electrical pattern of activity created by the specific tuning and location of olfactory receptor cells is called the “inherent” mucosal activity pattern. Again, this pattern is maintained at the level of the olfactory bulb.22
In rats, odorant receptor (OR) expression patterns in the olfactory mucosa are restored even after a high exposure to methyl bromide gas. These reconstituted neurons have been shown to have olfactory function. This observation suggests that olfactory problems observed after severe peripheral injuries are not likely to be caused by a lack of regrown olfactory neurons, but rather by a more central problem.22
Because the olfactory receptors are located at some distance from the tip of the nose, incoming odorant molecules interact with the mucous surface of the respiratory epithelium as they are directed toward the airspace adjacent to the superior concha. The nature of this interaction depends on the physical and chemical properties of the odorant molecules themselves. For example, one would expect there to be considerable sorption of mucosa-soluble odorant molecules to the surface of the respiratory mucosa. As a result, fewer of these molecules would reach the olfactory receptor area, and those that did would take longer than molecules of odorants that were not very soluble in the mucosa. In addition, there would be a spatial distribution pattern across the receptor sheet itself, with most of the mucosa-soluble odorant molecules being concentrated early in the flow path compared with a more even mucosal distribution pattern for less mucosa-soluble odorants. The different arrival times and mucosal distribution patterns resulting from an odorant′s solubility may be another of the mechanisms by which the central nervous system identifies smells. These distribution patterns are called “imposed” patterns,23,24 and are likely to be the basis for the differences between nasal and retronasal perception of odors.25,26
There is considerable evidence supporting the existence of imposed patterns in animals. Electrophysiological, gas chromatographic, and radioisotopic studies in frogs and rats have provided strong evidence that the degree of mucosal solubility can affect spatial and temporal distribution patterns along the respiratory and olfactory mucosas.1
Stimulus Parameters
Regardless of the odorant, within physiological limits, there is thought to be a direct relationship between the olfactory response and the number of odorant molecules delivered to the olfactory receptors. That is, more odorant molecules means a more intense smell. However, the olfactory stimulus is actually defined by three “primary” variables: the number of molecules (N); the duration of the sniff (T); and the volume of the sniff (V). These primary variables in turn define the three “derived” variables of concentration: (C = N/V); delivery rate (D = N/T); and flow rate (F = V/T).1,27
From an analysis of variance, Mozell and his coworkers27 generated a model using the three primary variables (NVT) to account for the variability (R) in the olfactory receptor neural activity in bullfrogs. The model was:

The model proposes that the magnitude of the response increases as the sniff volume decreases, whereas the magnitude of the olfactory nerve response increases as the number of molecules and sniff time increase. This model provides a good explanation of the variability in the response of the bullfrog olfactory nerve to subthreshold concentrations of octane.
The relationship among the sniff variables, which influences the interaction of odorants and the olfactory mucosa, is complex, and changing a variable such as flow rate, either experimentally or clinically, also changes other variables that can affect the olfactory response.
Because the primary variables can be represented as derived variables, Mozell was able to test other possible models that included combinations of the primary and derived variables. From this analysis, a model emerged that was even better than the NVT model in explaining the variability in the olfactory nerve response. This model was:

In the FN model, the effects of volume and time are equal and opposite, whereas the number of molecules is independent of the other two. Of all 11 models tested, the FN model was the best at explaining the variability in the olfactory nerve response.1,27
The FN model was generated from bullfrog neural activity. As, in the bullfrog, there is no splitting of the inspired air into different flow channels, all of the inspired air flows over the olfactory sheet. Therefore, if applicable to humans at all, perhaps the FN model best describes the results of the airflow delivered to the olfactory sheet, not what comes into the tip of the nose.
The results from human psychophysical studies looking at the relationship between the olfactory response and changes in the primary and secondary variables have been equivocal. As changing the primary variables also obviously changes the derived variables, determining the appropriateness of any of the above models to human olfactory response has been difficult.
Techniques using Nasal Dilators and Computational Fluid Dynamics
As a research tool, nasal dilators provide a way to temporarily alter nasal anatomy and thus, when combined with computational fluid dynamics, provide a way of beginning to evaluate the FN model.
Nasal dilators are elastic strips with plastic springs running along their long axis. When applied externally to the skin covering the nose, the springs increase the diameter of the nasal cavity in the region of the nasal valve. Psychophysical recordings have shown that nasal dilators increase perceived odorant intensity, reduce odorant detection thresholds, and increase odorant identification. In addition, pneumotachograph recordings have demonstrated that the mean flow rate, maximum flow rate, and volume and duration of the sniff at the tip of the nose are all increased when nasal dilators are worn.28,29
At first glance, there appears to be a contradiction between the results of the FN model and the observation that when wearing nasal dilators the olfactory perception is increased because of higher flows, larger sniff volumes, and longer sniffs. However, the increases in the flow characteristics reported from the nasal dilator studies were recorded at the tip of the nose. Any estimates of the actual flow to the olfactory receptor region and the subsequent odorant deposition patterns in this part of the nose have been a matter of only speculation.
Computational fluid dynamics techniques have been used in the past to predict the impact of regional airway changes on airflow and odorant distribution patterns, especially to the olfactory region.23 Three-dimensional numerical nasal models for numerical simulation of nasal airflow were constructed in dilated and nondilated conditions.
The data from these modeling studies may provide a glimpse into the appropriateness of the FN model in humans. The dilator-induced increase in size of the lower part of the nose directs more of the inspired air along the floor of the nose and therefore reduces the flow to the olfactory area.30 Obviously, there is some limit to the applicability of the FN model in explaining the olfactory response. At its extreme, a really low flow would not be expected to generate any olfactory response. Therefore, the model needs to be considered as describing events within certain physiological limits. The description of these limits awaits further studies.
Because of the location of the olfactory receptors in humans, changes along the flow conduit leading up to the receptors may have surprising effects on olfactory ability. Widening the nasal air passageways or increasing the air flow at the tip of the nose may not necessarily improve olfactory ability.
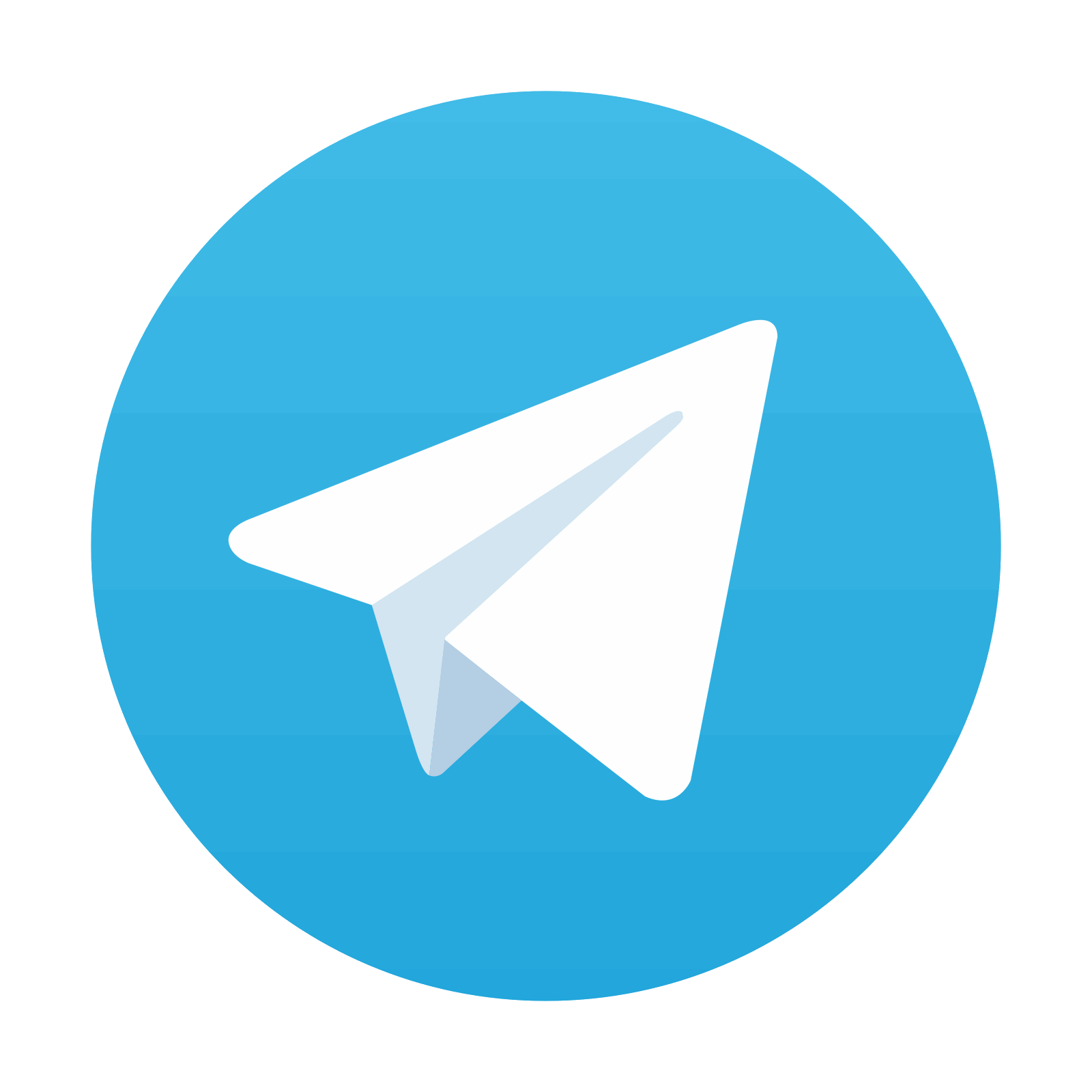
Stay updated, free articles. Join our Telegram channel

Full access? Get Clinical Tree
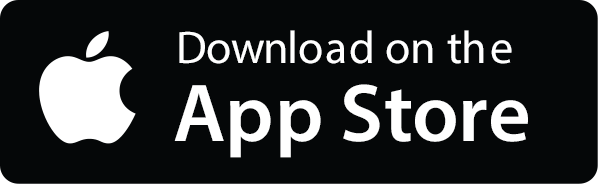
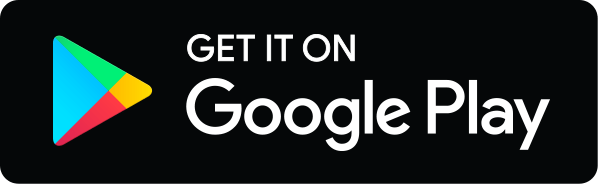
